Fig. 17.1
Advanced fatty infiltration of the rotator cuff muscle bellies following rotator cuff tear. SS supraspinatus, IS infraspinatus (Figure courtesy of Columbia University Center for Shoulder, Elbow and Sports Medicine)
In an effort to address the myriad physical and biologic challenges in performing a durable rotator cuff repair, the orthopedic research community has pursued biologic augmentation strategies. Biological augmentation describes any tissue-based or organic-synthetic device or technique which improves, supports, or directs the native healing response. Different methods of biological augmentation have been proposed ranging from novel arthroscopic techniques and instrumentation to tissue-engineered synthetic and biologic scaffolds, to systemic and local therapy, growth factors, and stem cells. This chapter will examine the principles and evidence supporting the various products that are currently available to the practicing surgeon. Emerging and promising technologies on the immediate horizon will also be discussed.
Regulatory Standards
When evaluating any bioengineered products and techniques, the clinician must be aware of the regulatory standards under which the product is marketed. Most biologic augmentation strategies for the rotator cuff can be divided broadly into cellular-/tissue-based products (e.g., stem cell injections) or synthetic medical devices (e.g., poly(lactic-co-glycolic acid) (PLGA) scaffold). The Food and Drug Administration (FDA) regulates human cell- and tissue-based products (HCT/Ps) under 21 CFR Part 1271 using a tiered risk stratified approach [8, 9]. New products meeting the regulatory definition of “articles containing or consisting of human cells or tissues that are intended for implantation, transplantation, infusion, or transfer into a human recipient” are regulated under FDA Part 361 of the Public Health Service Act (PHSA) if they are determined to be low risk or FDA Part 351 if they are considered high risk. Notably, this regulation applies only to cadaveric or living human donor-derived tissue; it does not extend to xenografts (animal based) or extracted products (e.g., growth factors). To be considered a low-risk biological product, the following four conditions must be met: (1) minimal manipulation during manufacturing, (2) homologous use: the product carries out the same biologic function it normally would, (3) noncombination product: it cannot be combined with any other substance except for simple electrolyte or preservative solutions, and (4) nonsystemic effect or autologous origin: the product must be derived from the intended recipient or a close relative to minimize the risk of an immune reaction [8, 9]. Products meeting these conditions are only required to be manufactured using Current Good Tissue Practice (CGTP) and do not require any formal pre-market review for efficacy or safety. HCT/Ps not meeting the guidelines for Part 361 approval are regulated under the more stringent Part 351. This regulatory pathway encompasses the complex pre-market approval process which requires multiple preclinical studies to establish safety and proof of concept, Investigational New Drug approval leading to regulated clinical trials, and final FDA review of safety and efficacy prior to marketing [8]. Biological augmentations such as platelet-rich plasma (PRP) and human-derived growth factors often fall under the more lenient Part 361. Stem cell therapies including autologous peripherally derived stem cells have recently fallen under increased scrutiny [8, 10]. In a February 2014 decision, the US Court of Appeals in United States v. Regenerative Sciences, LLC upheld an earlier district court ruling that autologous stem cells cultured ex vivo and later reintroduced to patients did not meet the Part 361 standard and were subject to full FDA regulation [11].
Similarly to HCT/Ps, devices such as synthetic scaffolds are regulated by the FDA under the Medical Device Amendments of 1976 to the Federal Food, Drug, and Cosmetic Act: 21 CFR 807(E). Devices are classified as Class I, II, or III in a stratified system based on risk potential with a respectively increasing level of regulatory scrutiny. Low-risk devices (Classes I and II), including most rotator cuff repair augmentation devices, are often introduced through the 510(k) process based on the concept of “substantial equivalence” to a “predicate” device already on the market. A device is considered substantially equivalent to an existing product if (1) it has the same intended use and (2) it has the same technological characteristics as the predicate device OR; if it has different technical characteristics, they do not raise new questions of safety and effectiveness and demonstrate equivalence to the predicate [12]. This 510(k) approval process does not require any preclinical proof of efficacy or controlled trials prior to marketing [13, 14]. Class III devices, those with the highest risk or that do not demonstrate substantial equivalence to an existing device, require a full pre-market approval process including preclinical trials, establishment of safety and effectiveness, and controlled human trials prior to FDA approval. Under the current FDA guidelines, most biologic and synthetic augmentations for rotator cuff repair enter the market under the less stringent regulatory bar set forth in Part 361 and 510(k) without a requirement for formal preclinical evaluation establishing safety and efficacy.
