In this article, development of articular cartilage and endochondral ossification is reviewed, from the perspective of both morphologic aspects of histogenesis and molecular biology, particularly with respect to key signaling molecules and extracellular matrix components most active in cartilage development. The current understanding of the roles of transforming growth factor β and associated signaling molecules, bone morphogenic proteins, and molecules of the Wnt-β catenin system in chondrogenesis are described. Articular cartilage development is a highly conserved complex biological process that is dynamic and robust in nature, which proceeds well without incident or failure in all joints of most young growing individuals.
The histologic sequence in the formation of epiphyseal and articular cartilage is very familiar to every orthopedic surgeon. Accordingly, this article addresses the recent advances in molecular biology that are illuminating the factors that drive and control articular cartilage growth and development.
Structure and function of articular cartilage
Articular cartilage, also referred to as hyaline cartilage, is a highly specialized connective tissue with biophysical properties consistent with its ability to withstand high compressive forces. Articular cartilage is termed hyaline because of its amorphous glassy appearance ( Fig. 1 ) reflecting isotropic collagen distribution and abundant proteoglycan content. Its smooth, wear-resistant lubricated surface allows the bones to glide over one another with minimal friction and to absorb impact forces. The synovial fluid plays an important role in joint lubrication. Adult cartilage is typically avascular, alymphatic, and aneural. Nourishment is primarily provided through long-range diffusion of the synovial fluid. Immature cartilage is in proximity to blood vessels; therefore, cartilage nourishment is also provided through diffusion of nutrients from blood. In addition, cartilage canals that connect the fetal and neonatal cartilage and subchondral bone are thought to contribute to the nourishment of cartilage. During growth and development, these cartilage canals extend as branches of the blood vessels to the articular-epiphyseal cartilage that forms the articulating surface of the bone, epiphyseal center of ossification, and growth plates. Cartilage canals composed of blood vessels are seen in fetal articular cartilage. Postnatally, through the first year the cartilage canal distribution decreases and disappears.
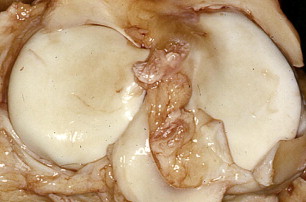
Articular cartilage is composed of an extracellular matrix with a complex macromolecular organization of predominantly collagen type II bundles intertwined with noncollagenous proteins, ions (primarily Na + and Cl − ions), and soluble negatively charged proteoglycans molecules. Collagen type II, and proteoglycans account for 15% to 22% and 4% to 7% of the cartilage wet weight, respectively.
The tissue fluid accounts for 65% to 80% of the wet weight of articular cartilage. This high fluid content enables nutrients and oxygen to diffuse through the cartilage matrix to its cells. Cartilage cells are composed of a single type termed chondrocytes, which are sparse in normal adult articular cartilage, accounting for less than 5% of the tissue wet weight. By contrast, immature cartilage is highly cellular and the cellularity decreases with the maturation. Chondrocytes are responsible for generating and maintaining the cartilaginous extracellular environment.
Collagen type II provides tensile strength to the cartilaginous matrix, and is important for the establishment of temporal and spatial organization with other matrix components such as the predominant proteoglycan termed aggrecan. Aggrecan is a composite macromolecule containing heavily sulfated glycosaminoglycans, which attract water molecules and forms large aggregates in cartilage. Aggrecan and other proteoglycans provide the cushioning capacity to the matrix, and also act to immobilize and store growth factors, thereby functioning as molecular organizers of the cartilaginous extracellular matrix.
Articular cartilage is composed of several morphologically distinct components that extend from the articulating surface (adjacent to the joint space) to the subchondral bone. These cartilage layers, also known as zones, are involved in the formation of an articulating surface and the compression-resistance core of the tissue as well as its attachment to the subjacent bone ( Table 1 ). Articular cartilage exhibits a gradation in matrix composition, collagen organization, and chondrocyte shape and aggregation from the articulating surface through to the underlying calcified cartilage, which varies with the process of cartilage maturation.
