Fig. 5.1
(a) Illustration of anatomy of meniscus orientation on the tibial plateau in the human left knee. (Reproduced from Makris et al. The knee meniscus: Structure-function, pathophysiology, current repair techniques, and prospects for regeneration. Biomaterials. 2011; 32: 7411). (b) Different aspects of the knees of seven different species. Column A shows the anterior aspect of the knees with the medial side being on the left and the lateral side on the right. Noticeable is the small band attaching the intermeniscal ligaments to the tibial plateau in the dog knee (6A). The anterior attachment of the lateral meniscus courses between the anteromedial and posterolateral bundles of the ACL in the cow, sheep, and pig knees (2A, 3A, 5A). Column B represents the posterior aspects of the knees. In all knees the medial meniscus passes behind the PCL. In the human knees, the posterior meniscofemoral ligament inserts more inferiorly on the medial femoral condyle (1B). Note the posterior thickening of the menisco-tibial coronary ligament between the lateral meniscus and tibial plateau in the sheep, goat, dog, and rabbit knees (3B, 4B, 6B, 7B). Column C shows the different tibial attachments of the knees. Notice the splitting of the tibial ACL insertion by the anterior lateral meniscus in the cow, sheep, and pig knees (2C, 3C, 5C). The lateral meniscus attachments are located central to the medial meniscus attachments in the human knee (1C). Column D shows the morphology of the menisci with the medial meniscus on the left, the lateral meniscus on the right and the anterior horns facing down. In the human knee (1D) the posterior horn of the lateral meniscus attaches anteriorly to that of the medial meniscus, a feature not seen in any of the six animal species examined. (ACL anterior cruciate ligament, ALM anterior lateral meniscus; AMM anterior medial meniscus, PCL posterior cruciate ligament, PLM posterior lateral meniscus, PMM posterior medial meniscus). (Reproduced from Proffen et al. A comparative anatomical study of the human knee and six animal species. Knee. 2012 Aug;19(4):493–9)
Compositional Considerations
Cellular morphology of the normal meniscus is consistent between large animal species, with ovoid or round chondrocyte-like cells in the inner part of the meniscus and fibroblastic, stellate or fusiform cells on the outer surface [14]. However, in rodents (rats and mice) and rabbits, the meniscus is more cellular than that in human [13]. Proteoglycan content in human meniscus is lower than in rabbit and sheep, but across all species is identified mainly in the inner zone, with some species (rabbits and cows) demonstrating proteoglycan additionally in the middle zone. Type I collagen expression is highest in the peripheral zone, where it is organized as circumferential fibers, and lowest or absent in the inner zone. In contrast, Type II collagen is greatest in the inner zone, and is organized as radial fibers [14]. Rabbit menisci demonstrate significantly different vascularity, collagen orientation, and glycosaminoglycan content compared with human, limiting their usefulness in this respect [15].
Several species commonly undergo meniscal calcification, including the Dunkin Hartley guinea pig [16], rat, mouse and cat [17]; other species are affected sporadically (Fig. 5.2). In the Dunkin Hartley guinea pig, articular cartilage lesions are tightly correlated and co-localized to the site of development of OA, and inhibition of meniscal ossification significantly reduces OA [16]. In cats, meniscal mineralization is identified in the anterior horn of the medial meniscus and is associated with medial compartment OA [17]. In humans, meniscal calcification has been identified in patients undergoing total knee arthroplasty [18], and calcium deposition is commonly identified in OA menisci [9], but the role of meniscal calcification in pathogenesis of OA has yet to be determined in human. While the aforementioned species undergo increased calcification than is typical for human, the potential advantages of some of these species for studying meniscal injury outweigh this potential disadvantage in many respects.
