Biomechanical Outcomes of Cartilage Repair of the Knee
Articular cartilage defects of the knee have been documented in over 60% of all patients undergoing knee arthroscopy.1 In the athletic population, the prevalence of these lesions may be even greater than in the general population.2 Patients with focal cartilage defects may have major problems with pain and loss of function, equivalent to those scheduled to undergo total knee arthroplasty.3 Cartilage repair or restoration is a reliable option for symptomatic patients who have failed non-operative treatment. Clinical outcomes measures are important to aid in the development and optimization of articular cartilage treatment strategies. As techniques in cartilage surgery continue to improve, it is vital that high-level evidence be employed to evaluate patient-reported surgical and functional outcomes. Despite the high levels of interest in cartilage repair and restoration techniques, there are few studies that have evaluated the effects of cartilage surgery on biomechanical and neuromuscular function of the joint and the lower extremity. The focus of the following review is to evaluate the current evidence available on biomechanical functional outcomes after cartilage repair with clinical applications to the rehabilitation process.
As one of the primary load-bearing surfaces of the body, the knee joint surface endures high loads not only during high-impact sports activities but also during activities of daily living. The knee joint may be subjected to loads two to eight times body weight during simple squatting tasks and gait.4–7 The articular surface of the knee is able to withstand these high loads due to its highly durable, biologically active tissue, with nearly frictionless and elastic material properties that withstand large compressive loads without permanent deformation. The biphasic nature of articular cartilage, composed primarily of water and extracellular matrix, provides unique properties necessary to sustain a healthy knee joint, despite the heavy load demands sustained by the tibiofemoral and patellofemoral surfaces. However, the hypocellular, relatively avascular environment of articular cartilage hinders the healing potential of damaged articular cartilage. In the setting of a neural structure, patients may have large cartilage defects with minimal symptoms and subconscious gait alterations that may help unload damaged areas. Given the poor healing potential of articular cartilage lesions and the subsequent disability, there is a high interest in cartilage repair and tissue-engineering techniques.
The knee joint is subjected to a variety of external loads (ground reaction forces and perturbations) and internal loads (muscle forces, joint reaction forces as a result of the anatomy) during functional activities such as gait, squatting, and activities of daily living. Muscles that span the knee joint as well as muscles that do not cross the joint can lead to high knee joint loads. Small alterations in walking kinematics, in particular knee flexion angles, may significantly affect the magnitude of knee joint forces.8–10 Alterations in chondral surfaces and gross cartilage defects alter the load-bearing capabilities of the surrounding tissue.11,12 The rims of chondral defects bear significantly higher loads than healthy cartilage areas and may lead to defect progression.12–17
Knee joint loading profiles in both the tibiofemoral and patellofemoral compartments are influenced by the thickness of the articular cartilage, the joint′s radii of curvature, the shape of the menisci, mechanical axis, patellofemoral alignment, and ligamentous stability. In the setting of cartilage repair, achievement of joint surface congruity and defect filling likely decreases the rim stresses of articular cartilage at focal defect areas ( Fig. 17.1 ). Theoretically a decrease in rim stresses may prevent or minimize defect progression and may even lead to full integration of cartilage to surrounding normal articular cartilage and underlying subchondral bone. Cartilage repair techniques such as abrasion arthroplasty, drilling, and microfracture penetrate the subchondral bone to induce fibrocartilage formation and demonstrate positive functional and objective outcomes in the short term. However, longer follow-up studies indicate that functional and objective outcomes after these marrow stimulation techniques decline with time, particularly for larger defects.18–26 In contrast, cartilage restoration techniques (osteochondral auto-graft, osteochondral allograft, mosaicplasty, and autologous chondrocyte implantation [ACI]) that incorporate more hyaline-like cartilage in focal defects may offer more long-term successful outcomes.27
Knee Joint Biomechanics
Two separate layers of calcified and uncalcified cartilage contribute to the biomechanical properties of articular cartilage in the knee. The uncalcified region (includes superficial, transitional, and deep zones) possesses a unique structural composition and provides both tensile strength and resilience to compression and deformation. The calcified layer is deep to the tidemark, overlies the highly vascularized subchondral bone region, and provides an attachment to the underlying subchondral bone.
