Biomechanics of the Hip Joint
Under physiological hip joint loading, an inhomogeneous pressure distribution is present within the acetabulum, due to the physiological incongruence of the hip joint.1,2,3,4,5,6,7 Anatomically, there is a difference between the size of the femoral head and the corresponding diameter of the acetabulum, with the femoral head having a slightly enlarged diameter.8
The extent and the direction of the resulting forces determine the localization and amount of pressure distribution within the lunate surface of the acetabulum. Thus, low loading leads to initial anterior and posterior stress at the lunate surface, followed by loading of the acetabular roof only with higher loadings.4,5 Correspondingly, the acetabular roof is not loaded in the swing phase during walking.
This physiological joint incongruence is useful because, at low loadings, cartilage contact with synovial fluid leads to adequate nutritional cell support.5 Full bone contact between the femoral head and the acetabular surface is only present under loading with 50% body weight in adults and potentially already with 25% body weight in elderly people.
The main forces acting on the acetabulum are present in the superior parts, the acetabular dome.7,9,10 This is represented by the thickness of the acetabular and the corresponding femoral head cartilage.10,11,12,13 A further relevant factor is the horseshoe shape of the acetabular cartilage. The free segment of the acetabular fossa leads to an optimization of force distribution within the acetabulum.14
A tensile principle is attributed to the transverse acetabular ligament.6,15 In contrast, injury to this ligament, as can be suspected in some acetabular column fractures, has not been shown to have an effect on the acetabular mechanics.16
In a biomechanical analysis on 17 human cadaver pelvises, Konrath et al found a physiological (normal) load distribution within the acetabulum.16 Transsection of the transverse ligament led to an insignificant change in contact areas, pressure peaks, and pressure distribution at the acetabular cartilage in the anterior and superior joint regions, whereas the combined transsection of the transverse ligament and the acetabular labrum resulted in a reduction of posterior peak pressures.
Injury of these latter structures is therefore not a predisposing factor to the development of a posttraumatic osteoarthritis, although the acetabular labrum enlarges the femoral head coverage by about 30%.17
2.2 Loads Under Physiological Movements
Bergmann et al analyzed the resulting forces acting on the hip joint during certain physiotherapeutic exercises based on a telemetric hip prosthesis.18
The resulting forces acting on the hip joint in the double leg stance position were as high as approximately 70% body weight (BW). During normal walking, peak loads of approximately 300% BW could be observed, whereas using one crutch led to a one-fourth force reduction.19 During walking, depending on walking speed, peak loads increased up to 500% BW, jogging increased these loads to > 700% BW, and the most highest forces were observed while tripping (870% BW).19 Guided movements under physiotherapy lead to maximum loads of 50% BW, whereas active movements resulted in an increase to 250% BW.18
2.3 Biomechanics of Acetabular Fractures
In contrast to physiological load transmission, fractures of the acetabulum result in a substantial change of the biomechanics of the hip joint. Several studies analyzed different fracture situations.
2.3.1 Posterior Wall Fractures
Olson et al analyzed the importance of different defects of the posterior wall in relation to resulting contact areas at the acetabular cartilage.20,21 The contact area between the femoral head and the acetabular surface was found to be located superior in 48% of cases, anterior in 28%, and posterior in 24%. Posterior wall defects of approximately 33% of the joint surface led to an increase of the superior contact area to 64%, whereas a contact reduction was observed anterior and posterior to 21% and 15%, respectively. Further defect sizes led to a further increase of the superior contact area, but to a lesser decrease anteriorly and posteriorly. The total contact area was reduced from 9.21 cm2 in the intact acetabulum to 6.87 cm2 after complete defects of the posterior wall.
2.3.2 Posterior Column Fractures
Vrahas et al analyzed the hip joint stability in different posterior column fractures.22 They distinguished between very high fractures, extending to the superior area of the greater sciatic notch, as intermediate, deep, and very deep fractures. The latter had their most proximal level at the sciatic spine. The more proximal the fracture was, the more likely was the resulting displacement and hip joint instability.
