Biologic Response to Wear Debris
Christophe Nich
Stefan Zwingenberger
Stuart B. Goodman
Introduction
Total joint replacements (TJRs) are among the most successful surgical procedures. They provide predictable restoration of function in patients suffering from end-stage joint diseases, such as primary osteoarthritis, osteonecrosis, inflammatory arthropathies, and other disorders. Although worldwide standards still need to be defined, the principles of TJR currently used were established years ago. For example, Sir John Charnley (1) established in the 1960s the principles of the low-friction arthroplasty (LFA) applied to the hip, defined by the combination of a 22.22-mm diameter metallic head and an ultrahigh–molecular-weight polyethylene (UHMWPE) cemented socket. Based on this bearing couple, total hip arthroplasty (THA) survivorship reached 85% at 20 years in patients over 50 years of age, in historical series (2,3). Clinical success and increasing popularity in the surgical population have driven the indications of TJR in more and more young and demanding patients. Recently, Kurtz et al. (4) estimated that more than 50% of all TJR were done in patients less than 65 years in 2011 in the United States. In this study, projections estimated that young patients would represent 52% of all THA in 2030. However, there is a consensus that survivorship of TJR in patients less than 50 years is inferior as compared to patients over 50 years (5,6,7,8). Causes of implant failure in the mid and long term are multifactorial, including surgical technique, implant design, method of fixation, and aseptic loosening (AL) (9,10,11,12). AL secondary to periprosthetic osteolysis (OL) is one of the leading causes of revision procedures in patients with TJR (13).
AL is defined by the loss of implant fixation, resulting in prosthesis migration. Usually, AL is associated with significant periprosthetic bone loss, as the consequence of OL and adverse bone remodeling. Clinical symptoms are frequent, and drive the need for a revision procedure.
OL refers to bone destruction as seen on conventional radiographs. With respect to joint prostheses, OL may be the result of a biologic response to orthopedic wear debris, primarily from the bearing surface. It may take either a linear form or a larger and aggressive form, such as scalloping or ballooning areas (Fig. 21.1). OL is not an uncommon finding following TJR. Recently, in a series of 503 hybrid THAs, Lübbeke et al. (14) reported femoral OL in up to 24% of cases in the decade after the procedure, with more active patients at increased risk for developing osteolytic lesions. In total knee arthroplasty (TKA), OL has been found in 5% to 20% of cases, at follow-up times ranging from less than
5 years to 15 years (15). Periprosthetic OL is asymptomatic unless associated with painful synovitis, fracture, structural failure, or loosening of the implant. While there is general agreement that OL is mainly due to the production of wear debris, the precise mechanisms involved and the predisposing factors are still under investigation.
5 years to 15 years (15). Periprosthetic OL is asymptomatic unless associated with painful synovitis, fracture, structural failure, or loosening of the implant. While there is general agreement that OL is mainly due to the production of wear debris, the precise mechanisms involved and the predisposing factors are still under investigation.
In this chapter, the characteristics of wear debris generated from usage of TJRs and their biologic effects are reviewed, and the animal models, the influencing factors, and therapeutic strategies are discussed.
Orthopedic Wear Debris
The nature of the particulate debris present in the biologic environment is dependent on the materials used in the prosthesis (16,17,18). These include UHMWPE, bone cement, metal, and ceramic. Wear debris is formed at prosthetic joint articulations (the ball and socket of a normally functioning hip replacement for instance), at modular interfaces (the junction of the trunnion and ball, or polyethylene [PE] liner and metallic shell), at areas of impingement (abnormal contact between the femoral neck and the rim of the acetabular cup at extremes of motion), and at nonarticulating interfaces due to abrasion with the surrounding bone, cement mantles, or debris (19).
