Fig. 10.1
Schema of surgical procedure of cancellous bone osteotomy. Complete midsagittal osteotomy from the knee joint surface (small arrow) to the tibial diaphysis was performed using an electrically powered bone saw (a, b). The osteotomized proximal tibia was then fixed with a cerclage wiring (0.4 mm diameter) circumferentially (c). The dotted line indicates the growth plate of the proximal tibia (Reprinted with permission)
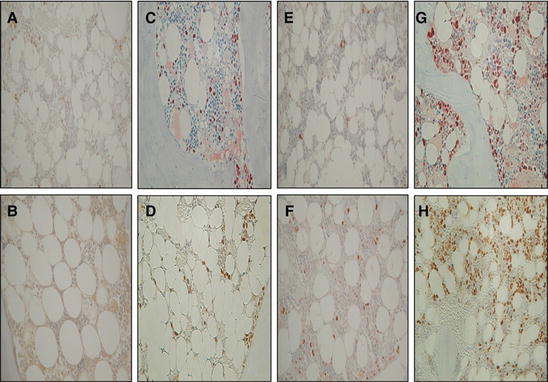
Fig. 10.2
Higher magnification of immunohistochemical analysis of PCNA at the non-osteotomy site and osteotomy site. Five randomized ROIs were analyzed at the bone marrow between the osteotomy line and endosteal surface in the proximal tibia, which corresponded to the measurement area of fat histomorphometry. PCNA-positive cells are identified as the cells stained with dark reddish-brown dye in the bone marrow. Sections at the non-osteotomy site from the vehicle-treated sham group (a), vehicle-treated OVX group (b), TPTD-treated sham group (e), and TPTD-treated OVX group (f). Sections at the osteotomy site from the vehicle-treated sham group (c), vehicle-treated OVX group (d), TPTD-treated sham group (g), and TPTD-treated OVX group (h). A large amount of PCNA-positive cells is observed in the sections from the osteotomy site (c, d, g, and h), especially in the sections from the TPTD-treated animals (g and h) (Reprinted with permission)
10.2.2 TPTD Before Osteosynthesis Stimulates Cancellous Bone Union
It has been reported that intermittent administration of TPTD promotes bone healing after surgery for osteoporotic fractures [6]. If bone healing is promoted by the administration of TPTD during the preoperative waiting period, prolonged bed rest can be prevented. Conversely, it has also been reported that pretreatment with intermittent TPTD administration is not effective for bone union [29]. However, Tsuchie et al. evaluated the effects of TPTD treatment on bone union in the period before osteosynthesis surgery after a fracture occurred [8]. Wire fixation surgery resulted in significant enhancement of bone union after an osteotomy of the proximal tibia over that of sham surgery, with an obvious effect on cancellous bone union after osteosynthesis surgery (Fig. 10.1) [6]. OVX rats underwent an osteotomy of the proximal tibia as a fracture model, and TPTD or vehicle was administered as a preoperative treatment for 1 week. After treatment, the tibiae were fixed with wire for osteosynthesis, and TPTD or vehicle was administered. A number of parameters were investigated: bone histomorphometry, Alcian Blue/hematoxylin staining, and PCNA, Sox9, and Runx2 (Figs. 10.3 and 10.4). Preoperative treatment with TPTD significantly increased bone volume, bone union, and cartilage volume. Bone union was also promoted by pre- to postoperative treatment with TPTD for 2 weeks. This treatment also significantly increased the percentage of cells positive for Runx2, but not PCNA or Sox9. Preoperative administration of TPTD enhances bone union by promoting cartilage formation and cell differentiation to osteoblasts, but not by promoting cell proliferation. A number of factors are thought to be involved in the stimulation of bone formation by TPTD, such as the promotion of Runx2, IGF-1, and activating protein-I, and the suppression of sclerostin and Smad ubiquitin regulatory factor (Smurf) [21, 35–39]. The Wnt family has also been implicated in the actions of TPTD on the bone [40].
