Abstract
The use of biologics in sports medicine has increased over the years, as the available research shows their potential to increase healing rates and improve outcomes. This chapter presents an overview of the historical origins of biologic therapies, currently available biologics in sports medicine, and future directions for research and treatment. Biologic therapies can be used to augment other procedures or as stand-alone treatments; they can be largely divided into growth factor treatments, cell therapies, or tissue transplantations. The most common growth factor treatment used in sports medicine is platelet-rich plasma (PRP). Common cell therapies include concentrated bone marrow aspirate (cBMA), stem cells, and autologous chondrocyte implantation (ACI). Tissue transplantation can include autograft, allograft, and xenograft products. Tissue transplantation can also be characterized by preparation method (fresh, frozen, preserved) and composition (whole tissue, matrix-only product). Use of biologic therapies is tightly regulated by the U.S. Food and Drug Administration (FDA) in the interest of patient protection but does affect the speed at which emerging technologies are available within the United States.
Keywords
Basic science, Biologics, Growth factors, Mesenchymal stem cells, Platelet-rich plasma, Sports medicine
Disclosures
Laura A. Vogel declares no conflicts of interest; Mary Beth R. McCarthy receives intellectual property royalties from Arthrex, Inc.
Augustus D. Mazzocca is a paid consultant for Arthrex, Inc. and Orthofix, Inc. and receives research support from Arthrex, Inc.
Introduction
Biologic therapies have been an area of interest within sports medicine for many years as a means to maintain function in both active and aging populations. Over the last 2 decades, however, surgeon interest and industry development have significantly increased. The market for biologic therapies within orthopedic surgery was estimated to be a $3.7 billion dollar industry in 2013 and is expected to continue to increase in worth.
In general, the goal of biologic therapies is enhanced healing and restoration of normal, native tissue or anatomy. Enhanced healing can be defined as improved quality of the healing tissue, decreased healing time, or improved healing rates. Examples of restoration of normal, native tissue include restoration of articular hyaline cartilage versus fibrocartilage or normal tendon to bone insertion anatomy. The variety of available biologic therapies is vast, and there is an equally large variability in the available preparations, applications, delivery systems, and outcome measurements. Biologic therapies can be used to augment other procedures or as stand-alone treatments; they can be largely divided into growth factor treatments, cell therapies, or tissue transplantations. In this chapter, we aim to provide the reader with an overview of biologic therapies in sports medicine by reviewing historical origins of biologic therapies, currently available biologics in sports medicine, and future directions for research and treatment.
Historical Perspective
Even before the advent of cutting edge biologic therapies, surgeons have attempted to improve healing in difficult cases. Bone graft is one of the original biologic treatments. The earliest report of viable bone allograft was in 1770 by John Hunter, a Scottish surgeon and anatomist. He transplanted a bony spur from a rooster foot into its comb and found that it still grew normally owing to the rich vascular supply of the rooster’s comb. The first report of a successful human bone allograft transplantation was in 1879 by Sir William MacEwan, a British surgeon, in which he successfully reconstructed two-thirds of the humeral shaft of a 3 year old boy with extensive osteomyelitis.
The modern concept of tissue grafting and banking began in the early 1900s. Tissue was frequently solicited from patient’s friends or family, and bone was often obtained from amputees. Obtaining tissue from corpses was rarely done at that time owing to concerns regarding infection and disrupting death rites and burial rituals. The US Navy Tissue Bank was founded in 1949 and established the standard of modern tissue banks. At its inception, the bank consisted of a single small freezer in which they collected and stored surplus bone from clean cases until needed for later grafting. Issues around allograft immunogenicity and disease transmission, including human immunodeficiency virus (HIV), were prevalent in the 1960s–1980s. This led to the development of modern preservation methods and protocols for donor screening. The use of human cell, tissue, and cellular and tissue-based products in the United States is regulated by the U.S. Food and Drug Administration (FDA). In order to be classified as a low-risk product (also known as 361 low-risk products vs. high-risk 351 products) that does not require specific licensing, there are four criteria that must be met. They are (1) minimal manipulation, (2) homologous use, (3) noncombination products, and (4) lack of systemic effect. These regulations affect the availability of emerging technologies to patients and clinicians in the interest of patient safety.