Structure/Macromolecules | Immature Cartilage (Children) | Mature Cartilage (Adult) | |
---|---|---|---|
Articular cartilage | Extracellular matrix synthesis vs degradation | Synthesis outweighs degradation | Synthesis balanced by controlled matrix degradation |
Cartilage thickness | Thick cartilage that decreases with maturation | Relatively thin articular cartilage | |
Cartilage morphology | 4 distinct zones:Zone of articular cartilage Zone of proliferation Zone of maturation Zone of calcification | 3 distinct zones of uncalcified cartilage: Superficial zone Middle zone Deep zone | |
Tidemark | Absent initially but develops as the cartilage matures | Well-demarcated lamina | |
Calcified zone | Absent | Hypertrophied chondrocytes embedded in calcified matrix | |
Vascularity | Present but decreases with cartilage maturation | Avascular | |
Growth plate | Morphology | 4 distinct zones: Zone of resting Zone of proliferation Zone of maturation Zone of calcification | Replaced by the primary tensile bone trabecula |
Articular-epiphyseal cartilage (immature)
At birth, the immature articular cartilage is thick and vascular, occupying the majority of the epiphysis. With growth and development, the immature cartilage forms a cap over the articulating ends of the epiphyses with the structural features of articular cartilage adjacent to the bursa (joint space) and epiphyseal cartilage adjacent to the subchondral bone of the epiphyses. The cartilaginous cap persists into adult life, whereas its epiphyseal component is lost.
The immature articular-epiphyseal cartilage show 4 morphologically distinct zones extending from the free surface to the subchondral bone, as follows ( Fig. 2 A, B ): (a) the zone of articulating cartilage with characteristic features of a mature cartilage, and the subsequent zones typical of the epiphyseal cartilage consisting of: (b) the zone of proliferation with active chondrocytes undergoing mitosis, (c) the zone of maturation with hypertrophic chondrocytes that accumulate glycogen and lipid and secretes alkaline phosphatase to the surrounding extracellular matrix, and (d) the zone of calcification with necrotic chondrocytes and an extracellular matrix rich in insoluble salts with traces of bone trabeculae and vascular infiltration.
In children up to the time of growth cessation, the epiphyseal cartilage or growth plate is located between the epiphysis and metaphysis. The growth plate enables bone to grow to its adult length and then is obliterated once the adult bone length is attained. Frequently, toward the end stage of bone growth (adolescent children/young adult) the epiphyseal component of the articular cartilage is still present, whereas the growth-plate cartilage disappears. The same principle applies for all mammals (see Table 1 ). The growth plate consists of 4 merging zones ( Figs. 2 C, D; 3 ), namely: (a) the zone of resting cartilage, which contains the inactive (resting) chondrocytes and functions to anchor the remaining growth plate to the epiphysis; (b) the zone of proliferation with cells actively undergoing mitosis, hence providing a continuing supply of new chondrocytes; (c) the zone of hypertrophy with enlarged cells containing glycogen and lipid; and (d) the zone of calcification that lies adjacent to the newly formed trabecular of the subchondral bone and characterized by necrotic chondrocytes embedded within calcified extracellular matrix.
Articular-epiphyseal cartilage (immature)
At birth, the immature articular cartilage is thick and vascular, occupying the majority of the epiphysis. With growth and development, the immature cartilage forms a cap over the articulating ends of the epiphyses with the structural features of articular cartilage adjacent to the bursa (joint space) and epiphyseal cartilage adjacent to the subchondral bone of the epiphyses. The cartilaginous cap persists into adult life, whereas its epiphyseal component is lost.
The immature articular-epiphyseal cartilage show 4 morphologically distinct zones extending from the free surface to the subchondral bone, as follows ( Fig. 2 A, B ): (a) the zone of articulating cartilage with characteristic features of a mature cartilage, and the subsequent zones typical of the epiphyseal cartilage consisting of: (b) the zone of proliferation with active chondrocytes undergoing mitosis, (c) the zone of maturation with hypertrophic chondrocytes that accumulate glycogen and lipid and secretes alkaline phosphatase to the surrounding extracellular matrix, and (d) the zone of calcification with necrotic chondrocytes and an extracellular matrix rich in insoluble salts with traces of bone trabeculae and vascular infiltration.
