Fig. 6.1
View of the lateral aspect of the shoulder. Different-colored sutures from the medial row anchor/aspect of tendon through the patch
Once the medial sutures are passed through the graft outside the cannula, the graft is slid down the lateral cannula on top of the rotator cuff. It helps to use different colored sutures anteriorly and posteriorly so that the graft can be oriented appropriately in the subacromial space. Knots are tied to secure the graft to the tendon medially (Fig. 6.2).


Fig. 6.2
Patch secured to medial aspect of tendon
The sutures are then brought out laterally over the top of the patch/cuff and placed through a knotless anchor laterally (Fig. 6.3).


Fig. 6.3
Final construct with patch being incorporated over the tendon in a transosseous suture bridge repair construct
Postoperatively, patients are kept immobilized in an abduction sling for 4 weeks. This is followed by physical therapy for range of motion. At 3 months, strengthening is initiated.
Future Directions: Cytokines and Cells
Research has shown that growth factors and cytokines can be manipulated to enhance the healing process. PDGF-β has been found to promote fibroblast chemotaxis and proliferative activity, macrophage activation, extracellular matrix production, angiogenesis, and collagen synthesis [38]. It has also been demonstrated that PDGF-β enhances the proliferation of bone cells, which can improve the biochemical, mechanical, and structural properties of the healing site [39].
In addition to inducing osteoclast formation and bone resorption, TGF-β can enhance the proliferative activity of fibroblasts and stimulate the synthesis of type I collagen and fibronectin. TGF-β is not only found during normal fetal tendon development but also in the differentiation of scar tissue during tendon-to-bone healing. The type of healing that occurs depends on the ratio of different isoforms expressed, with TGF-β1 associated with scar-mediated healing and TGF-β3 associated with tissue regeneration and “scarless” healing [40]. When TGF-β1 is expressed during the inflammatory phase of tendon healing, there is stimulation of collagen synthesis, cell proliferation, and cell migration. Ultimately, this results in the formation of scar tissue. On the other hand, TGF-β3 expression has been linked to the development of the enthesis during prenatal stages as well as to the reduction in scar tissue formation after healing in adult wounds or tendon repairs [40, 41].
Bone morphogenetic proteins (BMP) are cytokines normally expressed during embryonic development, which participate in fibrocartilage tendon formation via a series of physiologically orchestrated signals. In particular, BMPs 2–7 have good osteoinductive properties. In contrast, recombinant human BMPs (rhBMP) 12, 13, and 14 have significantly different biologic activity that may have increased clinical benefit. These BMPs are expressed at the tendon interface during embryonic development and are primarily involved in the formation of fibrocartilage and tendon.
Lastly, FGF, expressed by fibroblasts and inflammatory cells, is involved in the promotion of cellular migration and angiogenesis to aid in proliferation and remodeling at the site of tendon repair [42].
Preclinical work on the use of growth factors to enhance tendon healing reveals a positive trend. Rodeo et al. [43] used a mixture of osteoinductive growth factors (BMP 2–7, FGF, TGF-β) in a sheep rotator cuff repair model. Biomechanical testing showed a stronger repair and increased bone and soft tissue formation at the repair site. Seeherman et al. [44] delivered rhBMP-12 via a type I/III collagen sponge to a sheep rotator cuff repair. Results showed a significantly greater load to failure and stiffness compared to controls. Histologic analysis demonstrated reestablishment of the collagen fiber continuity between the bone and fibrovascular interface scar tissue and increased glycosaminoglycan content in the rhBMP-12-treated specimens. In a rat model of rotator cuff repair augmented with FGF-2, Ide et al. [45] showed improved biomechanical and histologic outcomes at 2 weeks. However, there were no differences between experimental and control groups at 4 or 6 weeks. Uggen et al. [46] transduced rat tendon fibroblasts with PDGF and found increased DNA and collagen synthesis in transduced fibroblasts. Additionally, in a chronic rotator cuff repair model in rats, there was improved histology and biomechanics in the PDGF group. Hee et al. [47] used a sheep rotator cuff repair model with PDGF-BB + type I collagen matrix. They found an increase in ultimate load to failure in two middle dosages of PDGF. However, the highest dose group of PDGF had inferior results indicating a potential negative feedback loop and the need to elucidate an ideal concentration.
Stem Cell Therapy
Stem cells are undifferentiated, unspecialized cells that have the potential to be expanded and differentiated into various cell types in the body. When implanted, stem cells may function by direct participation in the repair process, a paracrine effect by stimulating other local (or distant) host cells, or an anti-inflammatory/immunomodulatory role. Stem cell-based approaches may be useful for augmentation of tendon-to-bone healing, tendon-to-tendon healing, and muscle regeneration and possibly reversal of fatty infiltration and muscle atrophy. Studies have indicated potential for stem cells to improve tendon healing. Ni et al. [48] created a rat patellar tendon window defect model and delivered tendon-derived stem cells in a fibrin glue carrier. The tendon-derived stem cells significantly enhanced tendon healing as evidenced by increased collagen fiber alignment and a significantly higher ultimate stress and Young’s modulus. Nixon et al. [49] isolated stem cells from adipose tissue and induced tendonitis in eight horses. Forty-two days after injection of the stem cells, there was reduced inflammatory cell infiltrate and significant improvement in tendon fiber architecture and organization.