Current Applications in Clinical Practice of Sports Medicine
Growth Factor Therapies and Platelet-Rich Plasma
The use of single, isolated growth factors is not common in the clinical practice of sports medicine at this time, although bone morphogenetic protein 2 and 7 have shown benefits for fracture healing in clinical trials. However, there have been a number of basic science and animal model studies investigating the effects of single, isolated growth factors in injury models that may have practical applications in sports medicine. Insulin-like growth factor I (IGF-I) has been found to be a mediator in wound healing, and Kurtz et al. demonstrated that treatment with IGF-I decreased time until functional recovery and diminished the maximal functional deficit in a rat model of Achilles tendon injury. A study by Hildebrand et al. investigated the role of platelet-derived growth factor-BB (PDGF-BB) and transforming growth factor β1 (TGF-β1) on healing in a rabbit model of injury to the medial collateral ligament. Their results showed improved ultimate load, energy absorbed to failure, and ultimate elongation of MCL injuries treated with PDGF-BB in a dose-dependent manner. Interestingly, addition of TGF-β1 to the model appeared to have a negative effect on MCL healing. Their group had previously demonstrated that PDGF-BB positively affected ligament fibroblast proliferation and that TGF-β1 enhanced collagen and total protein synthesis; thus the authors expected a potentiated improvement in healing with PDGF-BB and TGF-β1 treatment of MCL injuries. These counterintuitive results of MCL healing in their rabbit model exemplify the complexity of interactions between individual growth factors and difficulties in effective treatment in clinical practice; optimizing results will require not only understanding interactions between growth factors but dose- and time-dependent effects as well.
Platelet-rich plasma (PRP) was first recognized as an adhesive and hemostatic agent in the 1970s and as a source of autologous growth factors in the 1990s. In its early years, it was used to augment tissue healing within the fields of dentistry, oral maxillofacial surgery, and plastic surgery. While PRP has been studied for use in almost every orthopaedic subspecialty, interest has remained strong within sports medicine owing to the potential promise of decreased recovery time and earlier return to play. Early studies of PRP were plagued by inconsistencies between preparation systems regarding cell and growth factor component concentrations. Several authors have demonstrated significant differences in commercially available systems and PRP preparation method regarding concentration of leukocytes, platelets, and growth factors. Interestingly, there were some differences in concentration levels of PRP components in samples prepared with the same commercially available systems in the studies by Castillo et al. and Mazzocca et al. Classification systems of PRP by DeLong et al. and Mishra et al. separate preparations by platelet count, leukocyte count, and activation method in an attempt to help researchers better document and compare results. This may allow researchers to determine crucial information, such as the growth factor concentration needed to enhance healing or reduce inflammation.
PRP has been used extensively in sports medicine in the treatment of a variety of conditions such as cartilage lesions, osteoarthritis, elbow epicondylitis, ulnar collateral ligament injury, hamstring sprains, plantar fasciitis, patellar tendinopathy, Achilles tendinopathy, and rotator cuff tendinopathy. It has also been used to augment surgical procedures including ACL reconstruction, rotator cuff repair, and Achilles tendon repair. PRP may also have the ability to protect against the cytotoxic effects of corticosteroids and local anesthetics on chondrocytes. While there is significant variation in different preparations of PRP based on available processing systems, the generally accepted definition of PRP is “a sample of autologous blood with concentrations of platelet above baseline values.” The components of PRP that are clinically relevant include the concentration of platelets, white blood cells (WBC), and growth factors. Mazzucco et al. reported that a platelet concentration greater than 200 × 10 3 platelets/μL is sufficient to produce a therapeutic effect and that concentrations 2.5 times greater than native blood have positive effects on osteoblasts and fibroblasts in vitro. Adverse effects have been reported at doses higher than 3.5 times the platelet concentration of native blood. Giusti et al. suggested that 1.5 × 10 6 platelets/μL is the optimum platelet concentration for tissue healing. Variation in platelet as well as WBC concentration can occur between repetitive blood draws from the same individual and may affect clinical outcomes after serial treatments, which are common in clinical practice.