2.3.3 Anterior Wall Fractures
Konrath et al analyzed resulting joint effects of anterior wall defects.23 Fractures of the anterior wall resulted in a significant reduction of the anterior and posterior contact areas and peak pressures. In contrast, no changes were observed in the superior acetabular region.
2.3.4 Anterior Column Fractures
Acetabular instability depends on the fracture course: the more inferior the fracture course, the more stable the fracture. Thus, the most common clinical fracture type, leaving the acetabulum at the anteroinferior horn, is the most stable fracture type in anterior column fractures, whereas anterior column fractures extending to the iliac crest are potentially unstable fractures.22
2.3.5 Transverse Fractures
High extending transverse fractures (transtectal) are the most unstable fractures.22 Clinically, the roof arc measurements are of relevance. If this angle is 30–40 degrees, an unstable fracture can be suspected, whereas a transition zone to the stable fractures is observed in roof arc angles of 40–60 degrees.22 These results were confirmed in another study, where stable fractures were observed in roof arc angles below 60 degrees.24
2.3.6 Both-Column Fractures
Levine et al analyzed the influence of secondary joint congruence in simulated both-column fractures.25 Congruence was held by the supraacetabular and posterior plate.
A significant increase in the resulting forces in the superior area was observed with a corresponding decrease of these forces at the anterior articular surface, whereas the decrease of the posterior resulting forces were not significant.25
2.3.7 Other Fracture Types
Biomechanical data on associated transverse and posterior wall fractures, associated posterior column and wall fractures, T-type fractures, and associated anterior column plus posterior hemitransverse fractures are not available.
Clinical Relevance
The fracture height determines the joint instability. Both anterior and posterior fractures as well as fractures involving both columns lead to stress concentrations in the acetabular roof area.
A recent analysis of bone density differences surrounding the acetabular cartilage in fractures stated a negative correlation to the functional long-term outcome, when the superior region showed an increased bone mineral density.26
Clinical Relevance
An injury in the acetabular roof area seems to be an important negative prognostic factor in acetabular fractures.
2.4 Biomechanical Consequence of Anatomical Joint Reconstructions
Various biomechanical studies reported on the effects of anatomical joint reconstruction for various acetabular fractures.
Olson et al analyzed the effects of the anatomical joint reconstruction by lag screw and plate osteosynthesis after posterior wall fractures.20 Even anatomical joint restoration caused stress concentrations at the acetabular roof area and a reduction of stress concentrations at the anterior and posterior joint surface without achieving values comparable to the intact joint.
Schopfer et al reported similar results after simulation of anatomical fixation of posterior column fractures.27 In contrast, anatomical fixation of anterior column or wall fractures was not associated with superior acetabular stress concentrations.23,28 However, comparable values to the intact acetabulum were not observed.
Clinical Relevance
2.5 Biomechanical Consequence of Articular Gaps and Step-Offs
Persisting steps or gaps after reconstruction of anterior column fractures lead to stress concentrations at the acetabular roof area.28
Malkani et al observed that step-offs up to 1 mm were tolerable, whereas step-offs ≥2 mm resulted in a significant acetabular peak pressure increase.31
Hak et al analyzed that the fracture height in the transverse fractures was of crucial importance for stress concentrations. A persisting step after transtectal fracture was associated with highest pressure maxima, whereas remaining steps and gaps after juxtatectal fractures were without significance.29
Clinical Relevance
Remaining joint step-offs were associated with more relevant increase in the peak forces at the acetabular roof than gaps, potentially increasing the risk of development of posttraumatic osteoarthritis.