Preclinical Evaluation of Biologic Augmentations
The preclinical evaluation of rotator cuff repair augmentations relies on animal models. However, the currently available models of rotator cuff injury all have significant limitations. Ideally, an animal model should (1) mimic the biomechanics of a human shoulder while sharing functional and structural similarities, (2) be large enough to allow appropriate and reproducible surgical techniques, and (3) contain muscles, tendons, and other tissues that exhibit a similar biological response to injury as that seen in the human shoulder (e.g., fatty atrophy of chronically torn cuff muscles) [15]. The general limitations of animal models include different soft tissue and bony anatomy, biomechanics, and injury response in the forelimbs. In many animals, the acromion, clavicle, and coracoid are generally vestigial or nonexistent and do not cover the rotator cuff. Biomechanically, animals are quadrupeds and rely on the rotator cuff to both accelerate a pendular extremity and actively stabilize a weight-bearing shoulder joint. The rotator cuff tendons themselves exhibit a parallel fiber orientation (with the exception of high primates), unlike the interdigitated patterns found in humans [15]. This can lead to decreased suture purchase and compromised pullout strength. In many animals the rotator cuff tendons are extra-articular and are not exposed to the unique milieu of proteins, nutrients, and growth factors that are present in the synovial fluid and can influence healing. Finally, animals form interposed scar following cuff tearing, unlike the persistent gap and fatty atrophy of muscle found in humans with chronic tears.
The rat is one of the most frequently used models in the literature. Unlike other animals, the rat has an overriding coracoacromial arch, a well-documented genome, and readily available reagents for immunohistochemistry. Furthermore, rats tolerate bilateral procedures well and use the forelimb for limited overhead reaching [15]. However, the rat shoulder is small and unsuitable for many clinically relevant repair techniques. Rats also heal with a robust interposed scar tissue mass bridging the tear to the tuberosity and do not undergo fatty degeneration. Rabbits are less frequently used, and the majority of studies involving them are limited to histological and mechanical studies following supraspinatus detachment. However, recent studies have shown that the torn rabbit subscapularis muscle undergoes fatty infiltration and atrophy while exhibiting some biomechanical resemblance to the torn human supraspinatus complex [16]. Canines have larger shoulders allowing more accurate evaluation of clinically relevant repair techniques. In addition, these animals can tolerate casting, slinging, treadmills, and swimming and have well-established orthopedic rehabilitation protocols in the veterinary literature. However, their status as a companion animal presents ethical and social challenges to research use [17]. Sheep represent the most common large-animal model used in rotator cuff studies. The sheep infraspinatus tendon is similar in size and shape to the human supraspinatus and is readily accessible through a simple surgical approach [18]. This allows accurate reproduction of clinical techniques, the use of standard instrumentation and implants, and evaluation with accepted imaging modalities. However, unlike the human shoulder, the sheep infraspinatus tendon is completely extracapsular, necessitating a capsulotomy if exposure to synovial fluid is desired. Sheep do not have a coracoacromial arch overriding the tendon and are notoriously resistant to postoperative immobilization or reduced weight bearing, leading to almost universal rotator cuff repair failure [19]. Additionally sheep have extremely dense bone and, like other animals, form a fibrous scar in the tendon-bone gap; therefore, this model has many limitations as well [18]. Higher primates (such as the baboon and chimpanzee) have a nearly identical bony and muscular anatomy to humans; however, lower primates (including monkeys) lack the interdigitation of the rotator cuff tendons proximal to their humeral insertion [20]. These animals are immunologically similar to humans and exhibit an analogous healing response [21]. However, high cost and significant ethical considerations limit their use in orthopedic research. Cadaveric human shoulders offer the most accurate physical model, but are obviously limited to time-zero studies.
