Fig. 13.1
This figure describes the neuroanatomical locations of the blood-based biomarkers that will be reviewed
Need for Serum Biomarkers for Concussion
Diagnostic and prognostic tools for risk stratification of concussion patients are limited in the early stages of injury in the acute setting. Unlike other organ-based diseases where rapid diagnosis employing biomarkers from blood tests is clinically essential to guide diagnosis and treatment, such as for myocardial ischemia or kidney and liver dysfunction, there are no rapid, definitive diagnostic tests for TBI. Research in the field of TBI biomarkers has increased exponentially over the last 20 years [16, 17], with most of the publications on the topic of TBI biomarkers occurring in the last 10 years [18]. Accordingly, studies assessing biomarkers in TBI have looked at a number of potential markers that could lend diagnostic and, prognostic, as well as therapeutic information. Despite the large number of published studies, there is still a lack of any FDA-approved biomarkers for clinical use in adults and children [17, 18]. Properties that should be considered when evaluating a biomarker for clinical application include the following: does the biomarker: (1) demonstrate a high sensitivity and specificity for brain injury; (2) stratify patients by severity of injury; (3) have a rapid appearance in accessible biological fluid; (4) provide information about injury mechanisms; (5) have well defined biokinetic properties; (6) monitor progress of disease and response to treatment; and (7) predict functional outcome [17, 19].
Biofluid Biomarkers of Astroglial Injury
S100β[Beta]
S100β[beta] is the major low affinity calcium binding protein in astrocytes [20] that helps to regulate intracellular levels of calcium and is considered a marker of astrocyte injury or death. It can also be found in non-neural cells such as adipocytes, chondrocytes, and melanoma cells [21, 22]. S100β[beta] is one of the most extensively studied biomarkers [23–33] and elevation of S100β[beta] levels in serum has been associated with increased incidence of post-concussive syndrome and impaired cognition [34, 35]. Other studies have reported that serum levels of S-100β[beta] are associated with MRI abnormalities and with neuropsychological examination disturbances after mild TBI [36, 37]. A number of studies have found significant correlations between elevated serum levels of S100β[beta] and CT abnormalities [38–40]. It has been suggested that adding the measurement of S100β[beta] concentration to clinical decision tools for mild TBI patients could potentially reduce the number of CT scans by 30 % [40]. Other investigators have failed to detect associations between S100β[beta] and CT abnormalities [41–44].
Amateur boxers have slightly elevated levels of S100β[beta] in CSF samples obtained by lumbar puncture after a bout [45]. In a study of S100β[beta] in basketball and hockey players by Stalnacke et al. in 2003, there was a significant correlation between the change in S100β[beta] (postgame−pregame values) and jumps in basketball players (r = 0.706, p = 0.002). In one ice hockey player who experienced concussion during play, S100β[beta] was increased more than for the other players [46]. The same investigators conducted a study of soccer players and found that changes in S100β[beta] concentrations (postgame minus pregame values) were statistically correlated to the number of headers (r = 0.428, p = 0.02) and to the number of other trauma events (r = 0.453, p = 0.02) [47]. Although S100β[beta] remains promising as an adjunctive marker, its utility in the setting of multiple trauma remains controversial because it also increases in trauma patients without head injuries and therefore be considered nonspecific to TBI [48–51].
Glial Fibrillary Acid Protein
Glial fibrillary acidic protein (GFAP) is a monomeric intermediate protein found in astroglial skeleton that was first isolated by Eng et al. in 1971 [52]. GFAP is found in white and gray brain matter and is strongly upregulated during astrogliosis [53]. Current evidence indicates that serum GFAP might be a useful marker for various types of brain damage from neurodegenerative disorders [54, 55] and stroke [56], to severe TBI [51, 57–61]. In 2010, Vos et al. described serum-increased GFAP profile in severe and moderate TBI with GCS <12 and found an association with unfavorable outcome at 6 months [32]. More recently, Metting et al. found GFAP to be elevated in patients with axonal injury on MRI in patients with mild TBI at 3 months post-injury, but it was not predictive of global outcome at 6 months [62]. In a study by Papa et al. in 2012, GFAP was detectable in serum less than 1 h after a concussion and was able to distinguish concussion patients from other trauma patients (without head injury) who had orthopedic injuries or who were in motor vehicle crashes [63]. In this same study, serum GFAP was significantly higher in mild TBI patients with intracranial lesions on CT compared to those without lesions and predicted patients who required neurosurgical intervention [63]. Similarly, Metting et al. demonstrated that serum GFAP was increased in patients with an abnormal CT after mild TBI. These studies suggest that GFAP has a good specificity for brain injury acutely after injury.
