Chapter objectives
- •
Define the terminology used with isokinetics.
- •
Apply general guidelines regarding the application of isokinetic testing.
- •
Explain the specific applications of isokinetic assessment of muscular power in the upper extremities.
- •
Implement the application of isokinetics as part of rehabilitation programs.
- •
Explain the scientific and clinical rationale for the use of isokinetics in evaluation and rehabilitation of sports injuries.
Isokinetics plays a significant role in the evaluation and rehabilitation of injured athletes. The use of isokinetics has changed as interest in isokinetics has varied over the past 25 years. Isokinetics was developed in the 1960s and used increasingly during the 1970s. However, research on this subject was minimal, and the potential uses and applications of isokinetics were not clearly understood. In the 1980s the field of isokinetics came into its own, with increasing popularity and, most importantly, an increasing body of knowledge through numerous publications that supported the appropriate use of isokinetics in the testing and rehabilitation of athletes. During this period, isokinetics was used increasingly in many different areas and with many different applications. The first book dedicated solely to isokinetics was published in the early 1980 s ; it provided an overview of the testing and application of isokinetics through a combination of published research and empirically based clinical experience. However, in the 1990s there was a trend away from the use of isokinetics as part of the total evaluation and rehabilitation process. Despite extensive publications on isokinetics (more than 2000 published articles on the use and efficacy of isokinetics, an entire journal dedicated to the art and science of isokinetics [ Isokinetics and Exercise Science ], and four books dedicated exclusively to isokinetics ), many practicing clinicians have discontinued using isokinetics on the grounds that it is not functional. A PubMed search (performed on 12/16/2010) of the term isokinetics identified 4196 references. However, when the search was limited to higher levels of evidence, including randomized controlled trials, systematic reviews, and metaanalysis studies, the actual number was 513 high-quality studies. More than 220 of these articles have been published in the last decade. Interestingly, when a Google search was performed for the term isokinetics , 79,400 citations appeared. Although admittedly most athletes do not sit and flex and extend their knees as a functional activity, there is high correlation between isokinetic testing of the knee and functional testing. Unfortunately, many clinicians are disregarding the extensive documentation of isokinetics in the evaluation and treatment of athletes and are embracing closed kinetic chain (CKC) exercises as a panacea without significant documentation of efficacy. We do not advocate that only isokinetics should be used or that CKC exercises should not be used; we would, however, like to emphasize the need for an integrated approach that uses many modes of testing and rehabilitation.
Overview and terminology
Numerous modes of exercise can be used for the evaluation and rehabilitation of athletes, including isometrics, isotonics, plyometrics, isoacceleration, isodeceleration, and isokinetics.
The concept of isokinetic exercise was introduced by James Perrine in the late 1960s, and it proved to be a revolution in exercise training and rehabilitation. Instead of the traditional exercises that were performed at variable speeds against a constant weight or resistance, Perrine developed the concept of isokinetics, which involves a dynamic preset fixed speed with resistance that is totally accommodating throughout the range of motion (ROM). Since the inception of isokinetics, this form of testing and exercise has become increasingly popular in clinical, athletic, and research settings, with the first article describing isokinetic exercise being published in 1967. Since then, numerous articles and research presentations have documented the use of isokinetics for objective testing or for training.
Isokinetics means that exercise is performed at a fixed velocity (ranging from 1°/sec to approximately 1000°/sec) with an accommodating resistance. Accommodating resistance means that isokinetic exercise is the only way to dynamically load a muscle to its maximum capability at every point throughout ROM. Therefore, the resistance varies to exactly match the force applied by the athlete at every point in ROM. This is important because as the joint goes through ROM, the amount of torque that can be produced varies because of the Blix curve (musculotendinous length-to-tension ratio) and because of the physiologic changes in the length-to-tension ratio that occur in the muscle-tendon unit and in biomechanical skeletal leverage. The advantages and limitations of isokinetics are listed in Box 25-1 .
Advantages
Efficiency: It is the only way to load a dynamically contracting muscle to its maximum capability at all points throughout the range of motion.
Safety: Individuals will never meet more resistance than they can handle because the resistance is equal to the force applied.
Accommodating resistance: Accommodating resistance occurs and is predicated on changes in the musculotendinous length-to-tension ratio, changes in skeletal leverage (biomechanics), fatigue, and pain.
Decreased joint compressive force at higher speeds: This is an empiric clinical observation that one of us (G.J.D.) has made in more than 25 years of using isokinetics in testing and rehabilitation of athletes. It occurs because often an athlete exercises at a slow speed and pain develops; however, if the athlete exercises at a faster velocity, pain does not develop. Furthermore, at faster speeds, there is less time for force to develop, and the torque decreases with concentric isokinetics according to the force-velocity curve.
Physiologic overflow through the velocity spectrum: When an athlete exercises at a particular speed, a specificity response takes place, with the greatest power gains occurring at the speed of training; however, a concomitant increase in power gain occurs at other speeds as well. The majority of studies demonstrate that this phenomenon occurs at slower speeds, although some research demonstrates an overflow in both directions from the training speed.
Velocity spectrum training: As a result of the various velocities at which functional and sporting activities are performed, the ability to train at various functional velocities is important because of the specificity of training. It is important to train the muscles neurophysiologically to develop a normal motor recruitment pattern of neural contraction of the muscle.