The pathophysiologic link connecting wear debris present around failed joint replacements and AL was established in the 1980s, based on the work by Willert (20). Histologic observations showed that the majority of wear debris present in tissues retrieved from failed TJRs was UHMWPE. Such particles originate from both the articulating or nonarticulating surfaces of TJR, and have been found around failed THA, TKA, total shoulder arthroplasties, and other artificial joints (21a,21b). Today, it is generally recognized that most instances of periprosthetic OL are caused by UHMWPE particles. For over 40 years, the metal-on-polyethylene (MOP) bearing couple has dominated the field of TJRs, and still accounts today for more than three-fourth of bearing couples used in THA in the United States. Several hundreds of thousands of PE particles may be generated during a single gait cycle (22). The relationship between PE wear and the degree of OL has been well supported by clinical data (23,24), with cementless acetabular components generating more particles than cemented ones (25). In addition, the penetration rate of the head into the PE acetabular cup has been shown to be linear over time with an average penetration rate of approximately 0.2 mm/yr. There is a strong relationship between the penetration rate and the occurrence of OL 10 years following implantation, with all patients with an average penetration rate greater than 0.3 mm/yr showing radiographic evidence of OL (26). In a long-term study of 122 Charnley THAs performed for osteonecrosis, it was shown that socket linear wear rate greater than 0.1 mm/yr was associated with a higher risk for revision for AL (27).
Studies have shown that UHMWPE particles were mainly globular spheroids in shape, ranging from 0.1 to 2.0 μm in size, with a mean 0.5 μm diameter (28). Some longer plate-like particles have also been reported, up to 250 μm in size. The spheroids may be interconnected with fibrils, forming aggregates. Noteworthy, 90% of particles are less than 1 μm (29,30), making visualization with light microscopy difficult. UHMWPE particles isolated from in vitro hip simulators appear to be predominantly submicron and round, with occasional fibrils. Also, particles in the nanometric range have been identified both in vitro (hip simulator), and in vivo (31). The latter could account for the greatest amount of particles in periprosthetic tissues.
Most of the studies reported in the literature relate to the biologic consequences of conventional UHMWPE, as opposed to highly cross-linked PE (HXLPE). HXLPE liners for THA acetabular components are now widely accepted as providing decreased wear over an extended period (32). Interestingly, head size and the nature of prosthetic head material (either zirconia, alumina, or CoCr) articulating with HXLPE socket did not show any effect on HXLPE wear rates (33). Particles issued from HXLPE appear to be largely spheroidal or oblong, with a mean 0.2 μm size (hip simulator), much smaller than the debris reported from clinical retrievals.
Metal-on-metal (MOM) THAs and resurfacings made of cobalt–chromium–molybdenum (CoCrMo) offer extremely low wear rates, but generate metallic wear particles and by-products. Wear rates of MOM couples range from 1.9 to 6.2 μm/yr. Metallic particles may be present in the biologic environment as (1) particulate wear and corrosion debris, (2) metal–protein complexes, (3) free metallic ions, (4) inorganic metal salts or oxides, and/or (5) sequestered in an organic storage form such as hemosiderin (34). Metallic wear particles have been shown to be one order of magnitude smaller than UHMWPE particles. Catelas et al. (35,36) found that wear particles produced by MOM implants from both hip simulator and those isolated from tissues, ranged from about 30 to 100 nm. They are mostly round to oval but also some are needle shaped, depending on the testing period (for in vitro) or implantation time (in vivo). These particles are in the nanometer size range (average size less than 50 nm) (37), and are more numerous compared with UHMWPE particles. Therefore, the resulting surface area is large. MOM articulations generate approximately 6.7 × 1012 to 2.5 × 1014 particles yearly, far greater than the number of particles (13,500 times) released by a conventional MOP articulation. However, the average size of the metallic particles could be even less than 50 nm, and therefore the number of particles actually produced may well have been underestimated. Despite reduced wear, the smaller size of metallic particles and the release of corrosion products have generated biologic concerns, and early adverse local tissue reactions (ALTR) have been documented (38,39,40,41,42,43).
Biologic Response to Particulate Debris
Cells in the periprosthetic environment are exposed to a continuous production of wear particles. The biologic response to particle wear debris is complex and drives the process toward periprosthetic tissue destruction and implant loosening. Although most of the studies have focused on UHMWPE particles, particles generated from other sources may induce an inflammatory reaction and subsequent OL. For example, silicate and stainless steel particles, as possible
contaminants from drilling and reaming tools, may elicit an aggressive cellular response. Although they may participate in initiating and/or instigating an inflammatory process, their role is considered minor. Alumina ceramic is a material commonly described as bio-inert. However, submicron-sized particulates of alumina and zirconia may elicit a similar but less intense reaction to those seen with submicron-sized polymers and metal debris. This subsequent section will focus on the characteristics and biologic consequences of PE (conventional and highly cross-linked) and metallic wear particulate debris.
contaminants from drilling and reaming tools, may elicit an aggressive cellular response. Although they may participate in initiating and/or instigating an inflammatory process, their role is considered minor. Alumina ceramic is a material commonly described as bio-inert. However, submicron-sized particulates of alumina and zirconia may elicit a similar but less intense reaction to those seen with submicron-sized polymers and metal debris. This subsequent section will focus on the characteristics and biologic consequences of PE (conventional and highly cross-linked) and metallic wear particulate debris.