It is likely that stem cells alone may not be sufficient for healing. In a rat rotator cuff model in which animals received bone marrow-derived mesenchymal stem cells in a fibrin carrier, Gulotta et al. [50] showed that there was no difference in fibrocartilage formation, collagen fiber organization, and biomechanical strength of the repairs, peak stress to failure, or stiffness. They concluded that the repair site may lack the cellular and/or molecular signals necessary to induce appropriate differentiation of transplanted cells. In another study, this group modified mesenchymal stem cells with membrane type 1 matrix metalloproteinase, a gene upregulated during embryogenesis in areas that develop into tendon-bone insertion sites. At 4 weeks, the modified stem cells had significantly more fibrocartilage, higher ultimate load to failure, higher ultimate stress to failure, and higher stiffness values compared with the unmodified stem cells [51]. A subsequent study by Gulotta et al. transduced stem cells with scleraxis, a transcription factor that is thought to direct tendon development during embryogenesis. Results at 4 weeks were similar to the previous study, and the authors concluded mesenchymal stem cells genetically modified with scleraxis can augment rotator cuff healing at early time points [52].
Scaffolds may provide further enhancement of cell-based approaches. In a rabbit model, Yokoya et al. [53] reconstructed a surgically created defect in the infraspinatus tendon with a polyglycolic acid (PGA) sheet seeded with MSCs or PGA alone. Their findings showed that the MSC group had a more consistent restoration of fibrocartilage and Sharpey’s fibers, improved type I to type III collagen ratio, and better tensile strength than PGA alone or control groups. However, the addition of PRP to patches does not appear to confer significant additive healing effect according to a recent study. In their rabbit model, Chung et al. [54] demonstrated that the local administration of PRP on repaired supraspinatus tendon enhanced biological tendon-to-bone healing and increased the load to failure of the repaired rotator cuff; however, porcine dermal collagen graft augmentation did not improve the biological and mechanical properties.
There is ample opportunity for impactful research in the cell-based treatment of rotator cuff tears. These approaches may be improved by targeted manipulation of the cells through culture conditions or sorting them by methods such as flow cytometry. Determining the optimal timing, concentration, and combination of different growth factors with stem cells would yield useful clinical information [55–57]. Another strategy to improve biologic augmentation involves gene therapy. For example, matrix metalloproteinase 3 has been identified as a candidate gene to enhance tendon healing through altering the postoperative/post-injury catabolic process. In sum, enhanced biological healing of the rotator cuff remains elusive, but future research should seek to find optimal methods for acquiring, processing, delivering, and maintaining autologous pluripotential cells within the healing zone [58].
Conclusion
As our understanding of the biology of healing improves, the hope is that we will be able to manipulate the rotator cuff repair milieu to improve outcomes following rotator cuff repair. In the future, this will likely come in the form of cellular strategies. At this point though, options to biologically enhance repair constructs are basically limited to platelet rich plasma and patches. To date, results of PRP augmentation in the setting of large rotator cuff repairs are not very promising. Patches can help when used in the appropriate setting.
Acknowledgement
Figures courtesy of Gary Gartsman MD.
References
1.
Nho SJ, Delos D, Yadav H, et al. Biomechanical and biologic augmentation for the treatment of massive rotator cuff tears. Am J Sports Med. 2010;38(3):619–29. doi:10.1177/0363546509343199.PubMed
2.
American Academy of Orthopedic Surgeons. Research statistics on rotator cuff repairs, national inpatient sample, 1998–2004. The Agency for Healthcare Research and Quality. 2006.
3.
Galatz LM, Ball CM, Teefey SA, Middleton WD, Yamaguchi K. The outcome and repair integrity of completely arthroscopically repaired large and massive rotator cuff tears. J Bone Joint Surg Am. 2004;86-A(2):219–24.PubMed
4.
Frank JB, ElAttrache NS, Dines JS, Blackburn A, Crues J, Tibone JE. Repair site integrity after arthroscopic transosseous-equivalent suture-bridge rotator cuff repair. Am J Sports Med. 2008;36(8):1496–503. doi:10.1177/0363546507313574.PubMed
5.
Barber FA. Platelet-rich plasma for rotator cuff repair. Sports Med Arthrosc Rev. 2013;21(4):199–205. doi:10.1097/JSA.0b013e31828a7c6a.
6.
Castricini R, Longo UG, De Benedetto M, et al. Platelet-rich plasma augmentation for arthroscopic rotator cuff repair: a randomized controlled trial. Am J Sports Med. 2011;39(2):258–65. doi:10.1177/0363546510390780.PubMed
7.
Jo CH, Shin JS, Lee YG, et al. Platelet-rich plasma for arthroscopic repair of large to massive rotator cuff tears: a randomized, single-blind, parallel-group trial. Am J Sports Med. 2013;41(10):2240–8. doi:10.1177/0363546513497925.PubMed
8.