Minimal postexercise soreness with concentric isokinetic contractions
Validity of the equipment
Reliability of the equipment
Reproducibility of physiologic testing (reliability)
Development of muscle recruitment quickness (time rate of torque development)
Objective documentation of testing
Computer feedback provided so that an athlete can train at submaximal or maximal levels
Limitations
Isolated joint/muscle testing
Nonfunctional patterns of movement
Limited velocities to replicate the actual speeds of sports performance
Increased compressive force at slower speeds
Increased tibial translation at slow speeds without proximal pad placement
Open Kinetic Chain
An open kinetic chain (OKC) assessment or rehabilitation exercise is considered to be an activity in which the distal component of the extremity is not fixed but is free in space. It is questionable whether many exercises are pure OKC, CKC, or combinations of the two. Nevertheless, an operational definition of an OKC test or exercise, within the limitations of this chapter, is one in which the distal end of the extremity is free and not fixed to an object. One of the best examples of the OKC pattern is performance of a knee flexion-to-extension pattern while sitting. This OKC pattern will serve as the model to describe OKC exercises.
Closed Kinetic Chain
A CKC assessment or rehabilitation exercise is considered to be an activity in which the distal component of the extremity is fixed. The fixed end may be either stationary or movable. An example of a CKC exercise in which the distal end is stationary is a squat exercise in which the foot is fixed to the ground. An example of a CKC exercise in which the distal end is movable is an exercise on a leg press system in which the athlete’s body is stationary and there is a movable footplate.
The acronyms OKC and CKC will be used often throughout this chapter in describing both testing and rehabilitation applications of isokinetic exercise.
Isokinetic testing
In this section some general guidelines and principles of isokinetic testing are described briefly. For more detailed information the reader is referred to A Compendium of Isokinetics in Clinical Usage and Rehabilitation Techniques .
The purposes of isokinetic testing are several: to obtain objective records, screen athletes, establish a database, quantify objective information, obtain objective serial reassessments, develop normative data, correlate isokinetic torque curves with pathologic conditions, and use the shape of the curve to individualize the rehabilitation program to a specific athlete’s needs.
Isokinetic assessment allows the clinician to objectively assess muscular performance in a way that is both safe and reliable. It produces objective criteria for the clinician and provides reproducible data for assessing and monitoring an athlete’s status. Isokinetic testing has been demonstrated to be reliable and valid. ,
Absolute and relative contraindications to testing and using isokinetics in rehabilitation must be established, as with any methodology in medicine. Examples of such contraindications are soft tissue–healing constraints, pain, limited ROM, effusion, joint instability, acute strains and sprains, and occasionally, subacute conditions.
A standard test protocol should be established to enhance reliability of the testing. Numerous considerations should be taken into account when devising such a protocol, including the following: (1) educating the athlete regarding the particular requirements of the test, (2) testing the uninvolved side first to establish a baseline and to demonstrate the requirements so that the athlete’s apprehension is decreased, (3) providing appropriate warm-ups at each speed, (4) using consistent verbal commands for instructions to the athlete, (5) having a consistent protocol for testing different joints, (6) having properly calibrated equipment, and (7) providing proper stabilization. A standard orthopedic testing protocol should be followed during isokinetic testing. Box 25-2 provides such an example.
Educate the athlete: The athlete must first be informed and educated about the purpose, procedures, and requirements of the testing.
Test the uninvolved side first: The uninvolved side is tested first to establish a baseline and to decrease the athlete’s apprehension before the involved extremity is tested.
Perform warm-ups: The athlete should perform several submaximal gradient warm-ups and at least one maximal warm-up before each test. The submaximal warm-ups (25%, 50%, and 75%) prepare the extremity for the test and allow the athlete to get a feel for the machine. The maximal effort is performed to create a positive learning transfer from a maximal warm-up to a maximal testing effort. This procedure improves the reliability of the testing sequence. ,
Give consistent verbal commands: Commands should be standardized and remain the same throughout the testing sequence to improve test-retest reliability.
Use standardized test protocols: The recommended testing protocols for each joint have been described in detail. The specific anatomic position and stabilization guidelines, range of motion, speed, and other considerations have been described.
Test at different speeds: We recommend the use of a velocity spectrum testing protocol. Velocity spectrum testing refers to testing at slow (0-60°/sec), intermediate (60-180°/sec), fast (180 to 300°/sec), and functional (300-1000°/sec) contractile velocities. Performing 3 to 5 repetitions at each speed and 20 to 30 repetitions at a fast speed (240-300°/sec) for an endurance test is recommended.
Isokinetic testing allows the use of a variety of testing protocols ranging from power to endurance tests (see Davies for a detailed description of the various isokinetic testing protocols). Our primary recommendation is to perform velocity spectrum testing so that the test will assess the muscle’s capabilities at different speeds, thus simulating various activities. Frequently, deficits in a muscle’s performance may be revealed at one speed and not at others. For example, athletes with a patellofemoral problem often have more deficits in power at slow speeds, whereas after various surgical procedures on the knee, athletes will have fast-velocity deficits.
Isokinetic data and analysis
One of the advantages of isokinetic testing is that it provides numerous objective parameters that can be used to evaluate and analyze an athlete’s performance. Various isokinetic testing data that are frequently used to analyze an athlete’s performance are peak torque, time rate of torque development, acceleration, deceleration, ROM, total work, average power, and shape of the torque curves. After these data are collected from the tests and analyzed to determine specific deficits and limitations of the athlete, the results need to be interpreted with use of the criteria presented in Box 25-3 . ,
Bilateral comparison: Comparing the involved with the uninvolved extremity is probably the most common evaluation. Bilateral differences of 10% to 15% are thought to represent significant asymmetry. However, this single parameter by itself has limitations.
Unilateral ratios: Comparing the relationship between agonist and antagonist muscles may identify particular weaknesses in certain muscle groups. This parameter is particularly important to assess with velocity spectrum testing because the percent relationships of the muscles change with changing speeds in many muscle groups. (Percent relationship means that the unilateral ratio of antagonistic muscle torque is a certain percentage of agonist muscle torque. This percentage of torque production of the antagonist to the agonist muscle changes throughout the velocity spectrum.)