In amateur boxers, GFAP has also been found to be elevated in CSF samples obtained by lumbar puncture after a bout [45, 64]. Neselius et al. examined the CSF of 30 Olympic boxers and 25 non-boxing matched controls at 1–6 days after a bout and after a rest period (>14 days). Both GFAP and S100β[beta] concentrations were significantly increased after boxing as compared to controls. However, GFAP concentrations remained elevated after the rest period but S100β[beta] did not. It was suggested that the presence of GFAP after the rest period indicated ongoing degeneration.
Biofluid Biomarkers of Neuronal Injury
Neuron-Specific Enolase
Neuron-specific enolase (NSE) is one of the five isozymes of the glycolytic enzyme enolase found in central and peripheral neuronal cell bodies and it has been shown to be elevated following cell injury [65]. NSE is also present in erythrocytes and endocrine cells and has a biological half-life of 48 h [66]. This protein is passively released into the extracellular space only under pathological conditions during cell destruction. Several reports on serum NSE measurements of mild TBI have been published [65, 67–70]. Many of these studies either utilized inadequate control groups or concluded that serum NSE had limited utility as a marker of neuronal damage. Early increased levels of NSE and MBP concentrations have been correlated with outcome in children, particularly those under 4 years of age [71–74]. A limitation of NSE is the occurrence of false-positive results in the setting of hemolysis [75, 76].
Stalnacke et al. obtained blood samples from 44 female soccer players before and after a competitive game and found that both S100β and NSE were increased after the game. NSE was not related to the number of headers and other trauma events but S100β was [77].
Ubiquitin C-Terminal Hydrolase (UCH-L1)
A promising candidate biomarker for TBI currently under investigation is ubiquitin C-terminal hydrolase-L1 (UCH-L1). UCH-L1 was previously used as a histological marker for neurons due to its high abundance and specific expression in neurons [78]. This protein is involved in the addition and removal of ubiquitin from proteins that are destined for metabolism [79]. It has an important role in the removal of excessive, oxidized, or misfolded proteins during both normal and pathological conditions in neurons [80]. Clinical studies in humans with severe TBI have confirmed, using ELISA analysis, that the UCH-L1 protein is significantly elevated in human CSF [81, 82], is detectable very early after injury, and remains significantly elevated for at least 1 week post-injury [82]. Further studies in severe TBI patients have revealed a very good correlation between CSF and serum levels [83]. Increases in serum UCH-L1 have also been found in children with moderate and severe TBI [84]. Most recently, UCH-L1 was detected in the serum of mild and moderate traumatic brain injury (MMTBI) patients within an hour of injury [85]. Serum levels of UCH-L1 discriminated concussion patients from uninjured and non-head-injured trauma control patients who had orthopedic injuries or motor vehicle trauma without head injury. Most notable was that levels were significantly higher in those with intracranial lesions on CT than those without lesions, as well as those eventually requiring neurosurgical intervention [85].
Biofluid Biomarkers of Axonal Injury
Alpha-II-Spectrin Breakdown Products
Alpha-II-spectrin (280 kDa) is the major structural component of the cortical membrane cytoskeleton and is particularly abundant in axons and presynaptic terminals [86, 87]. It is also a major substrate for both calpain and caspase-3 cysteine proteases [88, 89]. A hallmark feature of apoptosis and necrosis is an early cleavage of several cellular proteins by activated caspases and calpains. A signature of caspase-3 and calpain-2 activation is cleavage of several common proteins such as cytoskeletal α[alpha]II-spectrin [90, 91]. Levels of spectrin breakdown products (SBDPs) have been reported in CSF from adults with severe TBI and they have shown a significant relationship with severity of injury and clinical outcome [92–98]. The time course of calpain-mediated SBDP150 and SBDP145 (markers of necrosis) differs from that of caspase-3-mediated SBDP120 (marker of apoptosis). Average SBDP values measured in CSF early after injury have been shown to correlate with severity of injury, CT scan findings, and outcome at 6 months post-injury [99].