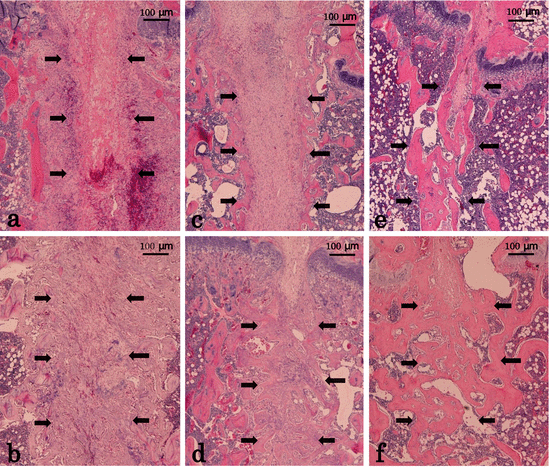
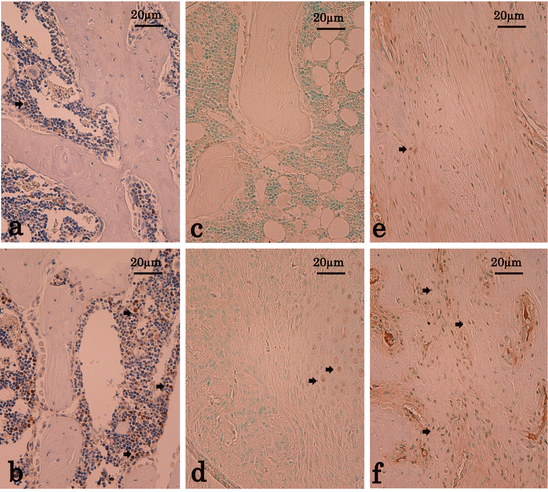
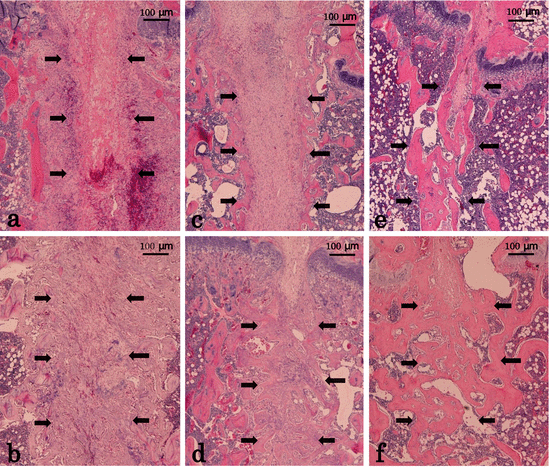
Fig. 10.3
Histological sections stained with hematoxylin and eosin at the osteotomy site. Shown are the histological sections stained with hematoxylin and eosin at the osteotomy site with a magnification of 40 x. Osteotomy sites are showed by arrows. One week after the osteotomy, the amount of new cartilage and woven bone produced in the TPTD group (b) was greater than that in the vehicle group (a). Two weeks after the osteotomy, the amount of remodeled new cancellous bones observed at the osteotomy site in the TPTD-TPTD group (d) was greater than that in the vehicle-vehicle group (c). Four weeks after the osteotomy, bone union was almost finished in both the TPTD-TPTD (f) group and vehicle-vehicle (e) group (Reprinted with permission)
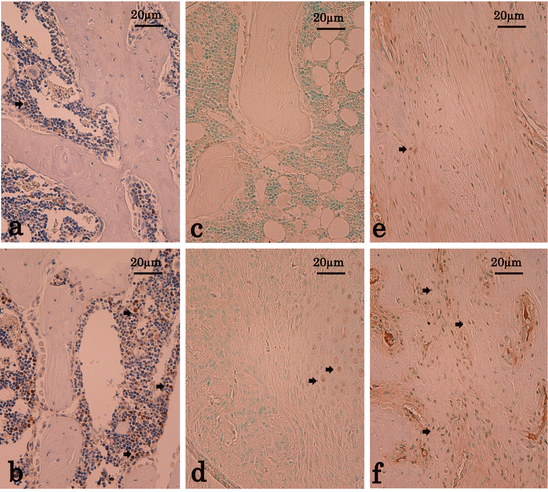
Fig. 10.4
Immunostaining for PCNA, Sox9, and Runx2 at the osteotomy site. Shown are the sections immunostained with antibodies against PCNA (a and b), Sox9 (c and d), and Runx2 (e and f) at the osteotomy site with a magnification of 200 x. Immunostained positive cells stained dark reddish-brown dye (some of them are showed by arrows). Four weeks after the osteotomy, PCNA-positive cell numbers in the TPTD-TPTD group (b) were higher than those in the vehicle-vehicle (a) group. Some Sox9-positive cells were observed in the TPTD group at 1 week (d), but there were hardly any Sox9-positive cells in the TPTD-TPTD group at 4 weeks (c). Two weeks after the osteotomy, Runx2-positive cell numbers in the TPTD-TPTD group (f) were higher than those in the vehicle-vehicle (e) group (Reprinted with permission)
10.2.3 TPTD Improves Bone-Hydroxyapatite Block Bonding
HA blocks have been widely used for the reconstruction of bone defects and as a bone substitute. Bone-implant bonding depends on both implant-related factors and patient variables. Kamo et al. evaluated whether intermittent TPTD administration enhances bone-HA block bonding in normal versus OVX rats. Cancellous bone osteotomy and HA-block implantation were performed in rats (Fig. 10.5) [7]. Newly formed cancellous bone around the HA block and bone-HA block bonding were evaluated. The