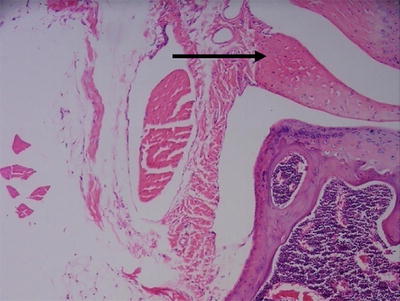
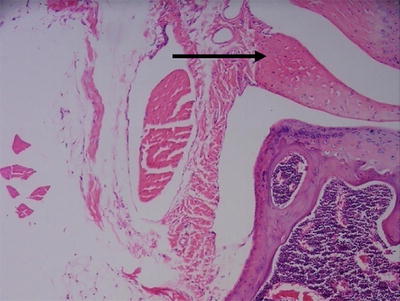
Fig. 5.2
Section of normal mouse knee joint with hematoxylin and eosin staining. The arrows points to an area of calcification within the meniscus 100×
Vascular Supply
At birth, almost the entire meniscus is vascularized, via the lateral and medial geniculate arteries, which form a perimeniscal capillary plexus with radial branches directed towards the inner zone of the menisci in human, cow, sheep, pig, dog, and rabbit [14, 19, 20]. During skeletal growth and development, radial meniscal vessels regress such that an avascular area initiates and develops from the inner zone to variable degrees in more peripheral locations. In the adult human, vascular penetration extends to only 10–30 % and 10–25 % of the meniscal width in the medial and lateral meniscus respectively [20]. This is comparable to the vascular penetration observed in sheep (6–17 % of meniscal length, compared to 9–19 % of meniscal length observed in human), but much greater than that observed in rabbit (1–3 % of meniscal length) [15]. Thus in the rabbit, the vascular region extends only 0.7–1 mm from the meniscosynovial junction, and 1.3 mm from this junction is considered to be in the avascular zone [21]. In the pig, the vascular pattern is similar to human [22], and in the dog, the peripheral 25 % of the meniscus is vascular [19]. Meniscal lesions in the vascularized zone have greater reparative ability than in the avascular zone, where lesions frequently fail to heal. Therefore, when considering the potential severity of subsequent PTA it is critical to know whether the induced lesion is in the avascular or vascular region, particularly if skeletally immature animals are used. If necessary, the vasculature should be mapped in pilot studies.
Tissues and Characteristics of Reparative Meniscal Surfaces
In humans, a small reflection of the synovium exists on both femoral and tibial surfaces of the peripheral meniscus and extends up to 3 mm from the peripheral margin. In other species, such as the rabbit, functional synovium is required to generate a robust fibrous reparative response following meniscectomy [23]. Reparative tissue is typically disorganized fibrous tissue which does not remodel to normal fibrocartilage within the time frame of any reported animal studies. This fibrous tissue may fill the gap formed after radial tear, or fill the site of the original meniscus following meniscectomy, or anterior or posterior horn resection. Fibrous reparative tissue is much less frequently encountered following longitudinal tears in the avascular zone, or following partial meniscectomy in a radial direction. Initiation of the reparative response requires extension of the injury into the vascular region, and reparative tissue generated from synovial origins attaches to the joint capsule and infrapatellar fat pad, but not the tibia. The reparative tissue is generally narrower than the original meniscus with less organization of collagen structure and inferior mechanical properties when compared to the native meniscus. An unusual response occurs in sheep following lateral meniscectomy; the popliteal tendon may dislocate into the joint, undergo structural changes and function to protect the posterior tibial plateau [24].
Mechanical Properties
Under uniaxial confined compression, the deformation of the posterior medial and lateral meniscus in human, bovine, dog, and sheep is comparable, but the permeability of the sheep meniscus is most similar to human, while the aggregate modulus of both sheep and pig are similar to human [25]. When human was compared to baboon, bovine, dog, rabbit, and pig [26], the aggregate modulus of the anterior horn of human meniscus was greater than that of the pars intermedia and posterior horn; this gradient was also seen in rabbit. Overall the aggregate and shear moduli of human medial meniscus were most similar to bovine, but the permeability of dog, rabbit, and baboon were most similar to human. The Poisson’s ratio of human, dog, bovine, and pig meniscus are similar. The pars intermedia of the medial meniscus of bovine, and pig are stiffer than human medial meniscus, whereas the stiffness of sheep meniscus was similar to human [27]. Overall, the mechanical properties of the sheep meniscus appear to more closely match those of the human than other species.
Severity of PTA
There is some species dependence with respect to the severity of OA that develops following meniscal injury. In general, the greater the degree of disruption of the meniscus, the more severe the resulting PTA, but there is some inter-species variation, and the degree of PTA may be different with lateral compared to medial injury. Compared to other models of PTA, rabbit histological lesions following meniscal injury alone are generally less severe than ACL transection models, or models in which meniscectomy is combined with ACL transection or other ligamentous injury [10]. In contrast, in the rat, medial meniscal transection may have a more rapid and severe disease course than either ACL transection or ACL transection and partial medial meniscectomy [28].