PRP can be created via a single or double spin centrifugation process that separates the liquid and solid components of blood; the processing method has significant impact on the concentration of platelets and WBCs. The effect of different preparations on cell proliferation varies based on target cell type. For example, an in vitro study showed that low platelet preparation has been shown to increase cell proliferation in osteocytes, myocytes, and tenocytes while a high platelet and high WBC preparation only increased cell proliferation in tenocytes. Furthermore, a high platelet and low WBC preparation increased proliferation in osteoblasts and tenocytes, but not in myocytes. Thus, different PRP preparations may be better suited to treat some conditions over others depending on the target tissue.
The literature regarding treatment of specific conditions with various PRP preparations are detailed in depth in subsequent chapters of this book. There have been conflicting results on efficacy throughout the literature. However, trends are beginning to emerge as the literature improves. For example, current thought is that low-WBC-concentration PRP may be beneficial to avoid the inflammatory reaction associated with leukocytes and thus may be preferred in treatment of conditions such as osteoarthritis where further inflammation is undesirable. Careful analysis of the literature and thorough reporting of techniques is paramount in optimizing outcomes of PRP treatment.
Cell Therapies
Stem cells are one generation more mature in cell lineage from germ cells. The four defining characteristics of stem cells are the following: (1) they are able to reproduce or have proliferative potential, (2) they are able to differentiate into different cell lines or have multipotentiality, (3) they are able to mobilize in situation of angiogenesis, and (4) they are able to activate and control cells within their environment or have paracrine functions. The regenerative properties of bone marrow were described as early as the 1860s by a Julius Cohnheim, a German pathologist, and Emile Goujon, a French physiologist. However, it was not until the mid-1900s that Alexander Friedenstein isolated mesenchymal stem cells (MSCs) in vitro, which he called CFU-F. Subsequent researchers used his protocol and created varying terminology to describe their cell lines including marrow stromal cells, bone stem cells, and mesenchymal progenitor cells. The term mesenchymal stem cells (MSCs) was coined by Arnold Caplan in the 1990s. To reduce ambiguity and variation in research and analysis of results, the International Society for Cellular Therapy introduced minimum criteria to define MSCs in a consensus statement. The criteria include four components: (1) cells must be plastic adherent in standard culture conditions, (2) cells must express CD105, CD73, and CD90 surface molecules, (3) cells must lack expression of CD45, CD34, CD14, CD11b, CD79α, CD19, and HLA-DR surface molecules, and (4) cells must be able to different to osteoblasts, adipocytes, and chondroblasts in vitro.
MSCs are of the utmost interest in orthopedics, as they are the precursors to musculoskeletal cells such as chondrocytes, tenocytes, and osteoblasts. Many uses of stem cell therapy in the United States are limited by the FDA owing to classification as 351 products. They have determined that laboratory expansion of stem cells (deemed more than minimal manipulation) and subcutaneous harvesting of adipose stem cells for intraarticular injection in the knee (nonhomologous use) are both high-risk products and have strict regulations regarding their use in the United States.
Bone marrow aspirate concentration (BMAC) has gained popularity because it is one of the few FDA-approved methods for delivering stem cells. BMAC is frequently formulated from iliac crest or tibial bone marrow. Techniques for harvesting MSCs from the proximal humerus have been described for use in arthroscopic rotator cuff repair. Subacromial bursa and the infrapatellar fat pad have also been reported as sources of MSCs. The concentration of stem cells in BMAC formulation is low and subject to hemodilution with large volume aspiration. The cell population in BMAC preparations is heterogenous and contains inflammatory cells, hematopoietic cells, endothelial cells, and nonviable cells. There are also growth factors present including platelet-derived growth factor, TGF-β, and bone morphogenetic proteins. BMAC has been for the treatment of osteoarthritis and focal chondral defects with relatively good results.