administration of TPTD significantly increased cancellous bone volume by stimulating bone formation in OVX rats. Although bone-HA block bonding was significantly decreased in OVX rats compared with that of sham-operated rats, TPTD improved the bone-HA block bonding in OVX rats (Fig. 10.6). These results suggest that TPTD treatment may improve bone-HA bonding in osteoporosis by restoring cancellous bone volume and enhancing cancellous bone formation around the HA block. Figure 10.7 illustrates the HA block interface with fluorescent labels under UV light. Most of the fluorescent label at the newly formed trabecular bone on the HA blocks was observed in the sham-vehicle (Fig. 10.7a) and sham-TPTD (Fig. 10.7b) groups. TPTD treatment stimulated fluorescent labeling on the newly formed trabecular bone on the HA blocks even in the OVX groups (Fig. 10.7d). More osteoid was observed in the OVX-TPTD group (Fig. 10.7d), but not in the OVX-vehicle group (Fig. 10.7c), at the newly formed trabecular bone on the HA blocks compared to those in sham-vehicle (Fig. 10.7a) or sham-TPTD groups (Fig. 10.7b). However, there were no fluorescent labels at the interface between HA blocks and trabecular bones in any group.
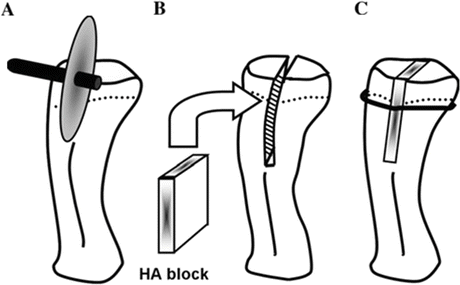
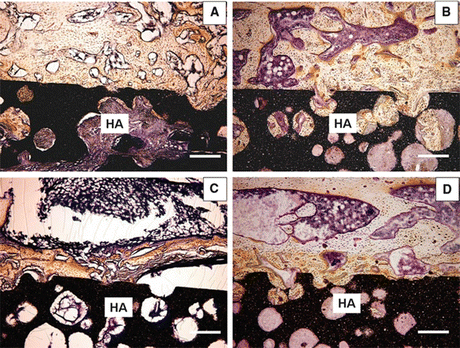
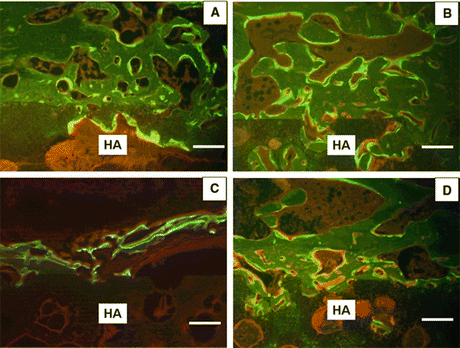
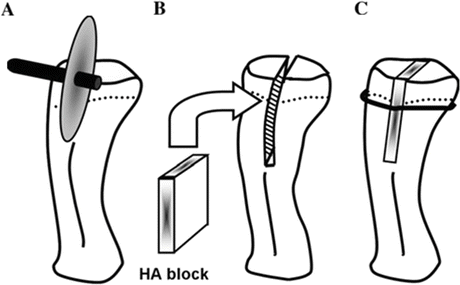
Fig. 10.5
Schema of cancellous bone osteotomy and implantation of HA block. Complete midsagittal osteotomy from the knee joint surface to the tibial diaphysis was performed using an electrically powered bone saw (a). The osteotomized proximal tibia was opened bilaterally, and the HA block was placed in the osteotomy site (b) and then fixed with a cerclage wiring (0.4 mm diameter) circumferentially (c). The dotted line indicates the growth plate of the proximal tibia (Reprinted with permission)
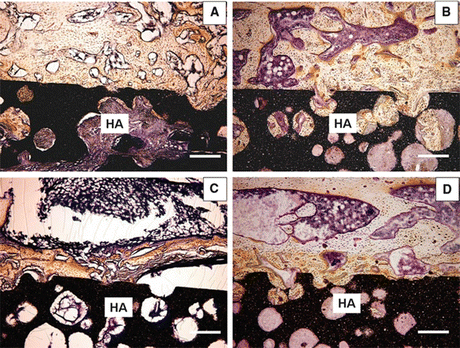
Fig. 10.6
Mid-frontal sections of the HA block interface stained with Villanueva bone stain. (a) Sham-vehicle group, (b) sham-TPTD group, (c) OVX-vehicle group, (d) OVX-TPTD group. Bars 300 μm. HA hydroxyapatite block (×40) (Reprinted with permission)
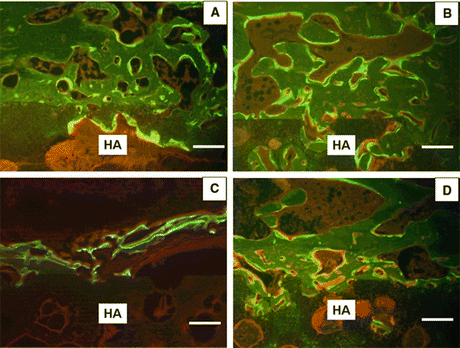
Fig. 10.7