Animal Selection and Numbers
It is generally accepted that skeletally mature animals, for example rats more than 12 weeks of age [28], rabbits more than 7–8 months of age [10], and guinea pigs more than 3 months of age [29] should be used. There may be compositional differences between immature and mature animals [30], and differences in meniscal vascularity and reparative responses. For example, following medial meniscectomy in rabbits, the amount of reparative tissue formed was similar between immature and mature rabbits, but the rate of maturation was slower in older rabbits [31]. The experimental endpoint relative the rate of development of PTA is a major determinant of numbers of animals needed to detect a treatment effect; thus, pilot studies to permit adequate power analysis should be performed.
Animal Strain
With rodent species, a wide variety of genetically modified strains are commonly used and are helpful to investigate the pathogenesis of PTA. However, investigators should be aware of known differences in the propensity of “wild-type” strains to develop PTA. For example, rats commonly used in medial collateral ligament transection (MCLT) and medial meniscal tear (MMT) models are the Lewis, Sprague–Dawley, or Wistar [28]. These strains respond differently to MCLT/MMT; Lewis rats have more severe OA in the outer third of the tibial plateau with a gradient to less severe OA in middle and inner thirds, but Sprague–Dawley rats have the reverse gradient, with more severe lesions in middle third, and develop larger osteophytes. Sprague–Dawley rats also develop spontaneous cartilage cysts with age, whereas Lewis rats do not [28]. Further, Lewis rats have a defective hypothalamic–pituitary–adrenal axis, thus may have increased pain after MMT/MCLT compared to Wistar rats [32].
Destabilization of the Medial Meniscus in Rodent Injury Models
Destabilization of the Medial Meniscus (DMM)/Cranial (Anterior) or Caudal (Posterior) Meniscal Pole Release
Destabilization of medial meniscus (DMM) was recently introduced in murine OA models using the 129/SvEv strain [33]. The surgical approach is to transect the medial meniscotibial ligament to induce PTA, while leaving the lateral meniscotibial ligament, medial collateral ligament and meniscus intact (Fig. 5.3). Mice following DMM surgery demonstrated greater stress on the posterior femur and central tibia of the medial side of the joint. DMM increases anterior–posterior range of motion, and results in bone and cartilage loss primarily on the posterior portion of the medial tibial plateau [34]. DMM induces mild-to-moderate knee PTA within 4 weeks, while moderate-to-severe PTA is observed at 8 weeks. The severity and location of lesions after DMM may be similar to lesions observed in naturally occurring OA in mice [33].
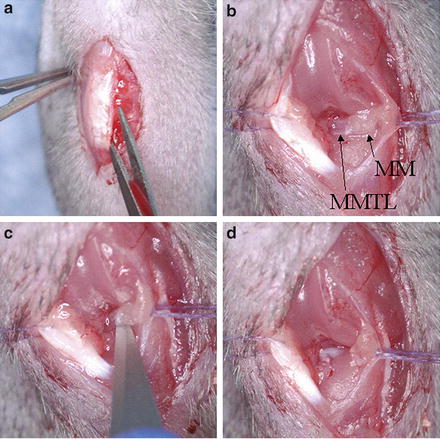
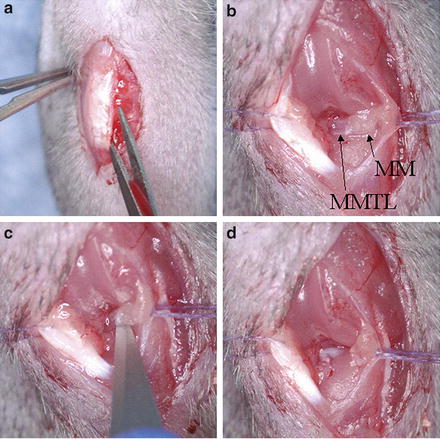
Fig. 5.3
(a) Surgical approach to the right mouse knee joint with a medial para-patellar ligament incision; (b) Following fat pad blunt dissection the medial meniscus (MM) and medial meniscotibial ligment (MMTL) are identified; (c) Transection of the MMTL; (d) Following transection, destabilization of the medial meniscus (DMM) is complete and the meniscus is free to displace medially. Note: Incisions are shown twice as long as usual in order to obtain good quality images
In rats following DMM, matrix loss occurs in the superficial and middle zones of cartilage by one week after surgery, and severe cartilage clefts are observed by four weeks [35, 36]. Similar to mice receiving DMM, rats also exhibit the most severe lesions in the central area of medial tibial plateau. However, to date, the majority of DMM data are from the mouse, possible due to the increased opportunity for use of transgenic animals.