In conclusion, preclinical models are chiefly used for histological analysis of repair microstructure and biomechanical analysis of the repair construct. Unfortunately, very few studies are able to show consistent results in either realm. The quality of biomechanical testing is technique dependent and varies highly between investigators. Further, the reported metrics are inconsistent in the literature and often of limited clinical relevance. Each preclinical model used to evaluate rotator cuff augmentation devices presents unique strengths and weaknesses; unfortunately, none are ideal.
Biological Augmentation with Scaffolds and Grafts
Scaffolds and grafts have been used to augment rotator cuff repair procedures that involve large defects. These devices seek to improve the physiologic healing response, improve the physical strength of the repair by mechanical reinforcement, or both [13]. To accomplish these goals, rotator cuff scaffolds must have several characteristics : (1) Biocompatibility: the implant must not encourage an inflammatory response over its lifespan and if degradable and must not produce toxic metabolites. A favorable host response in terms of tendon regeneration, recreation of the tendon-bone interface, and formation of a functional muscle-tendon-bone unit should be achieved. (2) Mechanically robust construction: a successful scaffold must be able to both hold sutures securely and endure or transmit any applied forces. This is particularly important for augmentation-style implants which are designed to “off-load” tensile forces from the injured tendon-bone interface. (3) Surgical compatibility: the device should be implantable with reproducible surgical techniques in either an open or arthroscopic approach. The implantation technique should not be overly complex or present additional operative risk to the patient.
Based on the materials used, these grafts can be considered as biologic, synthetic, or a hybrid of both. Most biologic tendon grafts are based on decellularized allogeneic or xenogenic extracellular matrix (ECM) [22–25]. Synthetic grafts are usually made of biocompatible and biodegradable polymers that erode or break down into nontoxic metabolites in the body. Compared to ACL grafts, of which there are currently no FDA-approved commercial products, there are several rotator cuff tendon grafts available on the market. In addition, numerous efforts have been devoted to developing new tendon grafts that aim to improve tendon-bone healing. In the subsequent section, currently commercially available rotator cuff grafts and those promising technologies nearing clinical trials will be reviewed.
Commercially Available Rotator Cuff Grafts
Table 17.1 summarizes commonly used commercialized rotator cuff patches. Most of them are based on biological materials derived from ECM, such as small intestinal submucosa (SIS) and dermis. These patches provide a chemical and 3D structural framework, native matrix composition, and residual remodeling biomolecules that direct repair and remodeling of the rotator cuff tendons by the host cells [13]. However, their clinical use, especially that of SIS, has been in question due to suboptimal outcomes observed in human trials [26, 27]. Several reported adverse outcomes have been attributed to a mismatch in mechanical properties and rapid matrix remodeling inherent in the graft within the demanding and often poor host environment of the shoulder joint. A systematic comparison of four commercially available ECM patches (Restore® of porcine SIS, CuffPatch® of porcine SIS, GraftJacket® of human dermis, and TissueMend® of bovine dermis) was conducted using a canine model [28]. All four patches were inferior mechanically to the native tendon and underwent premature graft resorption. To improve the mechanical properties of rotator cuff patches, synthetic materials have been developed with the goal of providing initial mechanical reinforcement as well as lasting reinforcement over time [13]. One concern with using all-synthetic materials is the risk of an undesirable host response. Two studies have been performed to test such response of synthetic patches in canine and rat models [29, 30]. Results showed that both patches (X-Repair®, made of poly-l-lactide, and Biomerix RCR Patch®, made of polycarbonate polyurethane) showed a biocompatible host cell response with tissue infiltration and minimal inflammation response, indicating the feasibility of using this synthetic material. Hybrid rotator cuff patches are designed with the aim of combining the benefits of both poly-l-lactide and polyurethane. However, very limited data is available for the performance of these scaffolds. Currently, Tornier BioFiber-CM®, a patch made by adding bovine collagen I to poly-4-hydroxybutyrate, is in clinical trials for full-thickness rotator cuff tear repair. Trial completion is expected by June 2015.