Tibiofemoral Joint
Knee joint motions between the femoral condyles and the tibial plateau can occur in all three planes (sagittal, frontal, and trans-verse) with six degrees of freedom that allow 12 directional motions ( Fig. 17.2 ).28,29 The tibiofemoral joint can rotate in the sagittal plane by flexion and extension, in the frontal plane by abduction and adduction, and in the transverse plane by internal and external rotation. Translations of the tibiofemoral joint occur in the sagittal plane anteriorly and posteriorly, in the frontal plane medi-ally and laterally, and in the transverse plane by compression and distraction ( Fig. 17.2 ). While the knee can move in all 12 of these potential directions, with the exception of sagittal plane rotations (flexion/extension), most of these motions occur through a relatively limited range. In addition, motions in the transverse and frontal planes (as well as sagittal plane translations) are influenced by the amount of knee flexion and the anatomy of the femur, tibia, and surrounding ligamentous structures.


Movements between the articulating surfaces of the tibia and femur play key roles in knee joint function. The menisci also contact both the tibial and the femoral surfaces. The menisci decrease the stress transmission to the articular cartilage by increasing the surface contact area.30 The contact points of the tibia and femur in the sagittal plane move posteriorly during knee flexion via a combination of both rolling and gliding motions. The fem-oral condyles glide anteriorly as the femur rolls posteriorly during flexion, reducing the posterior progression of the rolling effect and preventing the subluxation of the femur off the back of the tibia.30,31 There is a significant asymmetry in the anatomy and kinematics of the medial and lateral tibiofemoral compartments.32,33 The medial compartment, with the medial tibia possessing a firmly attached medial meniscus on two angled flat surfaces of the medial tibia and articulating with the convex medial femoral condyle, experiences minimal gliding in the anterior-posterior directions and minimal rotations in the trans-verse plane in unconstrained, nonweight-bearing conditions.34 In contrast, the lateral compartment is composed of a convex-on-convex articulation, due to the shape of the lateral tibial plateau.35,36 Increased anterior-posterior glide, as well as transverse rotations during unconstrained, non–weightbearing conditions occur in the lateral compartment due to the less adherent lateral meniscus and less bony constraint imposed by the lateral plateau relative to the medial plateau.37 Thus, the center of rotation of the knee joint during non–weightbearing conditions is likely in the medial compartment.32,37,38 In contrast to non–weightbearing conditions, knee kinematics are different during gait. Koo and Andriacchi demonstrated that, during gait, the center of rotation of the knee joint is primarily on the lateral side with the medial side demonstrating greater anterior-posterior translation.39 The convex–concave surface contact area of the medial compartment is much greater than the convex–convex surface contact area of the lateral side during weight bearing. The greater potential surface area that may change with an alteration in gait (i.e., that may occur with pathology such as osteoarthritis) may make the medial compartment more sensitive to small changes in knee joint kinematics.35
Patellofemoral Joint
The bony anatomy of the patella and the trochlea of the femur provide inherent stability to the patellofemoral joint, with the medial and lateral facets of the posterior surface of the patella articulating and tracking within the trochlear groove. The superior 75% of the posterior surface of the patella is made up of articular cartilage, and, with thicknesses up to 5 to 6 mm, it has the thickest articular cartilage surface in the human body.34 Since contact pressures of up to 12 MPa may occur during maximal extensor mechanism contractions, the thick articular surface and smooth congruent surface contact between the trochlea and patella are necessary for optimal performance of the extensor mechanism.40–42
The patella undergoes both a rolling and a gliding motion along the articulating surface of the femur as it increases the mechanical advantage of the extensor muscles. The quadriceps muscles (rectus femoris, vastus lateralis, medialis, and intermedius), which are important for knee extension movements, originate from the pelvis (ilium) and proximal femur. The quadriceps muscles wrap around the patella and unite to form the patellar tendon that attaches the patella to the tibial tubercle. The patella serves as a fulcrum for quadriceps muscle moment generation since it displaces the quadriceps tendon anteriorly and increases the internal moment arm of the knee extensor mechanism (the larger the internal moment arm, the greater the internal moment produced per level of force generation).43 The knee flexion angle influences the patellar–trochlear surface area contact and pressure. The patella engages with the trochlea between 20 and 30 degrees of knee flexion. Near full extension of the knee, the inferior surface of the patella articulates with the superior portion of the trochlea in a single-contact, horizontal fashion. As knee flexion increases, more proximal portions of the patella contact the trochlea and the horizontal contact transitions into separate areas of contact on the lateral and medial facets at ∼ 130 degrees of knee flexion.42 Patellofemoral surface contact increases ∼ 24% during flexion with weight-bearing activities compared with non–weightbearing conditions.44 The highest contact pressures in the patellofemoral joint occur between 60 and 90 degrees of knee flexion.40
Biomechanics of Cartilage Rehabilitation
The rehabilitation program after cartilage repair is critical to optimize surgical outcomes. Physiologic healing and chondrocyte protection are paramount to the success of the surgical procedure. However, this healing and protection must be balanced with optimization of the rehabilitation program. The goals of the rehabilitation process should be to minimize disability, immobilization, and overall morbidity accounting for the complications that may occur with small arthroscopic procedures and/or large open procedures combined with osteotomies.