In children up to the time of growth cessation, the epiphyseal cartilage or growth plate is located between the epiphysis and metaphysis. The growth plate enables bone to grow to its adult length and then is obliterated once the adult bone length is attained. Frequently, toward the end stage of bone growth (adolescent children/young adult) the epiphyseal component of the articular cartilage is still present, whereas the growth-plate cartilage disappears. The same principle applies for all mammals (see Table 1 ). The growth plate consists of 4 merging zones ( Figs. 2 C, D; 3 ), namely: (a) the zone of resting cartilage, which contains the inactive (resting) chondrocytes and functions to anchor the remaining growth plate to the epiphysis; (b) the zone of proliferation with cells actively undergoing mitosis, hence providing a continuing supply of new chondrocytes; (c) the zone of hypertrophy with enlarged cells containing glycogen and lipid; and (d) the zone of calcification that lies adjacent to the newly formed trabecular of the subchondral bone and characterized by necrotic chondrocytes embedded within calcified extracellular matrix.
Articular cartilage (mature)
Adult articular cartilage is divided into 4 distinct zones: (a) the superficial zone, composed of thin collagen fibrils arranged parallel to the surface, and associated with a high concentration of decorin and a low concentration of aggrecan ; (b) the middle (transitional) zone, with thick collagen fibrils arranged as gothic arches; (c) the deep (radial) zone, in which the collagen bundles are thickest and are arranged in a radial fashion; and (d) the calcified cartilage zone, located between the tidemark (the interface between the uncalcified cartilage and the calcified cartilage) and the subchondral bone. The structural integrity between the more compliant articular cartilage and the rigid calcified cartilage beneath is achieved by a continuity of collagen fibers across the interface. The tidemark and the calcified cartilage serve as an interface between articular cartilage and underlying subchondral bone ( Fig. 4 ); this functions as a physical barrier for nutrient diffusion and vascularization. This interface also serves as a zone of decreased compliance, thereby facilitating the pressurization and physiologic loading of articular cartilage. The tidemark is typically absent in immature cartilage.
Chondrogenesis
Articular cartilage is a highly specialized connective tissue derived from the mesenchymal lineage. The process of chondrogenesis consists of a highly orchestrated series of events that is initiated through the precursor mesenchymal cell stimulation, followed by highly organized cellular condensation and differentiation of mesenchymal cells to chondrocytes along with secretion of extracellular matrix ( Fig. 5 ). During skeletal development, chondrogenesis is a crucial process that determines the shape and size of definitive bones in vertebrates, and results in the formation of a cartilage template (also known as the cartilage model). Chondrogenesis is then followed by a process called endochondral ossification, which includes programmed cell death and the gradual replacement of most of the cartilage model by bone formation.
Precursor Mesenchymal Cells
Mesenchymal stem cells are multipotent stem cells that can differentiate into a variety of multiple lineages and specialized cell types, including: chondrocytes, osteoblasts (bone-forming cells), adipocytes (fat cells), and myocytes (muscle cells), based on specific genetic and molecular stimulations both in vivo and in vitro. At the initiation of limb development, undifferentiated mesenchymal cells in the lateral plate mesoderm receive proliferation signals from the apical ectodermal ridge. Before condensation, the prechondrocytic mesenchymal stem cells produce extracellular matrix rich in hyaluronan and collagen type I, as well as collagen type IIA containing the exon 2–encoded amino-propeptide found in noncartilage collagens.