Table 17.1
Commercially available rotator cuff augmentation grafts
Product | Material | Company |
---|---|---|
Extracellular matrix grafts | ||
Restore | SIS (porcine) | Depuy Orthopaedics |
CuffPatch | SIS (porcine cross-linked) | Organogenesis |
GraftJacket | Dermis (human) | Wright Medical |
ArthroFlex | Dermis (human) | Arthrex |
Conexa | Dermis (porcine a-Gal-reduced) | Tornier |
TissueMend | Dermis (fetal bovine) | Stryker Orthopaedics |
Zimmer Collagen Repair | Dermis (porcine cross-linked) | Zimmer |
Bio-Blanket | Dermis (bovine cross-linked) | Kensey Nash |
OrthADAPT Bioimplant | Pericardium (equine cross-linked) | Pegasus Biologics |
Synthetic grafts | ||
SportMesh Soft Tissue Reinforcement | Poly(urethaneurea) | Biomet Sports Medicine |
X-Repair | Poly-l-lactide | Synthasome |
Biomerix RCR Patch | Polycarbonate poly(urethaneurea) | Biomerix |
Hybrid grafts | ||
OrthoADAPT PR Bioimplant | Cross-linked equine pericardium with woven polymer | Pegasus Biologics |
Although rotator cuff patches are frequently used in surgery, systematic follow-up studies that evaluate performance of such scaffolds are sparse. Results from the limited number of follow-up studies available demonstrated a mixed performance of commercially available patches. Specifically, for a SIS-based scaffold (Restore®), an early study reported that when compared to preoperative levels, shoulders repaired with the Restore® patch improved in strength, motion, and function with no increased risk in infection at 24 months [31]. However, subsequent studies failed to confirm these findings. In one study, no difference between preoperative and postoperative shoulder scores was observed with use of this product [27]. Another study showed no improvement in the rate of tendon healing or clinical outcome scores compared to repair without the Restore® patch [26]. Walton et al. showed several persisting deficits with no recognizable benefit compared to controls in addition to a severe inflammatory reaction in 20% of the patients [32]. As a result, the authors concluded that SIS-based scaffolds are not recommended for rotator cuff repair [32]. The Zimmer Collagen Repair Patch® also similarly showed inconsistent performance. In one study, four patients underwent rotator cuff repair surgery augmented with this patch. Despite a promising early postoperative period, all grafts failed within 3–6 months after surgery [33]. However; in another study, repairs augmented with this product showed improved pain scores and shoulder movement compared to the preoperative shoulder and an acceptable re-tear rate [34]. Compared to the SIS-based scaffold and Zimmer Collagen Repair Patch®, published results evaluating GraftJacket® (Fig. 17.2) have demonstrated more consistent results. In a prospective, randomized study, patients with a massive rotator cuff tear repaired with GraftJacket® showed improved pain scores and a higher ratio of intact tendon at the 24-month follow-up compared to the patients with shoulders repaired without the graft [35]. In other studies, results demonstrated that augmentation with GraftJacket® led to a lower re-tear rate, improved pain score, and increased shoulder functionality compared to the preoperative condition with no inflammatory response [36–38]. Currently, only one study is available reporting the performance of the synthetic Biomerix RCR Patch®. In this study, patients showed improved pain scores, satisfactory range of shoulder movement at 6 and 12 months, a low re-tear rate (10 %), and no adverse reactions [39].