OA patients often present with joint inflammation, with increased inflammatory immune cells infiltrating into the synovium [37]. It is important to note that DMM only induces mild synovial inflammation in mice [38], while enzymatically induced-OA model such as intra-articular injection of collagenase causes prolonged joint inflammation [39]. Similar to OA in humans, wild type mice following DMM also show subchondral bone changes and osteophyte formation [33, 36, 40]. Due to its reproducibility, the murine DMM model has been widely used in various studies investigating OA pathogenesis and therapeutic treatments for OA. In the following sections, we will discuss several important parameters in applying the DMM model to mice.
Mouse DMM PTA Model and Aging
Through microarray analysis of joint tissue, 12-month old C57BL/6 mice demonstrated increased activity of matrix genes and matrix-degrading enzymes compared to 12-week old mice after DMM [41]. Therefore, the authors suggested that age of the mice should also be taken into consideration during mouse DMM PTA experimental design. Furthermore, although It has been implied that DMM surgery induces cartilage lesions similar to those observed in spontaneous OA in mice, we are not aware of any longitudinal comparisons of DMM-induced PTA compared to naturally occurring OA in mice [33]. Therefore, the relationship between DMM-induced OA and spontaneous OA in mouse models remains unknown.
Mouse DMM PTA Model is Sex-Dependent
Similar to humans, the severity of DMM-induced PTA in mice is sex dependent. In the 129S6/SvEv strain, male mice develop more severe PTA than females after DMM, and the same trend was observed in 129SvEv, FVB/N, and C57BL/6 mice [42]. These differences may be associated with sex hormones since ovariectomized female mice had less severe PTA in comparison to intact females following DMM. In humans, however, it is important to note that women have higher prevalence of OA than men after age of 50 [43].
Mouse DMM PTA Model and Obesity
Obesity is one of the primary factors in OA development; therefore, researchers have been seeking to understand the relationships between obesity and PTA using mouse DMM or other similar surgically induced meniscus-destabilization models [44]. Increased tissue adiposity predisposes to anesthesia-related complications and increases the difficulty of visualizing the meniscotibial ligament of the medial meniscus in obese mice due to increased size of the infrapatellar fat pad. The infrapatellar fat pad is located in the anterior of the knee joint, between the joint capsule and the synovium [45], and blunt dissection over the intercondylar region through the infrapatellar fat pad must be used to visualize the meniscotibial ligament of the medial meniscus [33]. The infrapatellar fat pad consists of mainly white adipocytes but also contains immune cells and stem cells that are capable of differentiating into mesenchymal lineages [46, 47]. The infrapatellar fat pad also plays a role in patellar blood supply [48]. High-fat diet increases the size and blood vessel network of the infrapatellar fat pad [49]. Therefore, DMM surgery in obese mice is also more challenging since increased angiogenesis in the infrapatellar fat pad may increase hemorrhage at surgery. Based on our observation, epinephrine frequently needs to be applied to the fat pad for hemostasis when performing DMM in obese mice. While some researchers prefer to completely remove the infrapatellar fat pad (personal communications in animal model study session, OARSI 2013), total removal of the infrapatellar fat pad may influence disease progression. The infrapatellar fat pad may play an active role and stimulate development of PTA in human and animal models by secreting cytokines such as IL-6 [50–53], despite the fact that more clinical trials are required to elucidate the whether removal of IFP is beneficial or detrimental to development of OA in patients [54]. Therefore, care needs to be taken in interpretation of results if investigators remove the infrapatellar fat pad during DMM surgery. Here, we suggest that resection of the IFP should be restricted to the smallest amount possible to allow visualization of the medial meniscotibial ligament. Furthermore, clear description of the degree of resection of the infrapatellar fat pad in the manuscript will also help investigators compare PTA progression and severity in obese mice between different studies.
In addition, obesity accelerates DMM-induced PTA progression. The cartilage surface of obese mice after DMM exhibited deep fissures at 2 weeks (Fig. 5.4). The time needed to develop OA in obese mice is significantly shorter than in lean mice and subchondral bone erosion may occur in obese mice by 12 weeks post-surgery. Moreover, we found that metabolic factors such dietary fatty acids contribute a more critical role in DMM-induced PTA than do mechanical factors such as body weight in obese mice [55].