Torque–to–body weight relationship: Comparing torques with body weight adds another dimension in interpreting test results. Frequently, even though bilateral symmetry and normal unilateral ratios are present, the torque–to–body weight relationship is altered.
Total leg strength: Nicholas et al, Gleim et al, and Boltz and Davies have published articles on the importance of considering the entire kinetic chain concept of total leg strength.
Comparison to normative data: Although the use of normative data is controversial, if properly used relative to a specific population of athletes, it can provide guidelines for testing or rehabilitation.
Recent research has demonstrated the possible relationship between the isokinetic torque curve and joint function. Bryant et al indicated that specific characteristics of the isokinetic torque curve of the knee extensor (extensor torque smoothness) may provide valuable clinical information regarding joint function. The morphology of knee extension torque-time curves demonstrated that following reconstruction of the anterior cruciate ligament (ACL), the involved knee had significant deficits. Eitzen et al evaluated isokinetic quadriceps strength profiles in ACL-deficient potential copers and noncopers. The results demonstrated that the peak torque did not identify the largest quadriceps muscle strength deficit; rather, it was established at knee flexion angles of less than 40°. This resulted in significant differences in angle-specific torque values between potential copers and noncopers. Furthermore, moderate to strong associations were disclosed between angle-specific torque values and single-legged hop performance, but only for the noncopers. Eitzen et al concluded that interpretation of isokinetic curve profiles seems to be of clinical importance for the evaluation of quadriceps muscle performance after ACL injury. Interestingly, more than a quarter of a century ago in the first book dedicated to isokinetics, entire chapters were dedicated to isokinetic analysis, including angle-specific torques and shapes of the torque curves.
Although it is beyond the scope of this chapter to completely review all aspects of data interpretation, the reader is referred to several key texts , , and Ellenbecker and Davies for a more comprehensive review of the basic tenants of interpretation of data from isokinetic tests of the upper and lower extremity, as well as the trunk.
Rationale and need for isokinetic testing and rehabilitation
Even though the purpose of this chapter is to describe the rationale and need for isokinetic rehabilitation, a few comments about why CKC exercises should be used instead of just OKC exercises are necessary. Many articles have described the rationale for using CKC exercises, particularly in rehabilitating athletes after ACL reconstruction. , However, Crandall et al performed a metaanalysis of 1167 articles published between 1966 and 1993 on the treatment of athletes with ACL injuries and found only 5 articles (and 3 of these articles included data on the same athletes) that met the criteria for metaanalysis of prospective, randomized, controlled, experimental clinical trials. Consequently, many of the articles that are commonly thought of as “definitive treatment articles” are simply descriptive studies. Therefore, although the benefits of using CKC exercises in rehabilitation have been described quite extensively, few scientifically based prospective, randomized, controlled, experimental clinical trials have documented the efficacy of CKC exercises. The reader is referred to a text that outlines these research studies and the application of CKC exercise as a complement to the material presented here on OKC isokinetic training and testing.
The rationale for the use of CKC exercise only is thus founded not on scientific studies that have documented its efficacy but more on unverified empiric observations and descriptive studies.
Rational for open kinetic chain isokinetic assessment in the lower extremity
Despite the many disadvantages described for OKC assessment, there are still several reasons why OKC exercises should be incorporated in both assessment and rehabilitation, as listed in Box 25-4 . , , , , , ,
It is necessary to perform isolated testing of specific muscle groups usually affected by certain pathologic changes. If the component parts of the kinetic chain are not measured, the weak link will not be identified or adequately rehabilitated, which will affect the entire chain. ,
Muscle groups away from the specific site of injury must be assessed to determine other associated weaknesses (e.g., disuse or preexisting problems). ,
CKC or total extremity testing may not demonstrate the true weakness that exists; proximal and distal muscles often compensate for weak areas. ,
Performing OKC testing allows the clinician to have significant clinical control. The clinician can control ROM, speed, translational stress (by shin pad placement), varus and valgus force, and rotational force. When CKC exercises are begun, control of these variables decreases, thereby increasing the potential risk for injury to the athlete.
Although most athletes do not sit flexing and extending their knees in an OKC pattern when they are performing, numerous studies (based on a variety of functional assessment tests) demonstrate a correlation between OKC testing and CKC functional performance. ,
When an athlete has an injury or dysfunction related to pain, reflex inhibition, decreased ROM, or weakness, abnormal movement patterns often result and create abnormal motor learning. Isolated OKC training can work within the limitations to normalize the motor pattern.
The efficacy of rehabilitation with OKC exercises has been demonstrated in numerous articles throughout the literature. The reader is encouraged to check the references for a more detailed description of the studies.
CKC, Closed kinetic chain; OKC, open kinetic chain; ROM, range of motion.
The primary purpose for performing OKC isokinetic assessment is the need to test specific muscle groups of a pathologic joint in isolation. Although the muscles do not work in an isolated fashion, a deficit, or “weak link,” in a kinetic chain will never be identified unless specific isolated OKC isokinetic testing is performed. Furthermore, on serial retesting, one will not know how the athlete is progressing and whether and when the athlete meets the parameters for discharge. Examples of the importance of performing isolated testing of the kinetic chain to identify specific dysfunctions have been offered by several authors, including Nicholas et al and Gleim et al.
Nicholas et al performed total leg strength isokinetic testing and developed a composite lower extremity score. They evaluated several groups of athletes with various pathologic conditions and determined that certain characteristic patterns of muscle weakness could be correlated with specific pathologic syndromes. Athletes with ankle and foot problems, knee ligamentous instability, intraarticular defects, and patellofemoral dysfunction had an irrefutable deficit in total leg strength ( P < .01). For example, athletes with ankle and foot problems have statistically significant weakness of the ipsilateral hip abductors and adductors. Furthermore, there was a trend toward ipsilateral weakness of the quadriceps and hamstring muscles, although this trend was not statistically significant.