Bergeson AG, Tashjian RZ, Greis PE, Crim J, Stoddard GJ, Burks RT. Effects of platelet-rich fibrin matrix on repair integrity of at-risk rotator cuff tears. Am J Sports Med. 2012;40(2):286–93. doi:10.1177/0363546511424402.PubMed
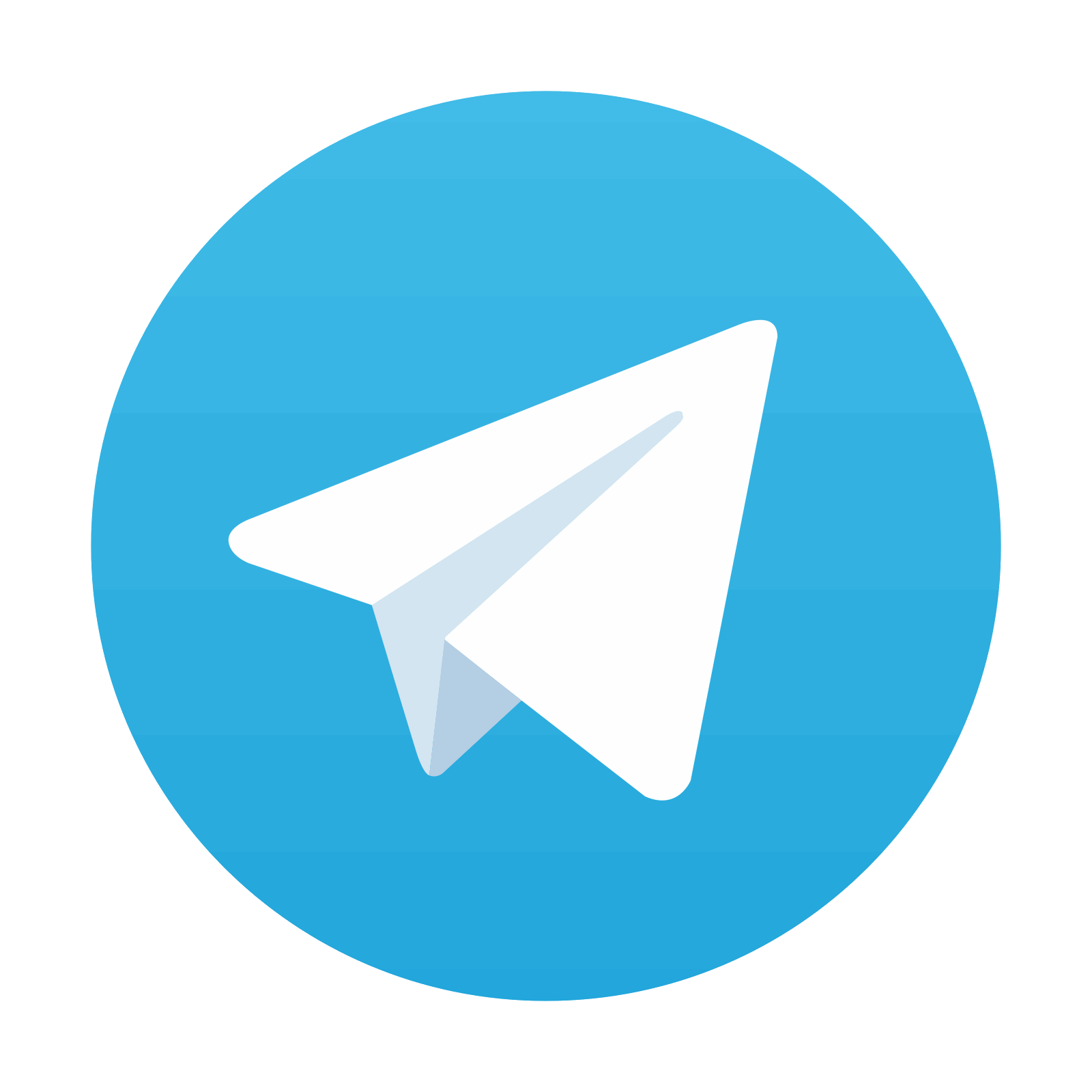
Stay updated, free articles. Join our Telegram channel

Full access? Get Clinical Tree
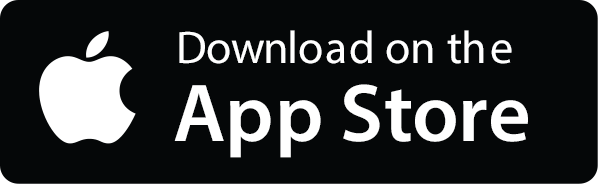
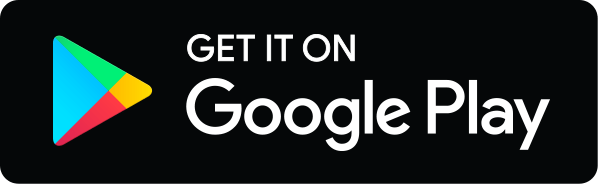