Due to the high variability in lesion location, nature of the defect, and surgical procedures performed, personalized rehabilitation strategies are necessary. Awareness of the location of the defect, size of the defect, concomitant procedures, type of healing response anticipated for the surgical procedure performed, and goals of the patient for return to both activities of daily living and high-level activities such as sports are important considerations during the rehabilitation stage.45 Currently, there is minimal evidence in the literature regarding rehabilitation programs following cartilage repair. Most of the available literature about rehabilitation is based on biomechanical theories and expert or nonexpert opinion.
Although protection of the knee joint after a surgical intervention is important, joint immobilization has known detrimental effects on muscle and articular cartilage.46 Even short-term immobilization can induce articular cartilage atrophy, and, although reversible, full restoration of cartilage integrity may not occur.47–49 Animal studies demonstrate that cartilage repair techniques supplemented with early mobilization show superior tissue regeneration and integration.50–52 Successful cartilage repair is predicated on chondrocyte viability, integration of the new repair tissue with the surrounding healthy tissue, and the ability of the repair to withstand high mechanical stresses. In the early postoperative period after cartilage repair, continuous passive motion (CPM) is often used within the first few hours after surgery to counter the immobilization effects, to enhance metabolic and nutritional activity of the cartilage, and to prevent joint stiffness.52,53 However, CPM recommendations are largely based on basic science and animal model studies with few in vivo clinical studies available to support this accepted clinical practice.54 A recent systematic review revealed only four human in vivo studies that evaluated the use of CPM after cartilage repair.53,55–58 No randomized controlled studies were available to support the use of CPM, and all studies varied significantly in the recommendation of time and duration of use (from 6 to 8 hours per day for 6 days up to 8 weeks of use).53,55–58 Rodrigo et al showed improvement of the defects on second-look arthroscopy in patients who used CPM compared with the control group; however, no correlation to functional outcome was reported.58 Although available only on a small group of subjects, histological outcomes on second-look arthroscopy do not show significant differences between patients who use CPM and those who perform only active range of motion.57,58 In general, rehabilitation programs encourage early, interval increases in joint motion starting with passive range-of-motion activities. Active joint range of motion may have higher joint-contact pressures than passive range of motion; thus, most programs begin with passive range of motion with slow progression into active range-of-motion and strengthening activities.59
Weight-bearing guidelines after cartilage repair vary widely in the literature.59 In general, most recommendations use separate guidelines for femoral/tibial defects compared with patellofemoral defects. The mechanics of weight bearing and walking may increase the risk of articular cartilage breakdown when the normal mechanical environment and balance between loading and biological maintenance of cartilage are compromised.60,61 During the early postoperative weeks following cartilage repair, the cells contained in the defect start differentiating and develop into a soft repair tissue that is highly vulnerable to, but also may be stimulated by, mechanical loads.62 It may take up to 2 years for the repaired lesion to develop into the optimal cartilage phenotype.63,64 Small mechanical loads may promote cartilage metabolic activity and nutrition, similar to CPM effects. Thus, the challenge in the early postoperative period is to provide small bouts of loading stimulus to the healing articular lesion, without subjecting the cartilage repair site to significant, potentially damaging mechanical overload.65 The restriction of weight bearing in the early postoperative phase, particularly in the tibiofemoral joint, may theoretically protect the cartilage repair and decrease the mechanical stress that the repair site is subjected to during the most vulnerable time period.