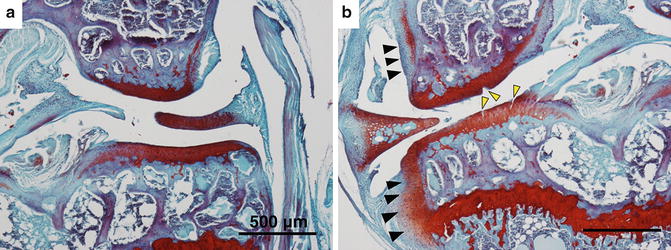
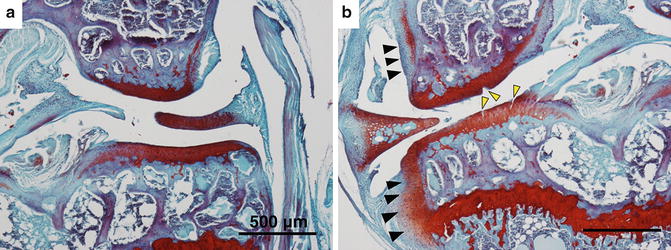
Fig. 5.4
Safranin O and fast green staining of knee joint of C57BL/6J obese mice (a) without DMM (b) with DMM surgery. The mice weighted around 35 g. Obese mice receiving DMM exhibited cleft and fissures in cartilage (yellow arrowheads) and osteophyte formation (black arrowheads) within 2 weeks post-surgery
Mouse DMM PTA Model and Biomechanical and Neurobehavioral Activity Measurements
The DMM model has been used to elucidate the relationships between OA, pain and behavioral activities. Interestingly, several studies have shown that DMM-induced OA does not significantly affect spontaneous locomotion and rota-rod performance in mice at any time point post-surgery [56], while some have reported that DMM surgery decreased the distance mice traveled per hour [57]. The discrepancy between these studies may result from the length of time mice were assessed for spontaneous activity. However, when mice receive transection of both anterior and posterior cruciate ligament, they showed decreased rota-rod performance one week post-surgery but maintained the same spontaneous locomotor activity compared to the sham group [58], which may be related to development of more severe PTA in ACL transected mice than the mice receiving DMM surgery.
The DMM model has also been used to investigate PTA-related pain. Inglis et al. [59] demonstrated that pain behaviors develop several weeks after detectable histologic change of articular cartilage post-DMM, and this delay could be associated with the inhibition by peripherally active endogenous opioid actions. It has been further reported that nerve growth factor (NGF) was highly upregulated in mice 3 days and 16 weeks post-DMM surgery but not at any interim time point. Treating mice with TrkAd5, a soluble NGF receptor, however, suppresses pain at these two time points, indicating that NGF could be a critical mediator in PTA-related pain. In addition, macrophages may be involved in OA pain resulting from DMM. Miller et al. [57] observed that macrophages massively infiltrate into dorsal root ganglia along with upregulated the monocyte chemoattractant protein-1 (MCP-1) and its receptor C-C motif receptor 2 (CCR2) at 8 weeks post-DMM surgery, correlating with the time mice start to exhibit pain behavior. Interestingly, the upregulation of MCP-1/CCR2 returned back to baseline levels at 16 weeks after DMM, while infiltrated macrophages still remained within the dorsal root ganglia [57]. The results of these studies imply that various mechanisms may regulate PTA pain at different time points following joint injury. Indeed, these mechanisms are further complicated by obesity and sex. For examples, in our previous studies, we found that C57BL/6 male obese mice preferentially affected spinal sites for hyperalgesia [55], while female C57BL/6 mice showed hyperalgesia primarily through supraspinal sites when fed a high-fat diet [60]. Thus, our findings and the results of others suggest that the effect of obesity on nociceptive responses of C57BL/6 mice may be sex dependent [61].
Mouse DMM OA Model and Cage Environment
In addition to age and obese status of the mice, mouse husbandry such as environmental enrichment devices may have a role in DMM-induced PTA. For example, Salvarrey-Strati et al. [62] reported that mice housed with heavy plastic tube (CPVC tube) and Tecniplast Mouse House exhibited higher OA score, while mice with Shepherd Shack had less cartilage degeneration. These authors also observed that the numbers of the mice housed in the cage had significant impact on OA severity as group-housed mice demonstrated higher OA score relative to individually housed mice. To establish a more reliable mouse DMM model, Kim et al. [63] suggest that using small cages (75 L × 40 W × 200 H mm3) with limited movement may decrease individual variation and control OA severity; however, animal welfare considerations of limited housing space need to be considered.