UHMWPE Particles
Cells and Molecular Mechanisms
The biologic response of the cells to UHMWPE wear particles is primarily a function of their size, composition, surface roughness, and concentration (44). UHMWPE particles in the phagocytosable range, up to about 10 μm in size, and especially those less than 1 μm in size, appear to be most stimulatory to cells. The host reaction to PE particles is generally thought to be both nonspecific foreign body reaction and chronic inflammatory reaction, dominated by cells of the monocyte-macrophage lineage. However, other different cell types have been identified in retrieved membranes surrounding loose implants (45), including fibroblasts, lymphocytes, mesenchymal stem cells (MSCs), osteoblasts, and osteoclasts.
Macrophage Response to Wear Particles
Macrophages are the sentinels of the immune response and key participants in innate immunity. They are able to degrade protein, to process and present antigen, and, subsequently, are involved in the recruitment and activity of B and T-lymphocytes. Macrophages, upon activation by wear particulate debris, trigger the complex chain of reactions that ultimately results in bone resorption by mature osteoclasts. Macrophages, multinuclear giant cells (MNGCs), and osteoclasts are derived from circulating monocytes. MNGCs and osteoclasts, resulting from the fusion of macrophages, are specialized cells in primary phagocytosis and bone resorption, respectively.
Tissue Retrieval Studies
The presence of macrophages with internalized wear particles has been reported in the membranes retrieved from cases of failed TJR. A direct relationship between the presence of macrophages and MNGCs and the presence of UHMWPE particulate debris has been established (Fig. 21.2) (45,46,47). In addition, the concentration of particles in the periprosthetic membrane correlates with the time from implantation (48). Macrophages are usually associated with submicron particles, whereas MNGCs are associated with larger particles (>10 μm). Macrophage activation can occur with or without phagocytosis (49). In this case, particles interact with macrophages’ outer membrane through single or multiple receptors, such as CD11b, CD14, and Toll-like receptors (TLRs) family members. The TLRs are a group of evolutionarily conserved transmembrane receptors with an outer leucine-rich motif and an inner interleukin (IL)-1 receptor homologous kinase domain. Each member of the TLR family is activated by a different stimulus, which subsequently activates a response to that type of chemical sequence (50). The intracellular signaling transduction involves the activation of the mitogen-activated protein kinase (MAPK) cascade, leading to activation of the transcription factor nuclear factor kappa β (NF-κβ) and nuclear translocation, which upregulates gene expression mechanisms for proinflammatory cytokines and chemokines. Recently, it was reported that in both murine macrophage cell line and primary mouse macrophages, disruption of MyD88, an
adapter protein that couples the TLR to downstream signaling kinases, as part of the TLR signaling pathway, reduced PMMA particle–induced production of TNF-α. Similarly, MyD88−/− mice demonstrated significantly less OL than WT mice at 10 days (51).
adapter protein that couples the TLR to downstream signaling kinases, as part of the TLR signaling pathway, reduced PMMA particle–induced production of TNF-α. Similarly, MyD88−/− mice demonstrated significantly less OL than WT mice at 10 days (51).