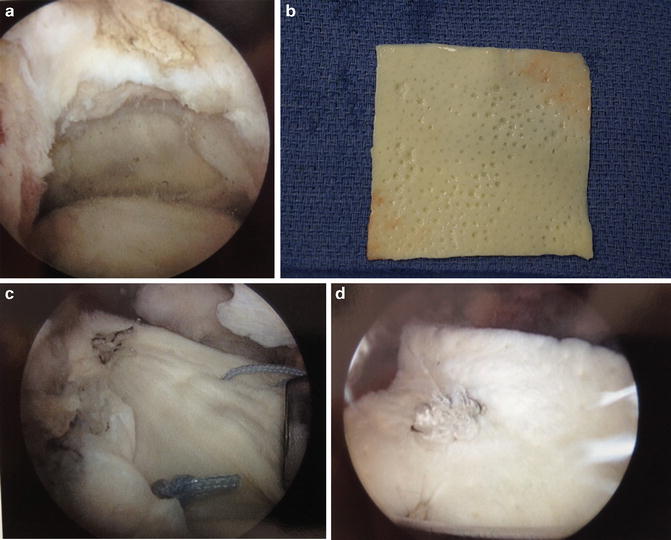
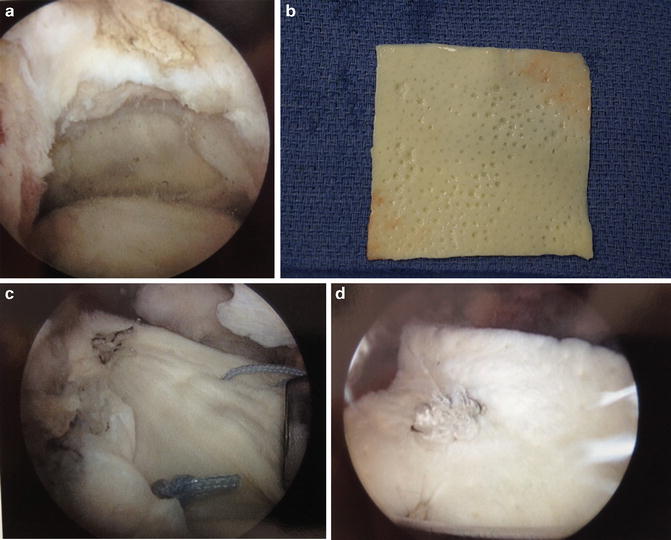
Fig. 17.2
(a) Large supraspinatus tear prior to repair. (b) GraftJacket (Wright Medical) prior to implantation. (c) GraftJacket in situ over defect. (d) Completed GraftJacket augmentation [Figure courtesy of Columbia University Center for Shoulder, Elbow, and Sports Medicine (b), Dr. John Kelly (a, c, d)]
Even with the limited number of follow-up studies performed, it is clear that despite a wide selection of commercial patches, very limited success has been found in early clinical trials. Surgical outcomes are also associated with other non-patch factors such as age of the patient, size and severity of the tear, and surgical techniques used. Surgeons should keep these factors in mind when evaluating the literature and be cautious when selecting an augmentation graft.
Tendon-Bone Insertion Scaffolds
In the rotator cuff, tendon naturally inserts to the bone through a complex fibrocartilaginous tissue. This insertion can be divided into four zones: tendon, noncalcified and calcified fibrocartilage, and bone. Each zone has distinct cell populations, matrix composition, and mechanical properties. As a result of this controlled heterogeneity, the insertion serves to minimize stress concentration, mediates load transfer, and supports communication of multiple cell types at the tendon-bone interface [40–43]. Therefore, regeneration of this multifaceted structure is an essential quality of a durable tendon graft. However, despite a variety of selections, none of the currently commercially available rotator cuff tendon products are designed for tendon-bone insertion regeneration. Rather, they focus solely on potentiating tendon repair. An augmentation that recaptures the organization of the interface, with region-dependent change in mineral content, will be highly advantageous for tendon-bone repair [44]. One way to control scaffold mineral distribution is to create a gradient of mineral in the patch. In one design, using a novel extrusion system, calcium phosphate nanoparticles were incorporated into polycaprolactone (PCL) nanofibers to create a gradient of mineral distribution across the depth of the PCL scaffold. In vitro analysis showed that when MC3T3 cells were cultured on the scaffold, a gradient of calcified matrix was formed on it within 4 weeks [45]. In another study, using a simulated body fluid immersion method, a calcium phosphate coating was deposited on a layer of gelatin-coated PCL nanofibers in a graded manner. This gradient led to controlled modulation of stiffness across the scaffold, which corresponded to varying mouse MC3T3 cell attachment on the scaffold [46]. However, these scaffold designs are still at a very early stage of development. On the other hand, Moffat et al. designed a composite nanofiber system of a poly(lactic-co-glycolic acid) (PLGA) layer and a PLGA layer loaded with hydroxyapatite (HA) nanoparticles aimed at regenerating both the noncalcified and calcified regions of the native insertion [47–49]. When seeded with bovine chondrocytes and cultured in vitro, a continuous layer of noncalcified and calcified fibrocartilaginous tissue was formed on the biphasic scaffold. Following this, a series of in vivo studies were performed to evaluate the function of the biphasic scaffold. First, biocompatibility of the scaffold and osteointegration between PLGA-HA phase and bone was confirmed. Then, the biphasic scaffold was used to repair acute, full-thickness rotator cuff tears in a rat model with results demonstrating that an insertion-like, fibrocartilaginous tissue was indeed formed only in the shoulders using the biphasic scaffold. Lastly, the efficacy of the biphasic scaffold to repair acute, full-thickness rotator cuff tears was confirmed in a sheep model. Collectively, these results demonstrate the potential of the biomimetic, biphasic scaffold for integrative, tendon-bone repair.