Serum SBDP145 has also been measured in serum in children with TBI. Levels were significantly greater in subjects with moderate and severe TBI than in controls and were correlated with dichotomized GOS at 6 months. This correlation did not hold true for mild TBI. More recently, however, serum levels of SBDP150 have been examined in patients with mild TBI and have shown significant association with acute measures of injury severity, such as GCS score, intracranial injuries on CT, and neurosurgical intervention [100]. In this study, serum SBDP150 levels were much higher in patients with mild TBI/concussion than in other trauma patients who did not have a head injury [100].
TAU Protein
Following a concussion, axons appear to be most susceptible to damage. Two promising biofluid biomarkers localized in the axons are tau protein and neurofilament protein. A supposedly cleaved form of tau, c-tau, has been investigated as a potential biomarker of CNS injury. Tau is preferentially localized in the axon and tau lesions are apparently related to axonal disruption [101, 102]. CSF levels of c-tau were significantly elevated in TBI patients as compared to control patients and these levels correlated with clinical outcome [103, 104]. Though levels of c-tau were also elevated in plasma from patients with severe TBI, there was no correlation between plasma levels and clinical outcome [105]. Total tau protein is highly expressed in thin, nonmyelinated axons of cortical interneurons [106], thus may be indicative of axonal damage in grey matter neurons. It has been found to be correlated with severity of injury in severe TBI [107–110]. Ost et al. found that total tau measured in CSF on days 2–3 post-injury discriminated between TBI and controls (normal pressure hydrocephalus) and also between good and bad outcome at 1 year per dichotomized GOS score [109]. However, total tau was not detected in serum throughout the study. In a study by Zetterberg et al. in amateur boxers, levels of total tau in CSF from lumbar puncture within 10 days of a bout were elevated in both boxers who had received many hits (>15) or high-impact hits to the head, as well as in boxers who reported few hits.
Neurofilaments
Neurofilaments are heteropolymeric components of the neuron cytoskeleton that consist of a 68 kDa light neurofilament subunit (NF-L) backbone with either 160 kDa medium (NF-M) or 200 kDa heavy subunit (NF-H) side-arms [111]. Following TBI, calcium influx into the cell contributes to a cascade of events that activates calcineurin, a calcium-dependent phosphatase that dephosphorylates neurofilament side-arms, presumably contributing to axonal injury [112]. Phosphorylated NF-H has been found to be elevated in the CSF of adult patients with severe TBI as compared to controls [81]. Similarly, hyperphosphorylated NF-H has also been correlated with severity of brain injury in children [113]. In a study by Zurek et al. NF-H levels taken on the second to fourth days remained significantly higher in patients with poor outcomes in comparison to patients with good outcomes. Additionally, NF-H was significantly higher in those children with diffuse axonal injury on initial CT scan [113].
Conclusion
In an effort to prevent CTE and long-term consequences of concussion/mild TBI, early diagnostic and prognostic tools are becoming increasingly important, particularly in athletes and in military personnel, where concussions/mild TBI are common occurrences. The studies conducted on biofluid biomarkers for mild TBI to date show great promise. Should serum biomarkers for TBI be validated and become widely available, they could have many roles. They could help with clinical decision making by clarifying injury severity and help monitor progression of injury and/or recovery. Biomarkers could have a role in managing patients at high risk of repeated injury and could be incorporated into guidelines for return to duty, work, or sports activities.
As a final thought, we must continue the exploration and validation of biomarkers for TBI, especially mild TBI. Ideally, biomarkers would provide information on the pathophysiology of injury, improve stratification, assist in the monitoring of injury progression, monitor response to treatment, and predict functional outcome. Despite the heterogeneity of TBI, there is a unique opportunity to use the insight offered by biochemical markers to shed light on the complexities of this injury process. The development of a clinical tool to help healthcare providers manage TBI patients more effectively and improve patient care is the ultimate goal.