Mesenchymal Condensation
The aggregation of chondroprogenitor mesenchymal cells into precartilage condensations ( Fig. 6 ), first described by Fell, represents an early event in chondrogenesis. The mesenchymal condensation involves an active cellular movement that causes an increase in mesenchymal cell aggregation in close apposition with one another and migration into the core of the embryonic limb bud, resulting in an increase in cells per unit volume without an increase in cell proliferation. This event favors an increase in cell-cell contacts, and interaction through cell-cell adhesion molecules and gap junctions. In parallel, cells peripheral to the condensation region differentiate into a fibroblastic cell layer, termed the perichondrium, which in turn differentiates into bone-producing osteoblasts, forming the periosteum.
Chondrocyte Differentiation
Chondrogenic differentiation involves the specialization of mesenchymal cells into chondrocytes. Each stage of mesenchymal cell differentiation to chondrocytes is characterized by modifications in cell proliferation, morphology, and biosynthetic activity as well as secretion of the extracellular matrix macromolecules. The matrix produced by differentiated chondrocytes is essential for the formation of the future bone as it maintains and regulates the chondrocyte phenotype.
Growth of Articular Cartilage
The embryonic chondroblast (early-stage, actively synthesizing chondrocyte) secretes the extracellular matrix at its vicinity, forming a fibrous layer known as perichondrium ( Fig. 7 ). The layer of chondroblasts underneath the fibrous perichondrium secretes the cartilaginous matrix and embeds the chondroblasts, which are then termed chondrocytes. Eventually a cartilage template is formed, which persists during fetal life (see Fig. 6 ).
The growth of cartilage occurs by 2 independent mechanisms, both of which can occur simultaneously, namely the appositional growth and the interstitial growth. The appositional growth occurs at the chondrogenic level of the perichondrium where new layers of the extracellular matrix are formed on the surface of the existing cartilage. This process increases the thickness of cartilage at the level of the perichondrium. The interstitial growth, on the other hand, involves mitotic division of the existing chondrocytes and secretion of cartilage matrix by the daughter chondrocytes, resulting in the growth of the matrix surrounding the cells.
Molecular Factors Involved in Chondrogenesis
Several genes and their protein expression pattern the distribution and proliferation of mesenchymal condensations at sites of future skeletal elements (see Fig. 5 ). The molecular mechanisms underlying this complex process have been studied extensively. Several transcription factors such as Sox genes and Runx genes are crucial for chondrogenesis. Also, several signaling molecules including fibroblast growth factors (FGFs, such as FGF8), Indian hedgehog (IHH), bone morphogenetic protein (BMP), and the Wnt pathway regulate chondrogenesis through highly coordinated interactions along the 3 axes of the limb to ensure correct patterning along the dorsoventral and anteroposterior axes. Expression of genes encoding these signaling molecules are mutually regulated, and the proper limb development consists of the cooperative integration of these 3 axes.
Sox9 is a transcription factor with a high-mobility group DNA-binding domain that is expressed in all osteo-chondroprogenitors and plays an essential role in chondrogenesis. Sox9 regulates the differentiation pathways of chondrocytes and osteoblasts, and also activates chondrocyte-specific marker genes, such as Col2α1 , Col11α2 , and aggrecan. Sox9 is expressed in all chondroprogenitors and chondrocytes except hypertrophic chondrocytes. Inactivation of Sox9 during or after mesenchymal condensations causes severe chondrodysplasia, resulting in an almost complete absence of cartilage in the endochondral skeleton. Furthermore, a heterozygous mutation in the Sox9 gene has been shown to result in campomelic dysplasia, which is characterized by a general hypoplasia of the endochondral bones.
The family of Runx, a transcription factor that regulates chondrocyte differentiation, shares a highly homologous DNA-binding domain. The expression of these genes at precise times during skeletal development and chondrocyte differentiation is important, with each gene playing a distinct role in the differentiation of chondrocytes. Specifically, Runx2 and Runx3 are important regulators of chondrocyte hypertrophy, whereas Runx1 are most likely required only in the very early stages of chondrocyte differentiation. Overexpression of Runx1, Runx2, or Runx3 in nonhypertrophic chondrocytes leads to skeletal abnormalities.