The analysis of pseudomembranes around loose implants has shown the presence of TNF-α (52), IL-1β (53), IL-6, IL-8 (54), IL-11 (55), transforming growth factor-β (TGF-β) (56), and other inflammatory factors. Chiba et al. (57) found that TNF-α, IL-1, and IL-6 levels were higher in pseudomembranes harvested from THA associated with OL compared to THA without OL. The important role of TNF-α in particle-induced OL in vivo was shown in an experimental study by Kim et al. (58), with the finding that TNF-α mRNA expression was increased in the pseudomembrane-like tissue. Schwarz et al. (59) reported that mice that were unresponsive to TNF-α (TNFR1 receptor knockout mice) showed neither an inflammatory nor an osteolytic response to wear particles, conversely to wild-type mice. In addition, another study showed that in vivo blockade of TNF-α with etanercept (a recombinant TNF-receptor antagonist used in the treatment of rheumatoid arthritis) prevented wear debris–induced OL (60). Other inflammatory mediators found in the environment of failed THA include prostaglandin E2 (PGE2) (61), cyclooxygenase-2 (COX-2) (62), metalloproteinases (MMPs) (63), collagenase, receptor activator of NF-κβ ligand (RANKL), macrophage colony–stimulating factor (M-CSF) (64), granulocyte-macrophage colony–stimulating factor (GM-CSF) (56), chemokines monocyte chemotactic protein-1 (MCP-1, also described as CCL2/MCAF/TDCF), macrophage inflammatory protein-1α (MIP-1α) (65), vascular endothelial growth factor (VEGF), growth factors platelet-derived growth factor-α (PDGF-α) (66), and TGF-α (67). On the other hand, macrophages, MNGCs, and osteoclasts from periprosthetic tissues express receptors for IL-1, IL-6, TNF-α, M-CSF, and GM-CSF (68).
In Vitro Studies
The bioactivity induced by wear particles may be studied via the release of inflammatory mediators after macrophage stimulation by particles. The initial supposition that OL was due to cement particles led to a large number of in vitro experiments with PMMA particles. Although UHMWPE particles are the most relevant particles with respect to clinical reality, some difficulties exist with regard to their handling in vitro. Hence, their density (about 0.94 g/cm3) is slightly lower than the density of water, and therefore, UHMWPE particles may float up to the upper layer of the culture medium, resulting in an absence of contact with adherent cells to the bottom surface of the plate well. Technical options to evaluate the biologic consequences of PE particles in vitro have been proposed. For example, murine macrophage cultures in solid culture medium containing UHMWPE wear particles have been described (69). Another in vitro model employed murine macrophage cultures with UHMWPE particles fixed on the bottom of the plate with collagen protein (70). The drawback of these techniques relate to the fact that particles and macrophages cannot freely move in the culture medium, making the contact between them difficult. To increase the contact opportunities between macrophages and particles, an inverted cell culture system has been developed and appeared sufficient to induce biologic responses (71). Also, the direct coating of the plate with UHMWPE particles following the evaporation of an ethanol suspension has also been proven to provide sufficient contact between cells and particles, and to provoke an inflammatory response by macrophages (72).
In vitro studies allow for the evaluation of cell responses to particles with variable parameters, such as material, size, and concentration. For example, Green et al. (44) found that smaller PE particles (0.24 μm size) were most active in stimulating cytokine release and bone-resorbing activity in C3H murine peritoneal macrophages at a ratio of 10 μm3 per macrophage. A larger dose of the 0.45- and 1.71-μm size particles was necessary to stimulate similar levels of cytokine release and bone-resorbing activity, and particles >5 μm were inactive at all the doses tested. Petit et al. (73) reported that UHMWPE particles induced faster and larger effects on TNF-α release compared with alumina particles at similar sizes and concentrations. Other inflammatory mediators have been identified, such as PGE2, IL-1β, and IL-6 (74,75,76,77), and the results are comparable with in vivo studies.