Autologous chondrocyte implantation (ACI) was first described as a two stage procedure in which chondrocytes are first harvested from a patient, cultured in a laboratory, and reimplanted into a cartilage defect at a second surgery. ACI was approved by the US FDA in 1997 for treatment of focal chondral defects of the distal femur based on promising results from 159 patients in Sweden. In response to issues of hypertrophy associated with the periosteal flap used in ACI, subsequent methods including second generation collagen-covered ACI (CCACI) and third generation matrix-associated ACI (MACI) were developed. CCACI has been used exclusively in Europe. MACI was FDA approved for use of symptomatic full thickness cartilage defects of the knee in adults in 2016. A small series with 5 year follow-up showed improvement in clinical outcome scores after treatment of cartilage defects in the knee with MACI.
Tissue Therapies
Musculoskeletal human allograft tissue is used in orthopedic sports medicine for ligament reconstruction, articular cartilage reconstruction, and meniscal transplantation. There are more than 100 accredited tissue banks in the United States. The American Association of Tissue Banks (AATB) is responsible for establishing and enforcing the standards of these institutions, which supply approximately 90% of musculoskeletal allografts used in the United States. While not all tissue banks are accredited through the AATB, all banks are required to register with the FDA and abide by their current good tissue practices (CGTP). These standards were implemented in May 2005 to help prevent the transmission of communicable diseases. All donor tissue undergoes screening for HIV, hepatitis B virus (HBV), hepatitis C virus (HCV), Treponema pallidum , and human transmissible encephalopathies.
After screening and harvest, tissues that will be kept as fresh frozen grafts, such as tendon allografts, are sterilized. Sterilization methods include disinfectant washes, ultrasonics, centrifugation, γ irradiation, and carbon dioxide exposure at low temperature and pressure. Sterilization procedures can vary between tissue banks and may affect the biomechanical properties of the tissue. Knowledge of a tissue banks sterilization process allows surgeons to be informed consumers and provide their patients with the safest possible allograft product. A survey from 2006 of members of the American Orthopaedic Society for Sports Medicine (AOSSM) found that 21% of respondents did not know if their allografts came from tissue banks accredited by the AATB and 46% did not know if the samples were sterilized or the specific sterilization process used. After processing and packaging, some tissue, such as tendon grafts or meniscus transplants, is frozen for storage as a fresh frozen graft. Tissue that is used as a fresh graft, such as osteochondral allograft, has a window of chondrocyte viability of approximately 28 days. Testing typically takes about 14 days, which allows another 14 days for graft use.
Acellular dermal matrix from human as well as bovine and porcine tissue sources has been used for augmentation of soft tissue repair such as rotator cuff repair and Achilles tendon repair. The use of whole xenograft tissue is not standard practice in orthopaedic surgery in the United States and introduces additional concerns regarding disease transmission and immune responses.
Amniotic membrane products have been used in sports medicine for cartilage restoration, ligament and tendon healing, nonoperative treatment of knee osteoarthritis, and plantar fasciitis. Amniotic membrane products are a source of stem cells and avoid many of the ethical concerns over the use of fetal stem cells. As with many products discussed in this chapter, there are proprietary processing methods of different commercially available products, which may affect their efficacy in different applications. In addition to a source of stem cells, amniotic membrane products have been investigated in enhancing tendon and ligament healing. To date, the majority of studies on amniotic membrane use in musculoskeletal conditions are in basic science or animal models with only one small randomized control trial in humans demonstrating improved outcomes with amniotic membrane treatment of plantar fasciitis compared with controls.
Particulated juvenile articular cartilage has also been used for treatment of cartilage lesions owing to the increased growth potential of juvenile chondrocytes compared with adult chondrocytes. It has the benefit of being a single stage procedure unlike ACI. A case series from 2014 by Farr et al. on the treatment of articular cartilage lesions in the knee with particulated juvenile cartilage showed improved clinical outcomes and healing with a mixture of hyaline and fibrocartilage.