Other Meniscal Injury Models
DMM in Non-rodent Species
DMM surgery in the rabbit has recently been described [64]. Peak contact stress in the medial compartment of operated joints was significantly elevated immediately following DMM surgery. In addition, both medial femur and medial tibia had more severe OA as compared to the lateral side of the operated joint 8 weeks post-surgery. These data suggest that DMM in the rabbit model has similar distribution of cartilage PTA lesions as murine DMM models. However, in contrast to the murine model, the posterior rather than the anterior horn of the medial meniscus is destabilized by transection of the posterior root of the medial meniscus. Posterior horn medial meniscal release in dogs results in lameness, joint effusion, radiographic evidence (effusion, subchondral bone sclerosis, osteophytosis) of OA, gross and sonographic evidence of meniscal pathology, and arthroscopic and gross evidence of articular cartilage pathology, primarily on both surfaces of the medial compartment. Reparative tissue was only evident across the third of the meniscus in the vascular zone closest to the meniscocapsular border [65]. In the sheep, cranial pole release of the medial meniscus caused a temporary unloading of the limb (approximately 85 % of baseline at 2.5 weeks postoperatively), and cartilage lesions and thinning at 12 weeks predominantly in the craniomedial tibial plateau and corresponding femoral contact areas. However, while location of the focal lesions was different from medial meniscectomy and medial body transection groups, gross and microscopic OA scores were similar. The major pathological difference between medial meniscectomy, medial body transection, and cranial pole release was the degree of subchondral sclerosis in the medial femoral condyle [12]. Cake et al. also identified key molecular similarities between meniscectomy and cranial pole release, supporting the idea that maintenance of meniscal mechanical function and resistance to tensile hoop stresses is critical for prevention of cartilage pathology [12].
Medial Meniscal Tear/Medial Collateral Ligament Transection (MMT/MCLT)
In the rat, transection of the medial collateral ligament (MCL) distal to the attachment of the medial meniscus to the MCL, then transection of the medial meniscus at its narrowest point results in rapid cartilage degeneration and subchondral sclerosis by 3–6 weeks postoperatively, reduced weight-bearing by 3 weeks, and increased mechanical allodynia and modulation of motivational, reward-aversion and pain sensory circuitry in the brain at 3–5 weeks [28, 66–68]. However, increased thermal hyperalgesia or blunt pressure mechanical hyperalgesia are not features of this model [32].
PTA lesions are most severe on the outer third of the medial tibia adjacent to the synovium, and less pronounced in the middle third and inner third, adjacent to the ACL, although this is rat strain dependent [28, 67]. At 8 weeks, joint space narrowing is evident by μCT examination [69], and changes relating to chronic neuropathy and inflammatory pain are noted in dorsal root ganglia and spinal cord [69]. By 12 months, there is progression to eburnation of the medial tibial plateau [28]. Osteophytes progressively increase in size, and subchondral bone remodeling is also evident. The integrity of the MCL postoperatively is critical and influences the degree of capsular thickening observed, which alters joint stability and therefore development of OA [28]. Generally a 3-month time point is recommended to evaluate any local treatment effect [28], although longer time points may be needed for some outcome measures.
The additional effect of MMT compared to MCLT alone has been recently investigated in Lewis rats followed for 28 days postoperatively [70]. MMT/MCLT limbs had increased mechanical allodynia compared to unoperated in the same animal, but compared to control animals, there was only a trend to increased mechanical allodynia in MMT/MCLT groups, and no difference between surgical groups. High-speed videography was required to detect dynamic gait asymmetry that was identified in MMT/MCLT, but not MCLT groups. Peak vertical force and vertical impulse were reduced in MMT/MCLT, compared to control, and vertical impulse was reduced in MMT/MCLT, compared to MCLT alone. Propulsive forces were lower in MMT/MCLT animals than in controls, but this was only a trend in MCLT animals. Significant differences in serum cytokines were not identified between groups. Histological lesions were significantly worse in MMT/MCLT than MCLT [70]. Thus, MMT/MCLT produces very distinctive functional and histological deficiencies that MCLT alone does not. Together, these data demonstrate the importance of well-designed functional outcome measures, and the usefulness of MMT/MCLT in investigation of a range of joint-level to whole body measures of PTA.
Similar models in guinea pigs result in acute synovitis, loss of chondrocytes and proteoglycan, and collagen disruption in the superficial and middle zones of the tibial plateau as early as 3 days postoperatively. By 3 weeks, there is loss of one third of the medial tibial plateau cartilage and immature tibial and femoral osteophytes with synovial hyperplasia. By 12 weeks, cartilage loss extends into the deep zone of the tibial plateau and osteophytes are extensive. In contrast, in the guinea pig, lateral collateral ligament transection and meniscal tear results in inconsistent lesion development, suggesting that the degree and pattern of weight-bearing postoperatively is critical [29].