Gleim et al also determined that the total percent deficit in the injured leg was the one value that was most informative. Typically, when a single muscle group is compared bilaterally, values that fall within 10% are empirically determined to be normal. Because the total leg strength composite score is more sensitive and minimizes variability, Gleim et al suggested that even a 5% difference in bilateral comparison is significant.
It is important to note that the only way to document weakness in muscle groups distant to the site of injury is through performance of isolated OKC testing. Furthermore, specific muscle weakness at the site of injury can be identified only by isolated OKC testing.
Another example of the need for isolated testing was illustrated in work by Davies, who performed CKC computerized isokinetic tests on athletes with various knee injuries and also analyzed bilateral comparison data. Dynamic CKC isokinetic testing, which required a linear motion with force production being measured in pounds at slow (10 in/sec), medium (20 in/sec), and fast (30 in/sec) velocities, was done on a Linea computerized CKC isokinetic dynamometer system. *
* Available from Loredan Biomedical, West Sacramento, CA.
The same athletes were also tested on a Cybex OKC computerized isokinetic dynamometer, †† Available from Cybex, Medway, MA.
and bilateral analysis of the data was performed. Isolated joint testing was performed to provide rotational force and torque values, which were recorded at slow (60°/sec), medium (180°/sec), and fast (300°/sec) angular velocities. The results of the testing demonstrated that more significant deficits were shown to exist in athletes after OKC isolated joint and muscle testing than after CKC multiple joint and muscle testing ( Table 25-1 ).Cybex (OKC) | Linea (CKC) | ||||
---|---|---|---|---|---|
Parameter | Values | Deficit | Parameter | Values | Deficit |
Peak torque force | 60°/sec (quadriceps) | 29% | Peak torque force | 10 in/sec | 9% |
U | 142 ft-lb | – | U | 462 lb | – |
I | 101 ft-lb | – | I | 420 lb | – |
Peak torque (BW) force | 60°/sec (quadriceps) | 31% | Peak torque (% BW) force | 10 in/sec | 11% |
U | 95% | – | U | 298 lb | – |
I | 66% | – | I | 266 lb | – |
Peak torque force | 180°/sec | 21% | Peak torque force | 20 in/sec | 11% |
U | 99 ft-lb | – | U | 374 lb | – |
I | 78 ft-lb | – | I | 331 lb | – |
Peak torque (BW) force | 180°/sec | 25% | Peak torque (% BW) | 20 in/sec | 11% |
U | 64% | – | U | 239 lb | – |
I | 48% | – | I | 253 lb | – |
Peak torque force | 300°/sec | 20% | Peak torque force | 30 in/sec | 16% |
U | 80 ft-lb | – | U | 302 lb | – |
I | 64 ft-lb | – | I | 253 lb | – |
Peak torque (BW) force | 300°/sec | 20% | Peak torque (% BW) | 30 in/sec | 11% |
U | 51% | – | U | 193 lb | – |
I | 41% | – | I | 171 lb | – |
Similar results were reported by Feiring and Ellenbecker with isokinetic open and closed chain testing of 23 athletes 15 weeks after ACL reconstruction. Bilateral comparisons of OKC isokinetic knee extension muscle function ranged from 74% to 77% of the uninjured extremity, whereas the results of CKC isokinetic testing using a leg press extension-type movement pattern ranged between 91% and 93% of the uninjured extremity.
When testing multiple muscle groups and developing a composite score of their force, one sees that the proximal and distal muscles apparently compensate for the weak muscles and tend to demonstrate less of a deficit than actually exists in the area. We have made this empirically based observation for years, but now CKC isokinetic testing that objectively documents and quantifies performance has supported this observation. Again, if a muscle’s performance is not measured, a deficit cannot be identified. These research studies and examples provide justification of the need for OKC isokinetic testing.
Another major reason for performing OKC isokinetic testing is the clinical control that it provides. When testing, the clinician controls ROM, speed, translational stress (by shin pad placement), varus and valgus stress, and rotational force. However, when one begins CKC testing, control of these variables decreases, thereby increasing potential risk to the athlete.
An often-cited example is that performance of OKC isokinetic tests on an athlete who has undergone ACL reconstruction can stretch or injure the graft. This is a situation of good science being applied to an inappropriate clinical setting. If the graft were actually to be stretched during OKC testing, the problem is more one of the clinician performing an inappropriate test or testing at an inappropriate time rather than the OKC test itself. Box 25-5 lists guidelines that should be followed when one is testing or rehabilitating an athlete after ACL reconstruction.
Know the type of surgery (e.g., whether an autograft or allograft was used).
Know the fixation technique.
Determine the graft status (with KT1000 testing).
Establish testing guidelines for particular pathologic conditions (exceptions always exist).
Respect soft tissue healing times (based on clinical protocols).
Use a proximally placed pad. ,
Limit range of motion (avoid 30° to 0° of knee flexion to extension).
Use faster velocities.
Correlation of Open Kinetic Chain with Closed Kinetic Chain Functional Performance
In addition to obtaining clinical control, another reason to perform OKC isokinetic testing is because of its correlation with CKC functional performance. Although athletes do not regularly function by sitting in a chair and flexing and extending their knees and even though some research indicates that there is no functional correlation, , numerous studies do demonstrate a positive correlation between OKC testing and functional performance. , , , ,
Patel et al tested 44 normal subjects and 44 subjects with unilateral ACL deficiency isokinetically to assess knee extension and flexion strength. The group with ACL deficiency had significantly less isokinetic quadriceps strength than the control group did, and this difference in strength was related to a significant decrease in the peak external quadriceps moment during jogging, jog-stop, and jog-cut activities, as well as during stair ascent. Isokinetic quadriceps strength was significantly correlated with the external quadriceps moment for these functional activities in both the ACL-deficient and control groups. This study supports the use of isokinetic muscle testing because of the correlation with basic lower extremity functional measures.