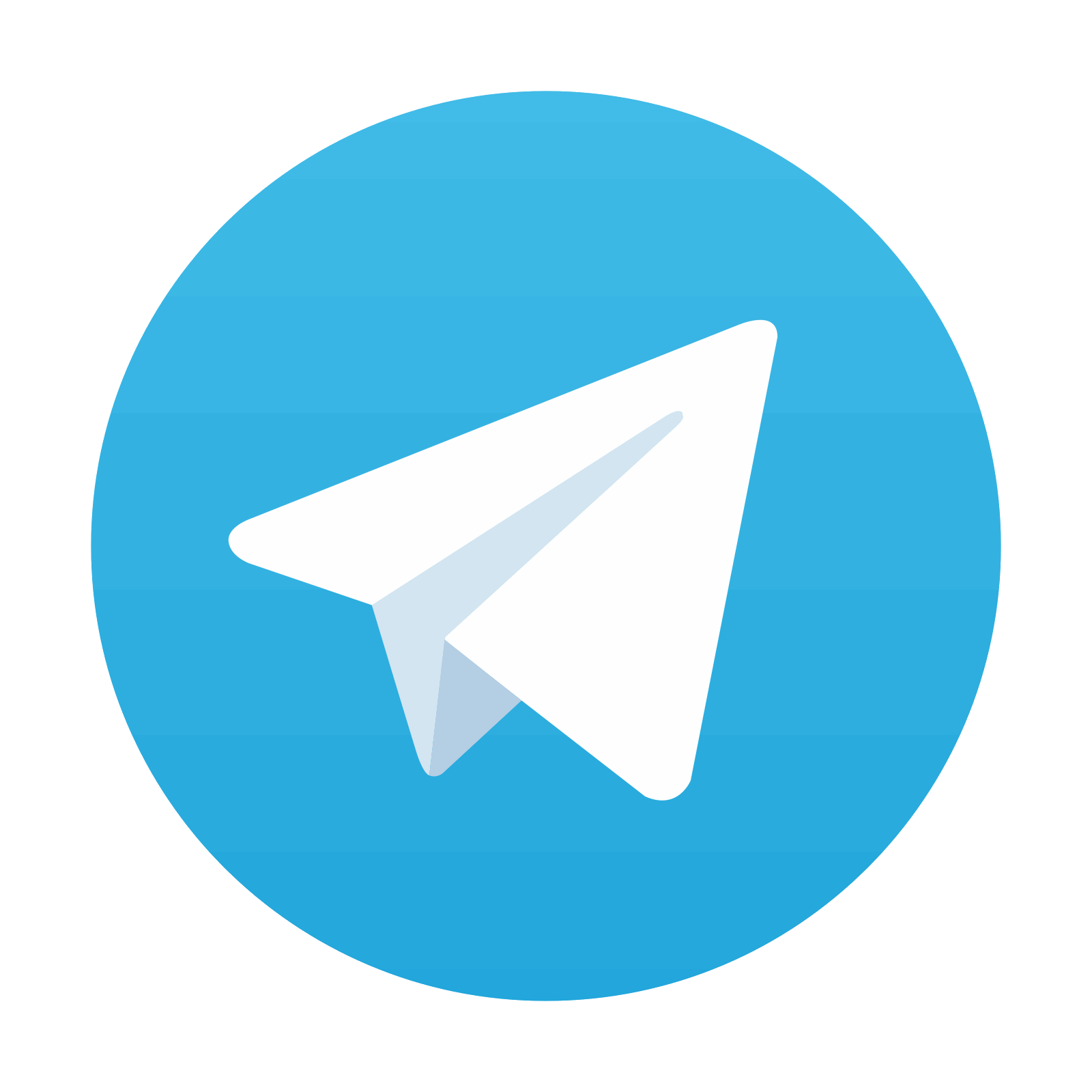
Stay updated, free articles. Join our Telegram channel

Full access? Get Clinical Tree
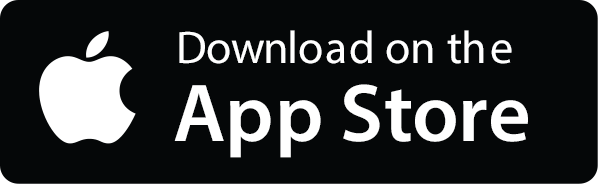
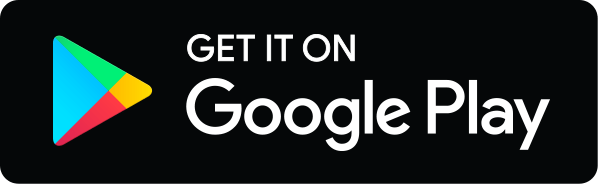