Macrophage Recruitment/Migration
It has been assumed that the cellular reaction to wear particles, notably macrophage recruitment, was mainly a local event. Recent studies have demonstrated that both PMMA and UHMWPE particles may stimulate systemic migration of macrophages to the site of particle deposition using a bioluminescence imaging technique (78). Briefly, following the infusion of the distal femurs of nude mice with suspension of either PMMA or UHMWPE particles, reporter RAW 264.7 macrophages expressing the bioluminescent reporter gene firefly luciferase (FLuc), were injected intravenously through the tail vein. Compared with the noninjected contralateral femurs, the bioluminescent signal of femurs injected with particles of both types increased at days 6 to 8, whereas this effect could not be shown in the saline controls. Histology confirmed the large numbers of reporter macrophages within the medullary canal of mice that received the particles. In a mouse–human chimera model developed to study the role of human periprosthetic tissue in OL, Yang et al. (79) also showed marked cell trafficking by titanium-activated monocytes to an area of particle-induced inflammation. The mechanisms of macrophage trafficking have been investigated. Following UHMWPE particles’ phagocytosis, MCP-1 is abundantly released by macrophages. MCP-1 has been identified as an immediate early stress-responsive factor (17) that acts through its receptor C-C motif receptor 2 (CCR2). MCP-1 is also released by fibroblasts and bone marrow–derived osteoblasts in the presence of wear debris (80). The role of MCP-1 in local and systemic trafficking of inflammatory cells following PE particles’ stimulation has been recently reported by Gibon et al. (81). Local injection of exogenous MCP-1 into the mice distal femur stimulated systemic macrophage trafficking to bone, whereas the systemic injection of MCP-1 receptor antagonist decreased reporter cell recruitment to bone infused with PE particles. Similar effects were observed when the reporter cells were deficient in the CCR2 receptor. Therefore, the mitigation of
the MCP-1 ligand–CCR2 receptor axis appears as an interesting therapeutic strategy to modulate particle-induced OL.
the MCP-1 ligand–CCR2 receptor axis appears as an interesting therapeutic strategy to modulate particle-induced OL.
Macrophage Polarization and Particles
A current hypothesis suggests that the macrophage response in particle-induced OL may demonstrate different phenotypes, with M1 proinflammatory macrophages activated in response to wear debris production overshadowing the M2 anti-inflammatory response. The M1 activation pathway of macrophages is seen in response to type 1 T-helper cell responses (Th1) and promoted by the Th1 group of cytokines, classically IFN-γ. This results predominantly in TNF-α and IL-1 release. The alternative response is defined as “M2” and results in the production of other mediators such as IL-10, with a subsequent downregulatory effect on the inflammatory cascade. As a result, bone healing, debris scavenging, wound healing, and angiogenesis are promoted (82,83). The alternative activation pathway is driven by response to the Th2 cytokines, mainly IL-4 and IL-13. Previous work has shown that PMMA particles polarize macrophages toward an M1 proinflammatory response in vitro (84); this phenomenon can be partially reversed by the addition of IL-4. This pathway is currently investigated as a potential therapeutic strategy to polarize M1 macrophages to an M2 phenotype.
![]() Figure 21.3. Diagram of the biologic reactions associated with wear particles. TLRs, Toll-like receptors; Oc, Osteoclasts; Ob, Osteoblasts. |
The central role of macrophages and the cascade of the biologic reactions leading to bone resorption in wear debris–induced OL are shown in Figure 21.3.
Osteoblasts and Mesenchymal Stem Cells
Osteoblasts (Ob) are responsible for bone formation. MSCs are considered to be the main source of osteoprogenitor cells which become osteoblasts. Recent studies have shown reduced new bone formation in the presence of wear particles (85). In vitro experiments indicated that osteoblasts or osteoblast-like cells are able to phagocytose particles (86) and release chemokines (87) that trigger the migration of inflammatory cells. Particles influence osteoblasts in their gene expression profile, reduce matrix synthesis, and induce apoptosis (88,89).
There is cross talk between osteoblasts and osteoclasts via soluble factors. As mentioned above, wear particles
induce macrophages to a proinflammatory response. While low concentrations of TNF-α and IL-1β stimulate osteoblast proliferation, high rates and long exposure times of TNF-α inhibit their proliferation (90). Also these cytokines stimulate primary human osteoblasts to produce high amounts of M-CSF and soluble receptor activator of nuclear factor kappa B ligand (sRANKL) that promotes osteoclastogenesis.
induce macrophages to a proinflammatory response. While low concentrations of TNF-α and IL-1β stimulate osteoblast proliferation, high rates and long exposure times of TNF-α inhibit their proliferation (90). Also these cytokines stimulate primary human osteoblasts to produce high amounts of M-CSF and soluble receptor activator of nuclear factor kappa B ligand (sRANKL) that promotes osteoclastogenesis.