Often patients with cartilage repair in the tibiofemoral compartment are restricted to non–weightbearing in the first few weeks after restoration, with slow progression over 2 to 12 weeks to full weight-bearing conditions.45 Weight-bearing movements result in increased pressure on the tibiofemoral articulation throughout the entire arc of knee motion, and contact forces can reach four times body weight at 90 degrees of knee flexion.66,67 In addition to restriction of the amount of weight exerted on the limb after cartilage repair, clinicians should consider other external loads during gait that may subject the knee to mechanical stresses. Ebert et al found that in ACI patients the contribution of ground reaction force at different percentages of weight-bearing status demonstrates a large variability in kinematic, spatiotemporal parameters and external knee moments.68 Thus, in addition to the ground reaction force, other variables may affect the external loads experienced by the knee joint during gait. Also, from a patient perspective, despite strict instruction and practice of partial weight-bearing restrictions on bathroom-type weight scales it is difficult to accurately replicate “partial weight-bearing” restrictions.69 To prevent mechanical shear and high-contact pressures during the most vulnerable healing time after cartilage repair, weight-bearing restrictions have often been strict, with slow progressions. However, there is limited good evidence regarding how weight-bearing restrictions affect functional outcomes.
An assessment of ACI biopsies taken on 11 patients (12 graft sites on femoral condyles) at second-look arthroscopy at 3 to 7 years after the initial procedure demonstrated stiffness measures of 90% or more of the value of controls in 8 of the 12 biopsies and good to excellent grading of integration of graft to the surrounding cartilage in 10 of the 11 subjects. All patients followed a weight-bearing protocol that started with gradual weight-bearing for 8 weeks with progression to full weight-bearing at 10 weeks.70 A randomized controlled trial that evaluated clinical outcomes in “accelerated” versus “delayed” weight bearing for matrix- associated ACI of the femoral condyle demonstrated no differences in clinical outcome 2 years postoperatively. The accelerated group was allowed 20% weight bearing for the first 2 weeks, 50% weight bearing between 2 and 4 weeks, and progression to full weight bearing after 6 weeks. The delayed group was allowed toe-touch weight bearing only until 4 weeks postoperatively, followed by 20% weight bearing at 4 to 6 weeks, 50% weight bearing at 6 to 8 weeks, with gradual increase to full weight bearing at postoperative week 10.71 Ebert et al compared an accelerated rehabilitation that allowed full weight bearing at 8 weeks compared with delayed full weight bearing until 11 weeks postoperatively after matrix-induced autologous chondrocyte implantation (MACI) in the femoral condyle resulted in the accelerated group demonstrating improved function, reduced knee pain, and no significant changes on MRI at 3 months postoperatively compared with the delayed group.72 Thus, early pilot studies demonstrate that it may be possible to accelerate weight-bearing loads in femoral articular cartilage repair without compromising the repair.
In contrast to tibiofemoral cartilage repair rehabilitation, most patellofemoral cartilage repair protocols allow for immediate weight bearing with the knee in full extension or with restricted range of motion to 0 to 30 degrees of knee flexion. This is theoretically a “safe” range of motion for patellofem-oral patients because the patella does not engage with the trochlea until 20 degrees of knee flexion and the patellofemoral joint reaction force is 50% of the quadriceps force in full knee extension.40,73 In contrast to tibiofemoral cartilage repair, patients with patellofemoral pain or patellofemoral cartilage restoration likely benefit from weight-bearing restrictions and exercises that minimize the patellofemoral joint reaction forces but maximize quadriceps forces. Van Eijden et al demonstrated that between 70 and 100 degrees of knee flexion the patellofemoral joint reaction force is equal to the quadriceps force.73 In contrast, from full knee extension to 40 degrees of knee flexion the patellofemoral joint reaction force is 50 to 90%, respectively.73 During squatting activities, the highest patellofemoral joint forces and stresses occur at 90 degrees of knee flexion. Increased external loads (increased weight) significantly increase the patellofemoral joint stresses.74 Targeted rehabilitation exercises that avoid larger knee flexion angles may allow for quadriceps strengthening exercises that reduce patellofemoral joint stresses. However, timing of implementation of quadriceps activation and maximal muscle force recruitment may need to be modified and taken into consideration for cartilage procedures that are combined with tibial tubercle osteotomies.