Mid-frontal sections of the HA block interface with fluorescent labels under UV light. (a) Sham-vehicle group, (b) sham-TPTD group, (c) OVX-vehicle group, (d) OVX-TPTD group. Bars 300 μm. HA hydroxyapatite block (×40) (Reprinted with permission)
10.3 TPTD for Spine Surgery
10.3.1 TPTD Accelerates Lumbar Posterolateral Fusion
TPTD has been shown to enhance spinal fusion in women with coexisting postmenopausal osteoporosis when given after surgery for spondylolisthesis and to be more effective than oral bisphosphonates . Use of TPTD accelerated the fusion rate and shortened the duration of fusion after instrumented lumbar posterolateral fusion in women with postmenopausal osteoporosis. Ohtori et al. concluded that if other bone substitutes are not available, TPTD may be an option for improvement of spinal fusion. Preclinical data also support the use of TPTD for lumbar spinal fusion : in a rabbit model of posterolateral intertransverse arthrodesis, TPTD was shown to enhance spinal fusion after an autogenous iliac crest bone graft [41–43]. Lehman et al. reported that TPTD enhances spinal fusion, while calcitonin has a neutral effect [42]. TPTD elicited the best histologic fusion rate, while the calcitonin was similar to saline controls. Although not significant, TPTD treatment showed a strong trend toward superior radiographic fusion over calcitonin treatment in a rabbit spinal fusion model. TPTD administration increased posterolateral fusion success in rabbits [43]. Fusion bone mass and histologic determinants were also improved with TPTD treatment. These results suggest that TPTD shows promise for use as an adjunctive agent to improve spinal fusion in clinical medicine [41, 44]. Additional studies, in rats that underwent posterolateral spinal arthrodesis surgery using autologous bone grafts, showed that intermittent administration of TPTD enhanced bone turnover dominantly on bone formation at the graft site, accelerating spinal fusion [45]. This study suggests that intermittent injection of TPTD might be an efficient adjuvant intervention in spinal arthrodesis surgery and other skeletal reconstruction surgeries requiring bone grafts. Together, these studies suggest that TPTD may be one of the options for enhancing spinal fusion in women with postmenopausal osteoporosis.