Wear particles also have depressive effects on the differentiation of osteoprogenitor cells to bone-forming cells. Adult MSC-like progenitor cells can be found in bone marrow as well as in adult trabecular bone. The biologic function of these progenitor cells could be critically affected by increasing amounts of wear particles, potentially diminishing the pool of osteoblasts for bone formation and osseointegration. Exposure to submicron-size titanium particles resulted in reduced osteogenic differentiation and proliferation, and enhanced apoptosis in human MSCs (91). The toxic effect of titanium particles on MSCs is strongly dose dependent, as >300 particles per cell resulted in almost complete cell death, while at <10 particles per cell showed no effects on MSCs (92). With a diminished stem cell population and compromised stem cell function, the process of osseointegration and prosthesis fixation might be severely jeopardized.
Osteoclasts
Resorption of bone tissue results from the activation of osteoclasts. Osteoclasts play a fundamental role in the regulation of bone mass, by balancing bone production by Ob. Osteoclasts are derived from hematopoietic cells of the monocyte/macrophage lineage. Osteoclasts are multinucleated specialized cells formed from the fusion of Oc progenitors. The differentiation of mononuclear cell progenitors into the Oc phenotype is paralleled by the expression of c-src proto-oncogene (93). Most inflammatory mediators released by particle-activated macrophages and present at the bone–implant interface, including PGE2, TNF-α, IL-1β, and M-CSF, are potent stimulators of osteoclastogenesis (94,95). In addition, the recruitment of pre-Oc is under the control of chemokines, such as MCP-1, MIP-1α, and CCR-1 (receptor for MIP-1α) expressed on Oc and precursors.
The essential signaling for osteoclastogenesis is the interaction of RANKL and receptor activator of NF-κβ (RANK). RANK is a membrane-bound receptor expressed on Oc precursors and mature Oc. RANK activates NF-κβ, a transcription factor that regulates numerous proinflammatory and anti-inflammatory pathways, including TNF-α and IL-1β. RANKL, a member of the TNF superfamily, is a receptor ligand expressed on the cell surface of Ob, bone marrow stromal cells, and other activated cells, including T-cells, in the inflammatory process. RANKL is also active as a soluble factor. Following RANKL–RANK interaction, numerous signaling molecules are activated, such as TRAF6, AP-1, and NFAFc1. The latter is a major regulator of Oc (96). Also, RANKL, together with M-CSF, is essential for the differentiation, maturation, and survival of bone-resorbing Oc (97). M-CSF expression by Ob stimulates the proliferation of Oc precursors. Interestingly, M-CSF alone was not sufficient to induce the differentiation of Oc precursors into mature Oc capable of bone resorption (98). Zhang et al. (99) demonstrated that TNF-α stimulation enhanced osteoclastogenesis by RANKL pretreated murine Oc precursors, in the presence of M-CSF. This effect was abrogated when the authors utilized cells from TNFR1-deficient mice. Osteoclast formation by arthroplasty-derived macrophages was inhibited by osteoprotegerin (OPG) suggesting that RANKL was involved in the formation of Oc in these tissues (100). RANKL is inhibited by the soluble decoy protein receptor antagonist OPG. OPG is mainly produced by bone marrow stromal cells, dendritic cells, and monocytes/macrophages (101). Therefore, the molecular ratio of RANK/RANKL/OPG expression appears as a key indicator for assessing bone remodeling. The role of RANK/RANKL in directing particle-induced bone resorption has been suggested in several studies. For example, OPG has been shown to inhibit in vitro murine Oc formation induced by fluid from failed THA (102). RANK signaling blockade with RANK: Fc prevented experimental particle-induced OL in an in vivo murine model (103). Clohisy et al. (104) showed that OPG completely abrogated basal and PMMA particle–stimulated osteoclastogenesis in a dose dependent and reversible manner in vitro. Interestingly, PMMA particles, RANKL, and TNF-α were all potent activators of the NF-κβ and c-jun/AP-1 signaling pathways, which are both fundamental to Oc formation.