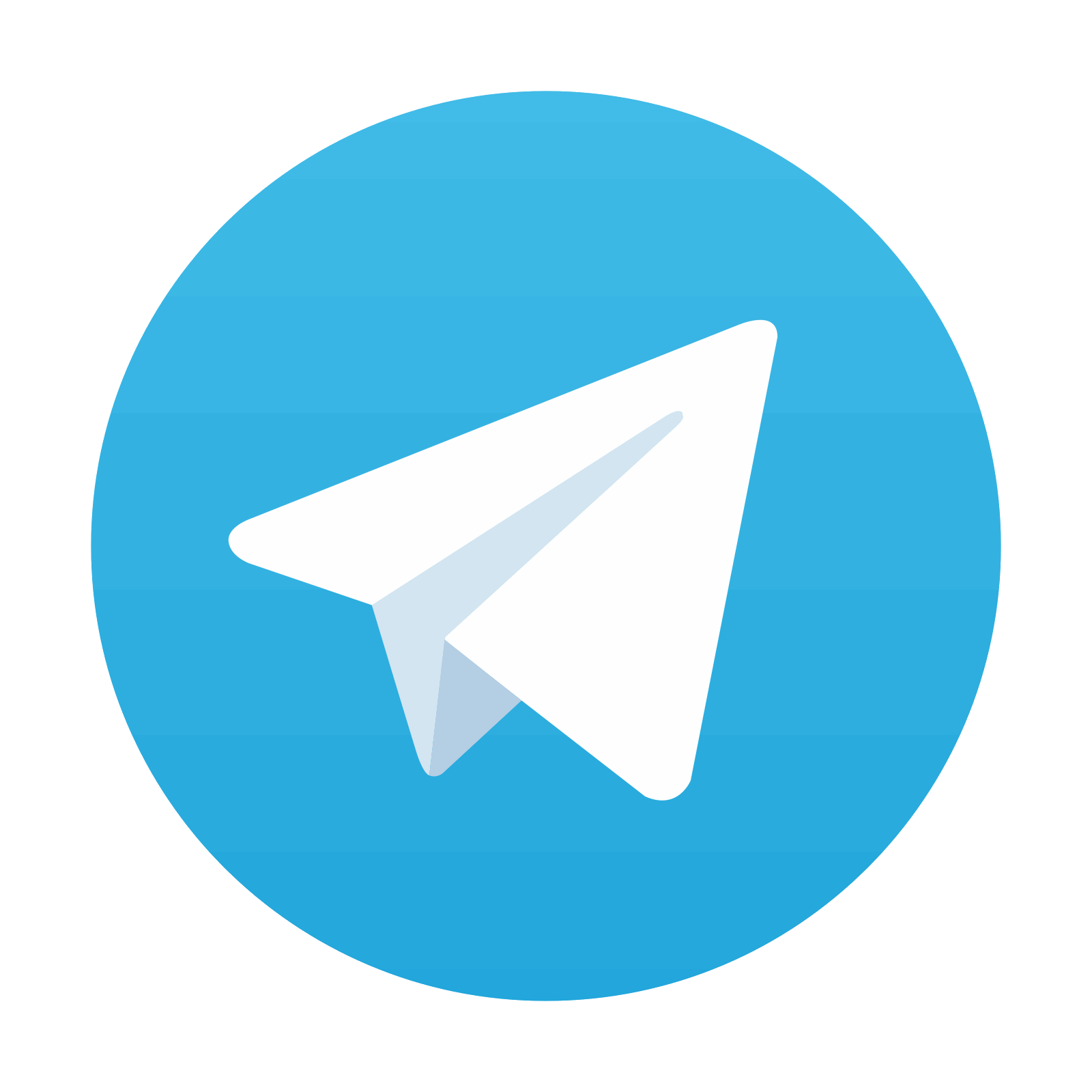
Stay updated, free articles. Join our Telegram channel

Full access? Get Clinical Tree
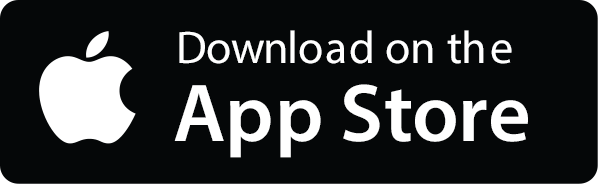
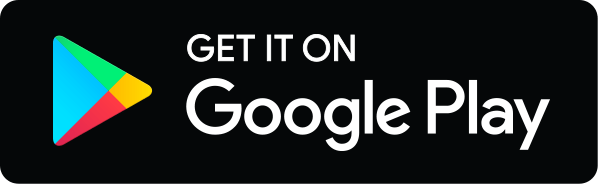