Petschnig et al demonstrated the relationship between isokinetic strength testing and several lower extremity functional tests. A limb symmetry index of 95% or higher was regularly demonstrated in normal subjects and patients after ACL reconstruction via isokinetic testing, hop tests for distance, and one-legged vertical jump tests. Additionally, Jones et al compared isokinetic dynamometry at 60°/sec with functional field tests (seated unilateral leg press, horizontal hop, single-leg vertical, and drop jumps). No significant relationships were identified between the isokinetic variables and the field tests. However, it has previously been demonstrated by Wilk et al that testing at slower speeds does not correlate with functional tests whereas faster speeds (> 180°/sec) do in fact correlate with functional hop tests. Moreover, because of the specificity of the angular velocities involved in functional activities, it relates empirically to faster isokinetic testing velocities. Admittedly, isolated joint testing is performed at velocities slower than functional velocities, but most functional movements are really a summation of velocities through the kinematic chain. Therefore, if each link in the kinematic chain were evaluated independently, the velocities would be much slower than the summated force of the entire kinematic chain—hence the reason to perform faster isolated joint testing.
Sbriccoli et al investigated the neuromuscular response of the knee extensor and flexor muscles in elite and amateur karateka. Elite karateka had higher lower extremity isokinetic torques than amateurs did. Elite karateka demonstrated a typical neuromuscular activation strategy that seems to be dependent on task and skill level. Furthermore, the results in elite karateka suggested an improved ability to recruit fast motor units as a part of training-induced neuromuscular adaptations.
Specific applications of isokinetic testing in lower extremity rehabilitation
A plethora of research exists that provides both the rationale and objective guidance for the use of isokinetics in the rehabilitation of individuals with specific lower extremity conditions, including ACL reconstruction, patellofemoral pain, hip injury, and knee osteoarthritis (OA). A summary of pertinent research in these areas will provide additional framework for the application of isokinetic testing and training in these patient populations.
Use of Isokinetics to Assist in Prognosis Following Anterior Cruciate Ligament Reconstruction and Injury
Karanikas et al investigated the adaptations in walking, running, and muscle strength after ACL reconstruction and examined the interactions between muscle strength and walking and running kinematics. Isokinetics was used for dynamic muscle assessment, and the results demonstrated that adaptation of the motor tasks and muscle strength follows different time patterns. They showed that patients can function normally at submaximal levels; however, with a decrease in muscle strength after ACL reconstruction, documented isokinetically as significant weakness that exceeds a certain threshold in comparison to the uninvolved side, the kinematics of these patients’ locomotion strategies was abnormal.
Oiestad et al identified risk factors for knee OA 10 to 15 years after ACL reconstruction. Individuals with low self-reported knee function 2 years postoperatively and loss of quadriceps strength as measured with isokinetics between the 2-year and the 10- to 15-year follow-up had significantly higher odds for symptomatic, radiographically detected knee OA. However, quadriceps muscle weakness, by itself, after ACL reconstruction was not significantly associated with knee OA.
Keays et al used isokinetic testing to evaluate 10 factors involved in the development of OA after ACL reconstruction. The incidence of OA after ACL reconstruction is disturbingly high, with reports of mild to moderate OA developing in nearly 50% of patients 6 years after surgery. The five factors found to be predictive of tibiofemoral OA were meniscectomy, chondral damage, patellar tendon grafting, weak quadriceps, and low quadriceps-to-hamstring strength ratios. The quadriceps deficits and unilateral quadriceps-to-hamstring ratios were evaluated by isokinetic testing. Use of hamstring/gracilis grafts and restoration of quadriceps/hamstring strength balance were associated with less OA of the knee.
Segal et al used isokinetics to evaluate the effect of quadriceps strength and proprioception on risk for knee OA. The finding that quadriceps strength protected against incident symptomatic but not radiographic knee OA regardless of joint position sense (JPS) suggests that strength may be more important than JPS in mediating risk for knee OA. The clinical implications of these findings are interesting because quadriceps strength is often influenced by rehabilitation interventions. Because of the accommodating resistance afforded by isokinetics, it provides an excellent intervention technique that can be used in patients with OA of the knee.
Hiemstra et al used isokinetic testing to demonstrate specific muscle imbalances in a group after ACL reconstruction versus a control group. Angle- and velocity-matched hamstring-quadriceps ratio maps demonstrated systematic variation based on joint angle, velocity, and contraction type for both the control and ACL-reconstructed groups.
Ageberg et al used isokinetics as an outcome measure to determine muscle strength in patients with ACL injuries treated by training only or by ACL reconstruction. No differences were observed between the surgical and nonsurgical treatment groups in muscle strength or functional performance. The lack of difference in patients treated by training and surgical reconstruction or by training only indicates that reconstructive surgery is not a prerequisite for restoring muscle function.
Sekir et al investigated an early versus late start of isokinetic hamstring-strengthening exercise after ACL reconstruction with a patellar tendon graft. The results of this study demonstrated that hamstring and quadriceps strength can be increased by early hamstring strengthening after ACL reconstruction with no negative impact on knee function.
Stefanska et al demonstrated that 13 weeks following ACL reconstruction, patients had significant deficits in peak torque, maximum work, and average power in the injured limb. The deficit exceeded 30% for all measured values on isokinetic testing.
Eitzen et al used a variety of outcome measures, including isokinetics, to assess whether an early 5-week exercise therapy program following ACL injury improves function. A progressive 5-week exercise therapy program led to significant improvement in knee function from before to after the program in patients classified as both potential copers and noncopers. The authors concluded that short-term progressive exercise therapy programs are well tolerated and should be incorporated in early-stage ACL rehabilitation, either to improve knee function before ACL reconstruction or as a first step in further nonoperative management.