Radial Tears
In rabbits, healing ability following radial tear is similar to longitudinal lesions and is influenced primarily by the extent of the tear, and whether it incorporates the vascularized peripheral region. However, the radial margins of the tear retract, resulting in a greater volume of repair tissue in radial compared to longitudinal tear [71]. Radial tears in the pars intermedia of the rabbit heal rapidly with fibrocartilage by 7 weeks [72]. In contrast, complete radial tear of the medial meniscus in sheep between the anterior horn and pars intermedia separates and fills with disorganized fibrous tissue by 6–12 months. This lesion results in mild synovial effusion and hypertrophy, severe medial compartment OA and mild lateral compartment OA at 6 and 12 months, and a significant increase in Outerbridge grade of the retropatellar cartilage. Atypically, compared to other studies, at these extended time points, there was no difference in OA between tibial plateau and femoral condyle [73, 74].
Mid-body transection of the medial meniscus in sheep fills with reparative tissue and results in more persistent gait abnormalities, but less severe PTA than following either medial meniscectomy or cranial pole release. Medial tibial plateau lesions are centrally located, with opposing femoral lesions. Subchondral sclerosis of the medial femoral condyle also occurs [12]. It is possible that a less robust reparative response occurs in the dog and pig compared to the rabbit and sheep following radial tear. Wedge-shaped defects in the dog, with the base of the wedge in the peripheral zone, result in an incomplete fibrovascular healing response with little progression over 1-year. The resulting PTA is more prominent on the tibial plateau than on the femoral condyles [75]. In contrast, a radial incision of the medial meniscus formed a 1–2 mm gap, but by 2 weeks was filled with a fibrin clot, and 6 weeks the gap was filled with well integrated fibrovascular scar [19].
In pig, radial tears at the junction of the anterior horn and pars intermedia extending from the inner zone for two thirds of the width do not heal but development of PTA was not evaluated [22]. In general, radial tears heal with reparative tissue, but there is loss of the tensile hoop function of the meniscus which results in PTA. Healing response may be less robust in the pig and depends on minimal trauma to the synovium if a wedge-shaped defect is used.
Longitudinal Tears
The majority of longitudinal tears in the avascular zone of the pars intermedia of rabbit, dog, pig, and sheep fail to heal by 6 months, with no evidence of intrinsic repair. If the tear is extended to the meniscosynovial junction, then evidence of healing by extrinsic tissue is seen [76]. For example, peripheral lesions in the vascular region of the rabbit heal by 10 weeks and significantly better than lesions in the meniscal body and inner rim, which do not heal by 10 weeks [71, 77]. In rabbits, 3–6 mm longitudinal lesions in the avascular zone of the medial meniscus, between the pars intermedia and the anterior horn fail to heal and may lengthen by 11 months. PTA is identified by 3 months, with the most contribution to aggregate joint scores from the tibial plateau and no contribution from the lateral femur. By 9 months, OA is identified on all cartilage surfaces [78], and at 11 months, range of motion in extension may be limited by 10°–25° [79]. Likewise, in the lateral meniscus, 4 mm longitudinal lesions in the avascular zone of the pars intermedia fail to heal [80]. In the posterior horn longitudinal tears in the avascular zone only partially heal by 6–12 weeks, and the reparative tissue has tear load propagation resistance only 20–25 % of that of control tissue [81].
In large animal models of longitudinal tear, 15–20 mm lesions in the anterior horn are typical, also fail to heal and result in PTA. Longitudinal tears in the vascular region of the anterior horn of the medial meniscus in adult sheep result in a persistent hyperintense UTE T2* magnetic resonance imaging signal in the anterior horn and a hyperintense T2 MRI signal in the femoral and tibial cartilage at 4 months, even after immediate suture repair. On the tibial plateau, the T2 signal was more hyperintense anteriorly than posteriorly, indicative of greater collagen disorganization in the anterior tibial plateau [82]. Sixteen weeks after 10 mm longitudinal tears were made in the anterior horn of the medial meniscus in adult sheep, 3 mm from the meniscocapsular junction, synovial effusion, synovial hyperplasia, and failure of the tear to heal with no evidence of intrinsic repair were noted, but cartilage was not evaluated [83].