FGFs play important roles in the control of embryonic and postnatal skeletal development by activating signaling through FGF receptors (FGFRs). A complex pattern of spatial regulation of FGFs and FGFRs in the different zones of the growth plate has been documented. FGFs can substitute for the apical ridge and promote limb bud outgrowth and patterning. Cross-talk between the FGFR pathway and other signaling cascades controls mesenchymal cell differentiation. Cellular differentiation of mesenchymal cells into subtypes with different FGFRs occurs in the presence of spatio-temporal variations of FGFs and transforming growth factor β (TGF-β). FGF2, FGF4, and FGF6 have been used in vitro with TGF-β to increase the proliferation and induce the differentiation of mesenchymal cells to chondrocytes. FGF and Wnt signals act together to synergistically promote proliferation; whereas, withdrawal of both signals results in cell-cycle withdrawal and chondrogenic differentiation. Genetic analyses during mouse craniofacial skeletogenesis have shown that the interplay between Wnt and FGFR1 determines the fate and differentiation of mesenchymal cells. Mutations in FGFR constitutively activate FGFR signaling, causing chondrocytes and osteoblast dysfunctions that result in skeletal dysplasias.
In mammals, the TGF-β superfamily consists of about 30 structurally related proteins; these include 3 isoforms of TGF-β itself, 3 forms of activin, and more than 20 BMPs. TGF-β is critical for maintenance of synovial joints and is among the earliest signal in chondrogenic condensation. The initiation of condensation is associated with increased hyaluronidase activity and with the involvement of 2 cell adhesion molecules, N-cadherin and neural cell adhesion molecule (N-CAM), which are Ca 2+ -dependent and Ca 2+ -independent cell-cell adhesion molecules, respectively. TGF-β stimulates the synthesis of fibronectin (which in turn regulates N-CAM) and the cell-surface adhesion protein N-cadherin. Increased concentrations of TGF-β establish fibronectin-rich domains and cause the cells within these domains to be sorted out from the surrounding mesenchyme to form direct cell-cell adhesion, resulting in mesenchymal condensation. Syndecan binds to fibronectin and downregulates N-CAM, thereby setting the condensation boundaries. Disruption of TGF-β signaling stimulates chondrocytes to increase their biosynthetic organelles, enlarging the cell, and to secrete more structural matrix molecules, resulting in articular cartilage to hypertrophy. Loss of responsiveness to TGF-β promotes terminal differentiation of chondrocytes and alterations of the cartilage matrix. N-cadherin and N-CAM disappear in differentiating chondrocytes and are detectable later only in perichondrial cells. The extracellular matrix molecules such as tenascins and thrombospondins, including cartilage oligomeric matrix protein (COMP), interact with the cell adhesion molecules to activate intracellular signaling pathways involving focal adhesion kinase and paxillin, to initiate the transition from chondroprogenitor cells to a fully committed chondrocyte.
BMPs are members of the TGF-β superfamily that play an important role in the initiation of cartilage formation. To date, almost 30 BMPs have been described and classified into several subgroups according to their structural similarities. Within the developing limb cartilage model, BMP-2, -4, and -7 have been detected in the perichondrium. Activation of the TGF-β pathway is necessary for subsequent induction of chondrogenesis by BMPs. The interaction between Activin-TGF-β and BMP pathways promotes the expression of SOX9 along with a local decrease in cell proliferation. Barna and Niswander demonstrated that BMP signaling leads to compaction of mesenchymal cells, regulating the cell cohesion in condensations. Deletion of BMP-encoding genes such as BMP-2 and BMP-4 results in the loss of precartilaginous condensation, whereas deletion of BMP receptors (BMPR) such as BMPR1a and BMPR1b leads to either the absence of most limb condensations or failure of mesenchymal cells to differentiate into chondrocytes.