In summary, grafts are being clinically used and researched to augment rotator cuff repair. Currently, there are numerous commercially available products. However, caution must be taken when using these scaffolds since their performance may be inconsistent. In addition, none of them are designed specifically to fully reproduce regeneration of the tendon-bone junction, a prerequisite for integrative and truly functional rotator cuff repair. To achieve this, several research groups have been focusing on developing a scaffold that recapitulates the controlled heterogeneity of the tendon-bone insertion, specifically mineral distribution across the scaffold. Currently, a bilayered scaffold that consists of a PLGA layer and a PLGA-HA layer demonstrates a great potential for integrative and functional rotator cuff repair (Fig. 17.3).
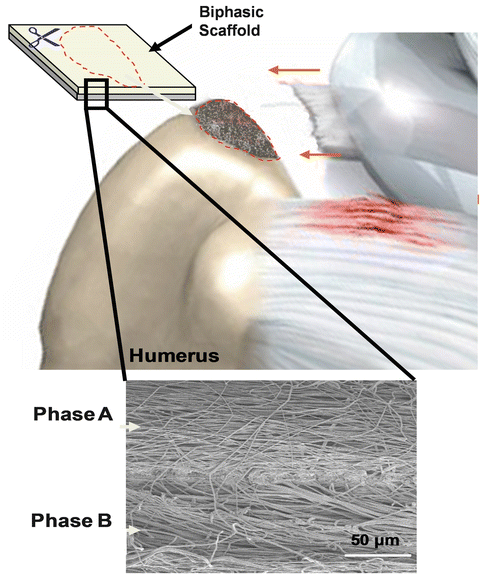
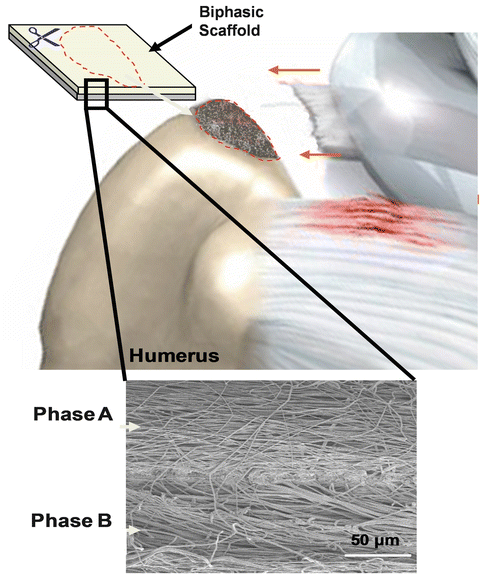
Fig. 17.3
Schematic demonstration a biologic scaffold uses as an inlay between the torn rotator cuff and the greater tuberosity footprint (Figure courtesy of Dr. Helen Lu)
Non-scaffold Biologic Augmentations
In addition to grafts, augmentation of rotator cuff repair can be achieved through biological and systemic enhancement. The three main categories of adjunctive therapy are growth factors, stem cells, and systematic interventions. In this section, current approaches in each category will be reviewed, followed by discussion of future directions and promising approaches.