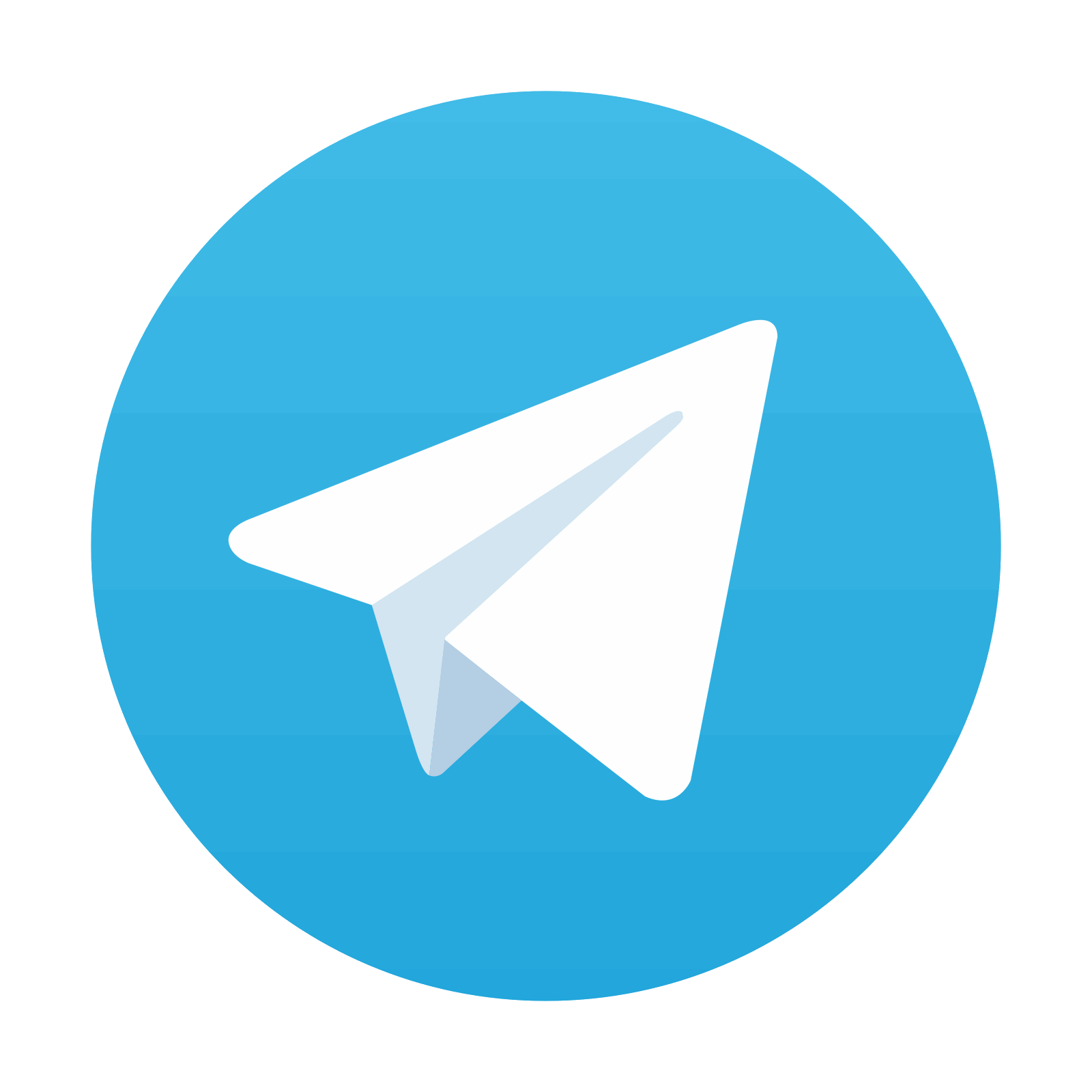
Stay updated, free articles. Join our Telegram channel

Full access? Get Clinical Tree
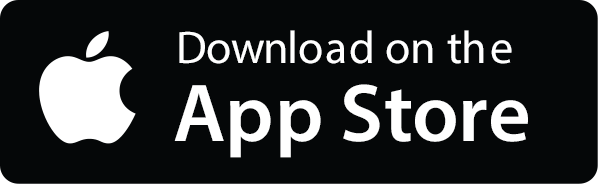
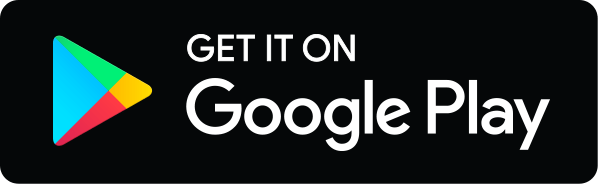