Isokinetic Testing Related to Patients With Osteoarthritis of the Knee
One of the most important reasons for locomotor dysfunction and disability in patients with knee OA is muscle weakness in the lower extremity. Isokinetics can be used in the treatment and assessment of functional outcomes in these patients very effectively. Important references in this section provide guidance and rationale for the application of isokinetic testing and training in patients with knee OA.
Diracoglu et al performed bilateral isokinetic testing for knee flexion/extension. Although manual muscle testing (MMT) produced normal or nearly normal results, significantly lower strength values were found in patients with knee OA with isokinetic testing than in healthy subjects. Muscle strength loss cannot be detected during clinical examination but can be identified during isokinetic measurements. This again demonstrates the accuracy of dynamic isokinetic muscle testing versus static muscle testing as an indicator of muscle performance.
Segal et al used isokinetics to evaluate the effect of quadriceps strength and proprioception on risk for knee OA. The finding that quadriceps strength protected against incident symptomatic but not radiographically detected knee OA, regardless of JPS, suggests that strength may be more important than JPS in mediating risk for knee OA. The clinical implications of these findings are interesting because the strength of the quadriceps is often influenced by rehabilitation interventions. Because of the accommodating resistance afforded by isokinetics, it provides an excellent intervention technique that can be used in patients with OA of the knee.
Segal et al also performed isokinetic testing of the quadriceps and hamstrings to examine the relationship between quadriceps strength and worsening of knee joint space narrowing over a 30-month period. It was demonstrated that women with the lowest quadriceps strength had increased risk for whole knee joint space narrowing. However, no associations were found between strength and joint space narrowing in men. Consequently, quadriceps weakness was associated with increased risk for tibiofemoral and whole knee joint space narrowing.
Kean et al examined test-retest reliability and quantified the minimal detectable change (MDC) in quadriceps strength (using isokinetics and isometrics) and voluntary activation in patients with knee OA. Intraclass correlation coefficients (ICCs) for all measures ranged from 0.93 to 0.98. Based on the standard error of measurement for the isokinetic tests, the MDC was 33.90 Nm for quadriceps strength. Therefore, based on maximal quadriceps isokinetic strength testing, the results demonstrated excellent test-retest reliability in patients with knee OA. The findings suggest that these measures are appropriate for use when evaluating change in neuromuscular function of the quadriceps in individual patients.
Rydevik et al compared functioning and disability in patients with hip OA. The patients with hip OA had mild to moderate pain and significantly lower knee extension strength based on isokinetic testing. Their conclusions recommended including quadriceps strengthening and hip ROM exercises when developing rehabilitation programs for patients with hip OA in the aim of improving function and reducing disability.
Isokinetic Testing of the Hip
Because of the positioning, stabilization required, muscle mass, free limb acceleration, and impact artifact caused by the size of the muscle mass, hip testing performed with isokinetics is limited. Julia et al demonstrated very good ICCs (values between 0.75 and 0.96) for concentric and eccentric peak torque values of the hip flexors and extensors. Additionally, they demonstrated no differences between the dominant and nondominant sides of the body, which enables use of the contralateral limb as a reference.
Boling et al compared the concentric and eccentric torque of the hip musculature in individuals with and without patellofemoral pain syndrome (PFPS). Patients with PFPS displayed weakness in eccentric hip abduction and hip external rotation (ER), which may allow increased hip adduction and internal rotation (IR) during functional movements.
With an increase in the past decade in both early recognition and diagnosis of hip injuries, such as acetabular labral tears and femoroacetabular impingement, it is expected that outcomes research profiling hip strength following important new procedures to treat these injuries in elite athletes and young active patients will result in greater application of both isokinetic testing and training of the hip. Further research and publication of normative data in this region will allow greater application of isokinetics to this important joint.
Specific application of isokinetic assessment in the upper extremity
Application of isokinetic exercise and testing for the upper extremity is imperative because of the demanding muscular work required in sport-specific activities. The large unrestricted ROM of the glenohumeral joint and its limited inherent bony stability necessitate dynamic muscular stabilization to ensure normal joint arthrokinematics. Objective information on the intricate balance of agonist and antagonist muscular strength at the glenohumeral joint is a vital resource for rehabilitation and preventive evaluation of the shoulder. Therapeutic exercise and isolated joint testing for the entire upper extremity kinetic chain, including the scapulothoracic joint, are indicated for overuse injury or postoperative rehabilitation of an isolated injury of the shoulder or elbow.
Rationale for the Use of Isokinetics in Assessing Upper Extremity Strength
Unlike the lower extremities, in which most functional and sport-specific movements occur in a CKC environment, the upper extremities function almost exclusively in an OKC format. The throwing motion, tennis serve, and ground stroke are all examples of OKC activities for the upper extremity. OKC muscular strength assessment methodology allows the isolation of particular muscle groups, as opposed to CKC methods, which use multiple joint axes, planes, and joint and muscle segments. Traditional isokinetic upper extremity test patterns are open chain with respect to the shoulder, elbow, and wrist. The velocity spectrum (1°/sec to approximately 1000°/sec) currently available on commercial isokinetic dynamometers provides specificity for testing the upper extremity by allowing the clinician to assess muscular strength at faster, more functional speeds. Table 25-2 lists the angular velocities of sport-specific upper extremity movements.