Following cellular condensation the cells start differentiating into chondrocytes, a process associated with expression of cartilage-specific genes. These genes include components of cartilage extracellular matrix genes, such as those encoding collagen type II α1 (Col2α1), collagen type IX, collagen type XI, aggrecan, COMP, and LINK protein (which stabilize the proteoglycan aggregate in ground substance of cartilage matrix). Expression of these genes is regulated at the transcriptional level, spatially and temporally, so that they have different and dynamic expression patterns during chondrogenic differentiation. Cells undergoing chondrogenesis acquire a distinct spherical cell morphology and initiate expression of the transcription factors SOX9 , SOX5 , and SOX6 , which regulate the genes encoding the cartilage matrix molecules, collagen type II and aggrecan. Subsequently, the chondrocytes proliferate and secrete a cartilage-specific matrix within which the cells are encased to form the cartilage model. This matrix contains collagen type II, collagen types IX and XI, GLA protein, aggrecan (large chondroitin sulfate–rich proteoglycan), and LINK protein, while the expression of collagen type I is turned off.
Endochondral ossification
Endochondral ossification is a complex process by which the cartilaginous model formed during fetal life provides the template and contributes to the longitudinal growth of the long bones postnally. This process occurs first at the center of the cartilaginous model and later at each end by a mixture of cells that establish the primary and secondary centers of ossification in the diaphysis and epiphysis, respectively.
With continued growth of the cartilage model (through interstitial and appositional growth), the chondrocytes in its midsection hypertrophy, and with vascular penetration the hypertrophied chondrocytes begins to calcify, resulting in the formation of bone collar under the periosteum. When the calcified cartilage begins to break down, periosteal capillaries with osteogenic cells penetrate to establish the primary center of ossification in the diaphysis (mid-shaft of long bone). This process initiates the formation of the medullary cavity. Through a similar process the epiphyseal centers of ossification develop at the ends of the long bone postnatally.
Although the process of endochondral ossification begins during fetal life, the articular cartilage covering the ends of long bone emerges postnatally, and its maturation is completed by adulthood. Appearance of articular cartilage occurs at 2 weeks postnatally, when a small domain of secondary ossification occurs in the center of the epiphysis, separating the articular cartilage from the growth-plate cartilage.
The epiphyseal cartilage component at the articular surface and growth plate plays an essential role in the development and growth of long bones. At this stage, the deep zone of the newly formed articular cartilage is a growth plate–like tissue that contains a thin layer of enlarged chondrocytes expressing collagen type X (ColX), which is essential for formation of the calcified zone seen in adult articular cartilage. ColX assembly into a lattice-like network around hypertrophic chondrocytes is required for retaining or trapping the essential cartilage matrix components, including matrix vesicles and proteoglycans that promote initiation of normal mineralization. By 1 month of age, the secondary ossification center is expanded and the calcified zone starts to be formed.
In the growth plate, the zone of resting chondrocytes forms a template that attaches to the epiphyses, hence supporting the growth plate itself. The chondrocytes in this zone act as the reserve stem-like cells that replenish the pool of proliferative chondrocytes. Some groups suggest that resting zone cartilage cells also contribute to endochondral bone formation by producing a growth plate–orienting factor (morphogen) that directs the alignment of the proliferative clones into columns parallel to the long axis of the bone, and by producing a morphogen that inhibits terminal differentiation of nearby proliferative zone chondrocytes, thus helping to organize the growth plate into distinct zones of proliferation and hypertrophy.
Adjacent to the zone of resting chondrocytes is the zone of proliferation, where the chondrocytes replicate at a high rate and line up along the long axis of the bone. Following proliferation, chondrocytes pass through a transition stage in which they are known as prehypertrophic chondrocytes. Then, at the zone of hypertrophy, the chondrocytes increase in size by about 6- to 10 fold and secrete extracellular matrix that eventually becomes mineralized. The area of hypertrophic cartilage is invaded by blood vessels, bone marrow cells, osteoclasts, macrophages, and osteoblasts, the last of which deposit bone on remnants of cartilage matrix. At the zone of calcification, the hypertrophic chondrocytes undergo apoptosis shortly before the blood vessels invade the chondrocyte lacunae ( Figs. 8 and 9 ).