10.3.2 TPTD Increases the Insertional Torque of Pedicle Screws During Fusion Surgery
The use of pedicle screws has become common in spinal surgery. However, Inoue et al. reported that, despite their clinical usefulness, they are associated with mechanical problems, such as implant breakage, screw loosening, and other related failures, sometimes requiring revision surgery [44]. The frequency of screw loosening reported in the literature varies from 0.6 to 27 % [46–49]. Considerable problems exist both with the mechanical properties of the implants and with the standardization and accuracy of the operative techniques used. Osteoporosis is a very important risk factor for pedicle screw failure because BMD is reported to be highly correlated with the stability of the pedicle screw [50, 51]. Inoue et al. reported a study where postmenopausal women with osteoporosis underwent instrumented fusion surgery with or without at least 1 month of preoperative TPTD treatment [44]. TPTD significantly increased pedicle screw insertional torque during surgery compared with the values in patients who did not receive preoperative TPTD therapy (controls). The biomechanical pullout strength of pedicle screw fixation was in direct proportion to the torque at the time of screw insertion. A high correlation was found between insertional torque and BMD. Insertional torque is significantly lower in patients with osteoporosis than in those without osteoporosis, with a negative relationship reported between insertional torque and the grade of osteoporosis [44]. TPTD affects insertional torque regardless of screw length, but the effect is greater with longer screws. TPTD, administered daily for 2 months before surgery, reduced the incidence of pedicle screw loosening after instrumented lumbar fusion in postmenopausal women with osteoporosis, suggesting that TPTD increased not only bone mass but also the quality of the pedicle cortex.
10.4 TPTD for Fractures of Extremities
10.4.1 TPTD as a Systemic Treatment for Extremity Fractures
A clinical trial using TPTD for treatment of distal radial fractures was reported in [52]. Postmenopausal women who had sustained a dorsally angulated distal radial fracture in need of dosed reduction, but without surgery, were randomly assigned to 8 weeks of placebo or TPTD. The investigators concluded that the time to healing was shorter in the TPTD-treated group. Average periods of bone union and fusion rate in the TPTD group, as evaluated by radiographic imaging and by CT, were significantly superior to those in the placebo group. Systemic administration of TPTD to accelerate fracture union is an attractive option, which becomes particularly relevant in situations in which a high surgical risk exists.
10.4.2 TPTD for Nonunion/Delayed Fractures
Most adult fractures of the extremity necessitate surgical treatment. High-energy trauma, significant soft tissue injury, inaccurate reduction, unstable fixation, infection, alcohol abuse, advanced age, diabetes, corticosteroid treatment, and osteoporosis have been reported as risk factors of nonunion [53, 54]. Use of TPTD for the treatment of nonunion, particularly atrophic nonunion, is based on its osteogenic effect and has been demonstrated in case series [10–13]. The high prevalence of nonunions in spite of improved surgical techniques has directed research efforts toward development of novel treatments to increase bone formation and accelerate fracture repair [55].