Joint | Movement | Sports Activity | Angular Velocity (°/sec) | Source |
---|---|---|---|---|
Shoulder | Internal rotation | Baseball pitching | 7000 | Dillman et al |
Shoulder | Internal rotation | Tennis serve | 1000-1500 | Shapiro and Steine |
Shoulder | Internal rotation | Tennis serve | 2300 | Dillman et al |
Elbow | Extension | Baseball pitching | 2500 | Dillman et al |
Elbow | Extension | Tennis serve | 1700 | Dillman |
Wrist | Flexion | Tennis serve | 315 | Vangheluwe and Hebbelinck |
The dynamic nature of upper extremity movements is a critical factor in directing the clinician to the optimal testing methodology for the upper extremity. MMT provides a static alternative for assessment of muscular strength that uses well-developed patient positions and stabilization. , Despite detailed descriptions of manual assessment techniques, the reliability of MMT is compromised because of differences in the size and strength of clinicians and patients and the subjective nature of the grading system.
Ellenbecker compared isokinetic testing of the shoulder internal and external rotators with MMT in 54 subjects who exhibited symmetric normal grade (5/5) strength by manual assessment. With isokinetic testing, 13% to 15% bilateral differences in ER and 15% to 28% bilateral differences in IR were found. Of particular significance was the large variability in the size of this mean difference between extremities despite the presence of bilateral symmetry on MMT. The use of MMT is an integral part of a musculoskeletal evaluation. MMT provides a time-efficient, gross screening of the strength of multiple muscles by using a static, isometric muscular contraction, particularly in patients with neuromuscular disease or in athletes with large deficits in muscular strength. , The limitations of MMT appear to be most evident in instances in which only minor impairment of strength is present, as well as in the identification of subtle isolated deficits in strength. Differentiation of agonist and antagonist muscular strength balance is also complicated when manual techniques are used rather than an isokinetic apparatus.
Glenohumeral Joint Testing
Dynamic assessment of the strength of the rotator cuff musculature is of primary importance in rehabilitation and preventive screening of the glenohumeral joint. The rotator cuff forms an integral component of the force couple in the shoulder, as described by Inman et al. The approximating role of the supraspinatus muscle for the glenohumeral joint, as well as the inferior (caudal) glide component action provided by the infraspinatus, teres minor, and subscapularis muscles, must stabilize the humeral head within the glenoid cavity against the superiorly directed force exerted by the deltoid muscle with humeral elevation. Muscular imbalances, primarily in the posterior rotator cuff, have been objectively documented in athletes with glenohumeral joint instability and impingement.
Shoulder Internal Rotation and External Rotation Strength Testing
Initial testing and training using isokinetics for rehabilitation of the shoulder typically involve the modified base position. The modified base position is obtained by tilting the dynamometer approximately 30° from the horizontal base position. , This causes the shoulder to be placed in approximately 30° of abduction ( Fig. 25-1 ). The modified base position places the shoulder in the scapular plane 30° anterior to the coronal plane. The scapular plane is characterized by enhanced bony congruity and a neutral glenohumeral position that results in a midrange position for the capsular ligaments and scapulohumeral musculature. This position does not place the suprahumeral structures in an impingement situation and is well tolerated by athletes.

Isokinetic testing using the modified base position requires consistent testing of the athlete on the dynamometer. Studies have demonstrated significant differences in IR and ER strength with varying degrees of abduction, flexion, and horizontal abduction and adduction of the glenohumeral joint. The modified base position requires the athlete to be standing, which compromises both isolation and test-retest reliability. Despite these limitations, valuable data can be obtained early in the rehabilitative process with this neutral, modified base position. , ,
Isokinetic assessment of IR and ER strength is also done with 90° of glenohumeral joint abduction. Specific advantages of this test position are greater stabilization in either a seated or supine test position on most dynamometers and placement of the shoulder in an abduction angle corresponding to the overhead throwing position used in sports activities. , As a precursor to using the 90° abducted position, we require initial tolerance of the athlete to the modified base position; 90° abducted isokinetic testing can be performed in either the coronal or the scapular plane. The benefits of use of the scapular plane are similar to those discussed for the modified position and include protection of the anterior capsular glenohumeral ligaments and theoretic enhancement of the length-tension relationship of the posterior rotator cuff. , , Changes in the length-tension relationship and in the line of action of the scapulohumeral and axiohumeral musculature are reported with 90° of glenohumeral joint abduction instead of a more neutral adducted glenohumeral joint position. The 90° abducted position for isokinetic strength assessment is more specific for assessing the muscular functions required for overhead activities.
Heavy emphasis is placed on assessing the IR and ER strength of the shoulder during rehabilitation. The rationale for this apparently narrow focus is provided by an isokinetic training study by Quincy et al. Six weeks of isokinetic training of the internal and external rotators produced statistically significant improvements not only in IR and ER strength but also in flexion-extension and abduction-adduction strength. Isokinetic training for flexion-extension and for abduction-adduction produced improvements only in the position of training. The physiologic overflow of strength caused by training the internal and external rotators provides a rationale for the heavy emphasis on strength development and assessment in rehabilitation. Additional research has identified the IR and ER movement pattern as the preferred testing pattern in athletes with rotator cuff tendinopathy.
Interpretation of Shoulder Internal and External Rotation Testing
Bilateral differences
As with isokinetic testing of the lower extremities, assessment of the strength of an extremity relative to the contralateral side forms the basis for standard data interpretation. This practice is more complicated in the upper extremities because of limb dominance, particularly in athletes in unilaterally dominant sports. In addition to the complexity caused by limb dominance, isokinetic descriptive studies demonstrate disparities in the degree of limb dominance, as well as in strength dominance, only in specified muscle groups. ,
In general, maximum limb dominance of the internal and external rotators of 5% to 10% is assumed in nonathletic persons and athletes engaging in recreational upper extremity sports. Significantly greater IR strength has been identified in the dominant arm in professional, , collegiate, and high school baseball players, as well as in elite junior and adult tennis players. No difference between extremities has been demonstrated in concentric ER in professional , and collegiate baseball pitchers or in elite junior , and adult tennis players. This selective strength development in the internal rotators produces significant changes in agonist-antagonist muscular balance. Identification of such selectivity with isokinetic testing has implications for rehabilitation and prevention of injuries.