Growth Factors and Cytokines
Tendon healing is a complicated and well-orchestrated process that involves several biological elements, including growth factors. Specifically, at the initial healing phase, transforming growth factor (TGF)-β is upregulated and stimulates cell migration and proliferation within the repair zone [50, 51]. Platelet-derived growth factors (PDGFs) promote expression of other excreted factors such as insulin-like growth factor (IGF)-1, which in turn further enhances the migration and proliferation of cells to the wound site [52, 53]. Basic fibroblast growth factor (bFGF) is also associated with cell proliferation and migration and is expressed by fibroblasts and inflammatory cells [54, 55]. Studies have shown that administration of such growth factors improves healing of tendon lacerations at different stages of tendon healing [56–58]. Up-regulation of the listed growth factors as well as others has been well documented in rotator cuff tendon healing studies performed in different animal models [59, 60]. Human growth hormone (HGH) has also been examined for rotator cuff repair, though the results are inconsistent. Intra-articular injection of GH improved histologic and gross appearance of focal articular cartilage injuries [61, 62]. However, when examined in a rotator cuff model, it did not improve any biomechanical parameter with daily injection and actually had a detrimental effect when dosed twice daily [63].
Augmentation of rotator cuff repair with local or systemic administration of these growth factors is being tested in vivo in a variety of studies, as listed in Table 17.2. In spite of recent advances, rotator cuff repair supplementation with growth factors remains a complicated and controversial process. First of all, in order to improve the retention rate of the growth factor at repair site, a carrier needs to be used. Therefore, in all studies, growth factors are either loaded in sutures or on a biological based matrix that act as a reservoir for sustained growth factor release. Second, the dose of growth factor is critical for the healing process. Local concentrations of the growth factor should be high enough for it to be effective, but if the concentration is too high, it could be detrimental to healing [75, 76]. In addition, healing is achieved through organized expression of a series of sequentially released growth factors, not the isolated action of any single one. Therefore, despite positive results reported in animal models thus far, single growth factor augmented repair has not gain popularity in clinical practice.
Table 17.2
Animal studies evaluating growth factor augmented rotator cuff repairs
Author | Growth factor | Animal model | Carrier type | Time point | Results |
---|---|---|---|---|---|
Chen et al. (2011) [64] | BMP-2 | Rabbit | Poly(ethylene glycol) diacrylate gel(PEGDA) with BMP-2 tethered PEG gel, mixed with periosteal progenitor cells | 4 and 8 weeks | Fibrocartilage formation and higher maximum pullout in BMP-2-loaded group |
Seeherman et al. (2008) [65] | BMP-12 | Sheep | Collagen and hyaluronic sponges | 8 weeks | Accelerated healing with BMP-12 |
Murray et al. (2007) [66] | BMP-13 | Rat | Collagen sponge | 2, 3, 4, and 6 weeks | More organized healing and higher mechanical properties at weeks 4 and 6 in BMP-13 loaded group |
Gulotta et al. (2010) [67] | BMP-13 transduced mesenchymal stem cells | Rat | Repair with 106 BMP-13 transduced MSCs | 2 and 4 weeks | No difference in cartilage formation, collagen fiber orientation and peak stress at failure, stiffness compared to non-transduced MSCs |
Dines et al. (2007) [68] | rhBMP-14 | Rat | Suture | 3 and 6 weeks | Accelerated healing at 3 weeks in rhBMP-14 loaded group |
Uggen et al. (2010) [69] | rhPDGF-BB | Sheep, repair at 2 weeks after detachment | Suture | 6 weeks | Increase in histology score but not in mechanical properties compared to without rhPDGF-BB |
Hee et al. (2011) [70] | rhPDGF-BB | Sheep | Collagen sponge loaded with rhPDGF-BB at 75, 150 and 500 μg | 12 weeks | 75 and 150 μg showed significant increase in ultimate load and tendon-bone interdigitation compared to control and 500 μg |
Ide et al. (2009) [71] | FGF-2 | Rat | Acellular dermal matrix | 2, 6, and 12 weeks | Accelerated healing with FGF-2 |
Ide et al. (2009) [72] | FGF-2 | Rat | Fibrin sealant with | 2, 4, and 6 weeks | Accelerated healing with FGF-2 |
Manning et al. (2011) [73] | TGF-β3 | Rat | Heparin/fibrin | 2, 4, and 6 weeks | Increased healing at early time points, increased mechanical properties at 4 and 6 weeks
![]() Stay updated, free articles. Join our Telegram channel![]() Full access? Get Clinical Tree![]() ![]() ![]() |