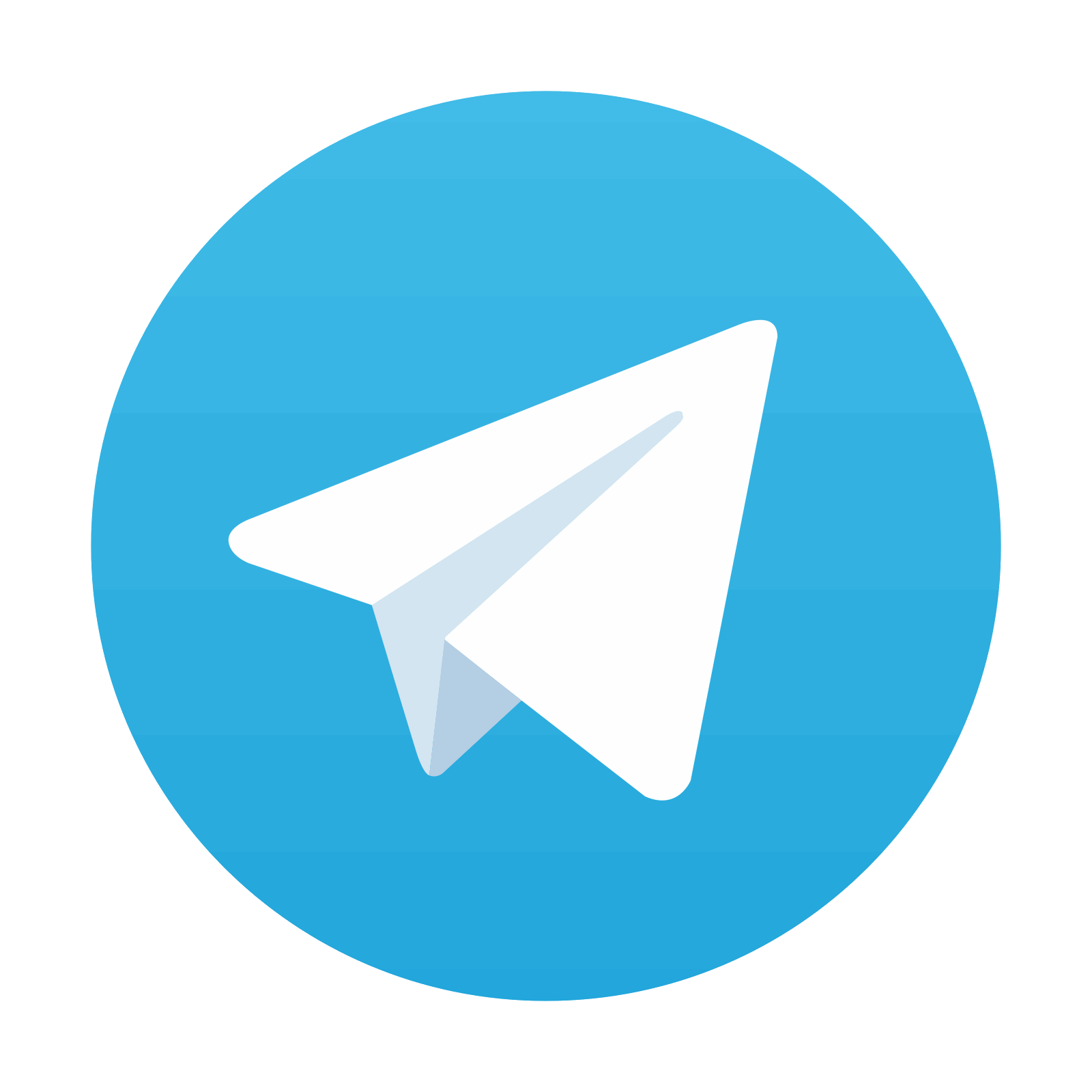
Stay updated, free articles. Join our Telegram channel

Full access? Get Clinical Tree
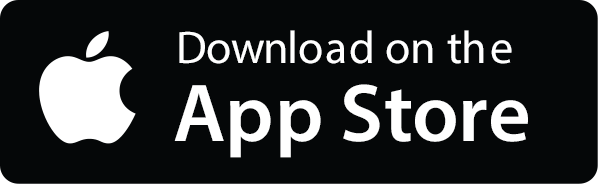
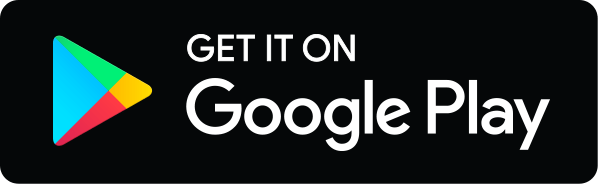