Use of normative data
Normative or descriptive data can assist clinicians in further analyzing isokinetic test data. Care must be taken to use normative data that are both population and apparatus specific. Tables 25-3 to 25-5 present data using two dynamometer systems from large samples of specific athletic populations. Data are presented with body weight used as the normalizing factor.
Speed | Internal Rotation | External Rotation | ||
---|---|---|---|---|
Dominant Arm | Nondominant Arm | Dominant Arm | Nondominant Arm | |
180°/sec | 27% | 17% | 18% | 19% |
300°/sec | 25% | 24% | 15% | 15% |
Speed | Internal Rotation | External Rotation | ||
---|---|---|---|---|
Dominant Arm | Nondominant Arm | Dominant Arm | Nondominant Arm | |
210°/sec | ||||
Torque | 21% | 19% | 13% | 14% |
Work | 41% | 38% | 25% | 25% |
300°/sec | ||||
Torque | 20% | 18% | 13% | 13% |
Work | 37% | 33% | 23% | 23% |
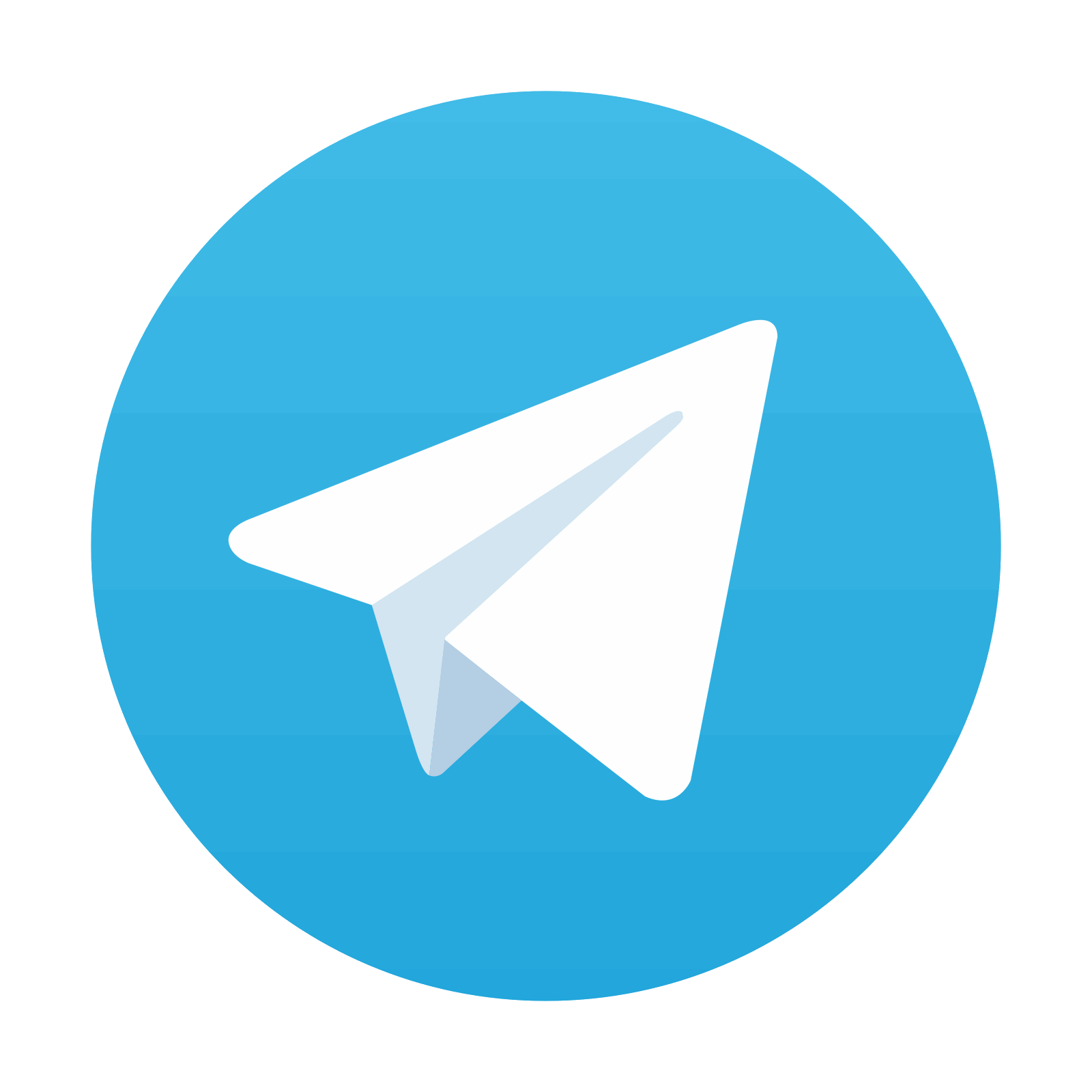
Stay updated, free articles. Join our Telegram channel

Full access? Get Clinical Tree
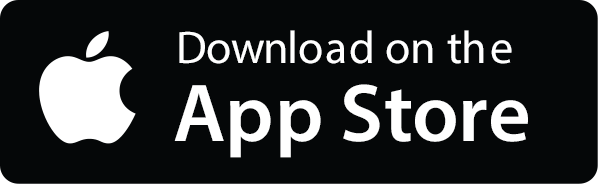
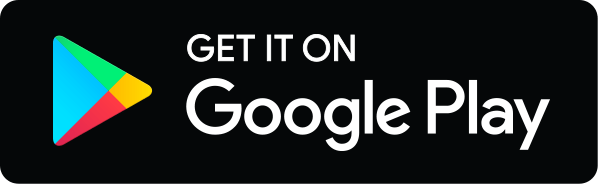