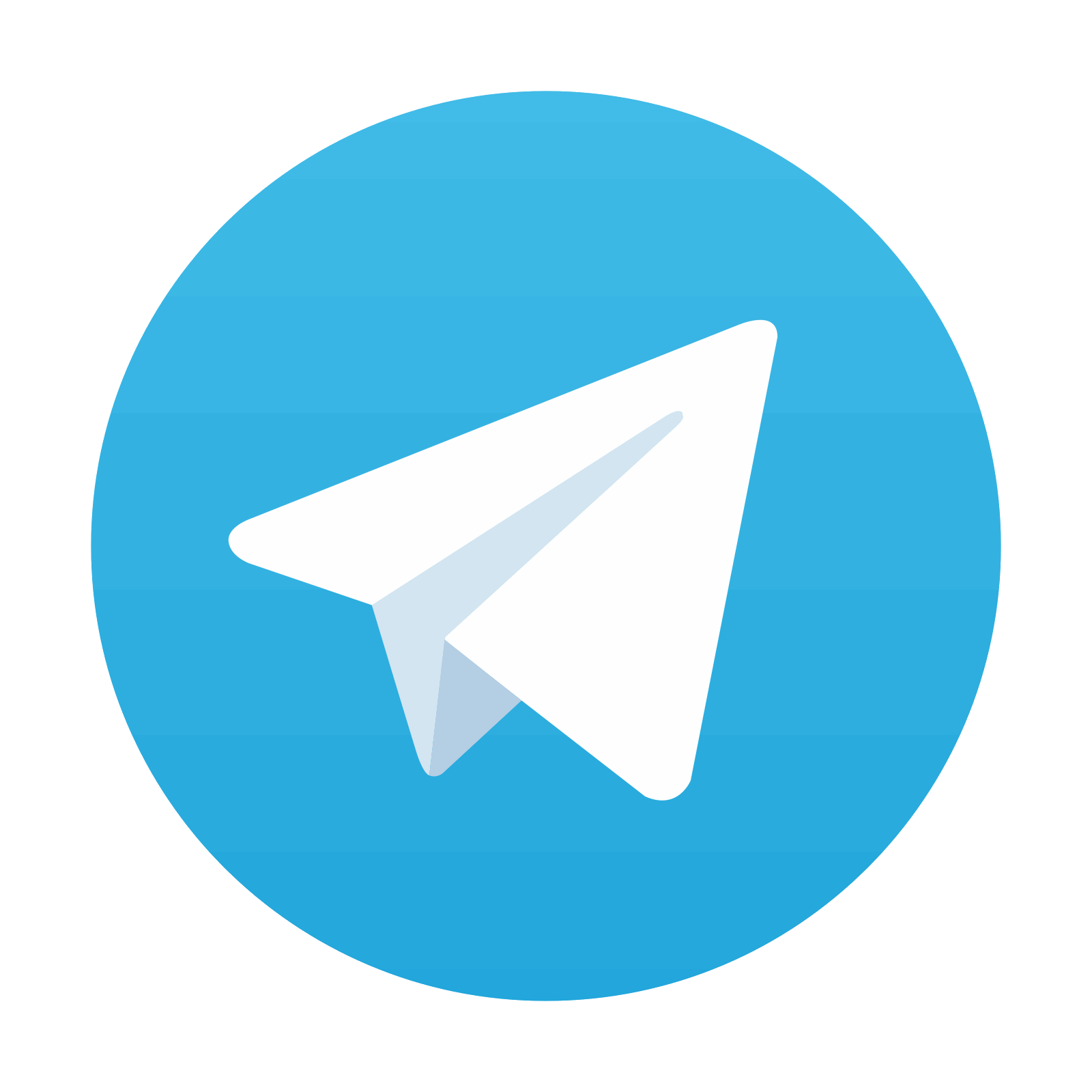
Stay updated, free articles. Join our Telegram channel

Full access? Get Clinical Tree
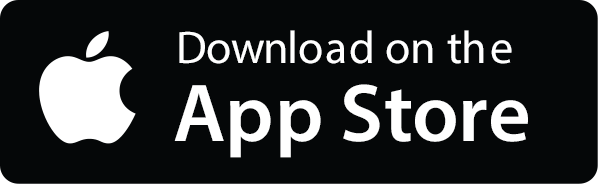
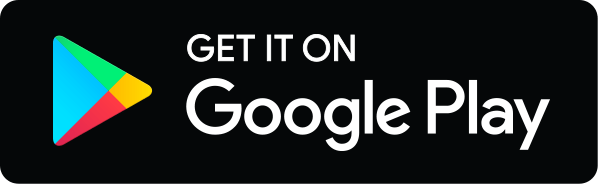