Mancilla EE et al. reported a retrospective series of patients treated for nonunion fractures of the lower extremity to evaluate the efficacy of TPTD on fracture healing and union as well as bone remodeling [56]. TPTD is approved for postmenopausal osteoporosis and glucocorticoid-induced osteoporosis in patients with high risk for fractures; therefore, this was an off-label use in most cases. TPTD led to early clinical and radiological improvement of chronic nonunion fractures of the lower extremities, with complete healing over 3–9 months. Two randomized case control trials examined whether TPTD might accelerate fracture healing when used during the initial treatment of fractures [52, 57]. On the other hand, there are only clinical case reports of one to three patients that describe the use of TPTD for management of fracture nonunion . Improved healing was observed with TPTD treatment in two cases of metatarsal stress fractures, three patients with type III odontoid fractures, an atrophic femoral nonunion, a sternal nonunion, three femoral fractures, one tibial fracture, and a humeral shaft nonunion [10, 12, 13, 58–62]. In relation to the form and dose of TPTD, most reports describe the use of TPTD, and all the trials and case reports in humans have employed doses of TPTD that are approved for treatment of postmenopausal osteoporosis. The standard parenteral dose of TPTD appears to be safe and effective in accelerating healing of lower extremity nonunion/delayed union fractures.
10.4.3 Combined Therapy with Low-Intensity Pulsed Ultrasound and TPTD for Fracture Healing
Few options exist for the conservative treatment of delayed union and nonunion fractures, including low-intensity pulsed ultrasound (LIPUS), electrical stimulation, and extracorporeal shock waves [63]. Recently, LIPUS was used for the treatment for Alagille syndrome, Charcot joint, and leg lengthening [64–68]. The good clinical healing in the present case indicates that these mechanisms are induced by LIPUS and that the effects are enough for the healing of fractures associated with this condition. Warden et al. reported that there were no interactions between TPTD and LIPUS indicating that their effects were additive rather than synergistic [69]. These additive effects were contrasting with LIPUS primarily increasing total callus volume (TV) without influencing BMC and TPTD having the opposite effect of increasing BMC without influencing TV. TPTD may have utility in the treatment of acute bone fractures, whereas LIPUS does not appear to be indicated in the management of closed, diaphyseal fractures in rat studies. Fracture healing is a complex biologic process and is impacted by multiple factors [59]. We reported a case of diaphyseal nonunion with deterioration of bone quality in long bone resolved with LIPUS and TPTD [70]. A combination of LIPUS and TPTD without a second surgical intervention was found to accelerate fracture-healing processes, with no side effects, in a nonunion patient with deteriorated bone quality.
10.4.4 Treatment of Atypical Femoral Fractures (AFFs) with TPTD
Bone remodeling suppressants like the bisphosphonates reduce bone loss and slow progression of structural decay. As remodeling removes damaged bone, when remodeling suppression is protracted, bone quality may be compromised predisposing to microdamage accumulation and atypical femoral fractures. Chiang CY et al. reported TPTD therapy assists in healing of atypical fractures and improves bone quality in patients with bisphosphonate-associated atypical femoral fractures [71]. TPTD treatment increases bone remodeling resulting in the removal of more completely mineralized bone and replacement with newly synthesized and less densely mineralized bone, the opposite sequence of events produced by remodeling suppressants [72, 73]. While remodeling intensity is increased, there is net deposition of the bone within each of the greater numbers of remodeling units as TPTD promotes the differentiation, work, and lifespan of osteoblasts in existing and newly created bone remodeling units [74]. TPTD also increases bone formation on quiescent bone surfaces [75].
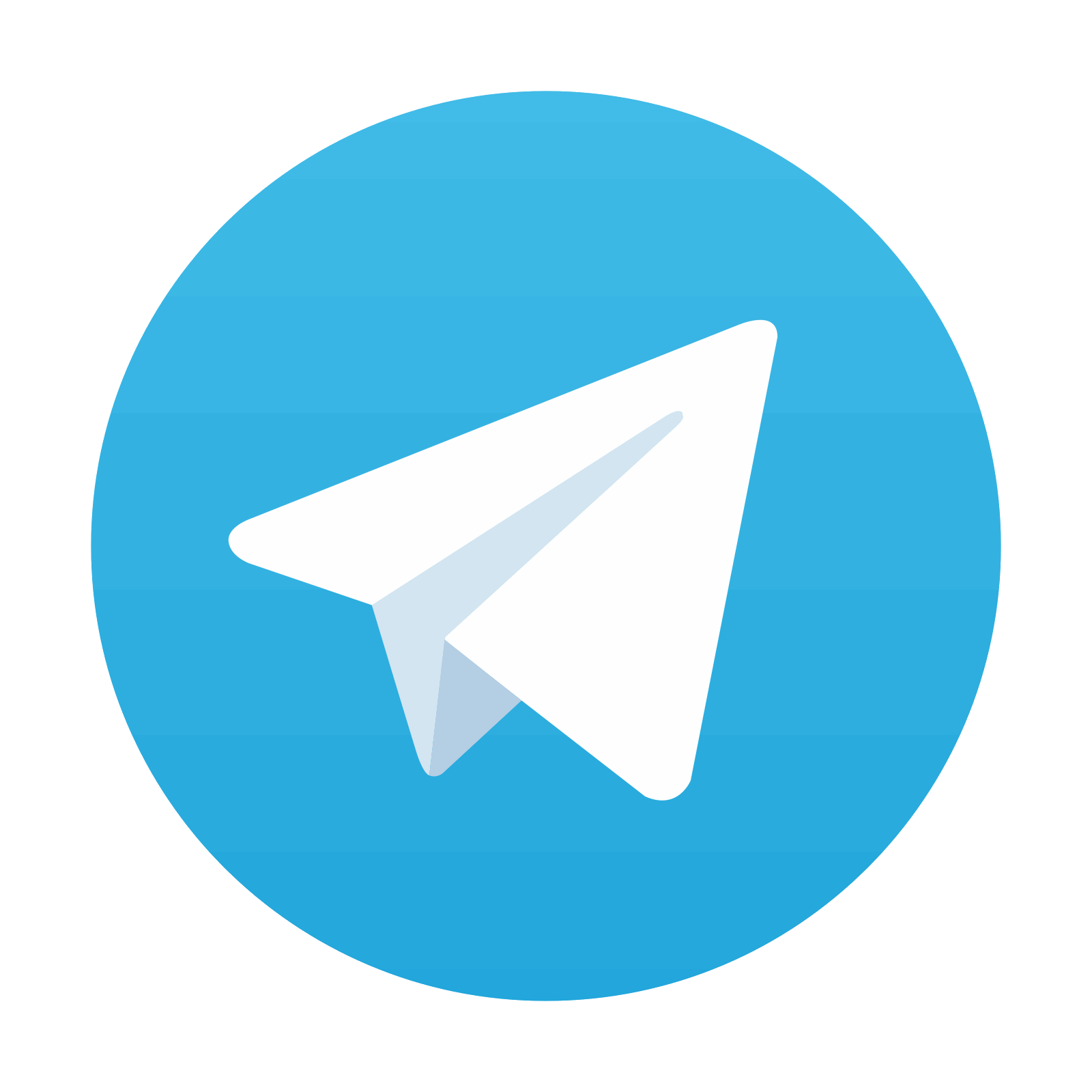
Stay updated, free articles. Join our Telegram channel

Full access? Get Clinical Tree
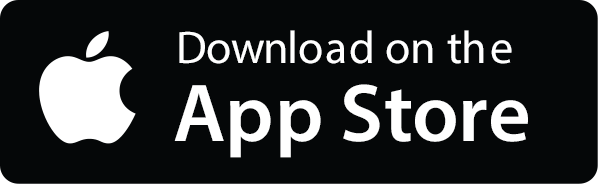
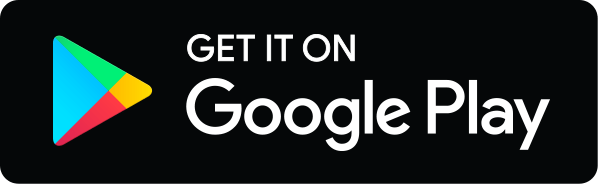