In the dog, a longitudinal tear extending between the anterior and posterior horn, then “trapped” in the intercondylar notch by suture resulted in synovial effusion at 2 weeks and synovial hypertrophy at 4 weeks which resolved by 12 weeks, but meniscal atrophy and gross evidence of tibial and femoral PTA was evident [84]. In the central avascular portion of the medial meniscus in the dog, 10 mm longitudinal incisions fail to heal by 10 weeks [19]. Longitudinal incisions in the posterior horn of the canine medial meniscus, 2–3 mm from the menisco-synovial junction partially healed by 12 weeks after surgery but osteophytes were evident at the condylar margins, OA histological scores were increased, synovitis was observed and linear and toe region tensile moduli were reduced compared to control [85]. The majority of 15 mm longitudinal tears in the peripheral 25 % of the posterior horn-pars intermedia of the goat fail to heal within 6 months, but chondral injury was also not observed [86]. Similarly, bi-meniscal 10 mm longitudinal tears in sheep in the avascular zone result in synovial effusion, decrease in synovial fluid pH and total protein, and failure to heal at 1 month [87].
Partial Medial Meniscectomy
Several approaches to partial medial meniscectomy are reported: either radial resection of one- to two-thirds the width of the meniscus [88, 89], or resection of anterior or posterior horn. Further, wide variation in the degree of resection has been reported, ranging from 50 to 80 % in the dog [90]. The reparative response generated following partial medial meniscectomy depends on the plane of resection, and on whether the injury site includes the meniscosynovial junction. The reparative tissue is generally not protective against PTA, but may mitigate the development in disease in areas of the tibial plateau formerly covered by meniscus.
In the athymic rat, resection of the medial meniscus obliquely from the inner rim to two thirds of the width at the junction between the anterior horn and the pars intermedia fails to heal [91]. In contrast, in the Lewis rat, resection of the anterior half of the medial meniscus at the level of the medial collateral ligament results in reparative tissue filling 60–80 % of the tibial plateau surface area within 4 weeks, but the reparative response does not prevent a large area of PTA on the medial tibial plateau, which is evident from 4 weeks [92]. In the rabbit, the defect following removal of the anterior half of the medial meniscus may still be evident at 12 weeks, but there is some evidence of a reparative response by 12–16 weeks. Highly reproducible osteochondral lesions and sclerosis of the subchondral bone are observed and are worse on the medial tibial plateau than on the medial femoral condyle, and joint space narrowing is seen in simulated weight-bearing radiographs [93, 94]. Resection of the pars intermedia of the medial meniscus in immature rabbits results in formation of additional longitudinal tears in the posterior horn of the operated meniscus, and the original defect remains largely unfilled [95].
Formation of robust reparative tissue is variable following radial resection of one- to two-thirds of the meniscus in the dog, in contrast to the rabbit where repair tissue is prominent [88, 89, 96, 97]. In the dog following partial radial resection, the lateral compartment is grossly normal, but the appearance of the medial compartment is variable, from normal to synovitis and synovial hyperplasia with focal articular erosion, and moderate histological damage [88], but in general there is correlation between degree of meniscus resection and tibial plateau OA [89]. In contrast to partial radial resection, resection of the anterior 50 % of the medial meniscus in the dog results in defect filling with large amounts of reparative tissue. Despite these reparative attempts, PTA is observed in both the tibial and femoral cartilages within 3 months. Medial compartment osteophytes are also observed, but lateral compartment or patellar groove osteophytes are rare [29]. Subchondral sclerosis is prominent at 3 months, but synovial pathology is mild [29]. Following 80 % subtotal medial meniscectomy, lameness increases after 180 days, and only 25 % of joints showed any reparative response of the medial meniscus [98].
Resection of the posterior horn of the medial meniscus in dogs results in obvious lameness and PTA at 12 months, with a persistent defect that is slow to fill and mature. Fibrous replacement tissue is disorganized, immature and shows poor integration even at 12 months. Osteoarthritic cartilage on the femoral condyles and tibia had reduced stiffness compared to normal [99]. Compared to longitudinal incisions in the posterior half of the medial meniscus, 2–3 mm from the meniscosynovial junction, partial posterior horn meniscectomy to within 2–3 mm of the meniscosynovial junction tends to have increased gross histological scores, but similar reductions in linear and toe region tensile moduli, compared to control, and histological scores correlate with linear region tensile moduli [85]. Ex vivo studies in the dog suggest that 30 % radial width partial posterior horn meniscectomy has no significant effect on contact mechanics, whereas 75 % radial width partial posterior horn meniscectomy and posterior horn meniscectomy increased contact pressures [100]. Though no direct comparison has been made, persistent lameness and reduced reparative response appear to be features of posterior horn compared to anterior horn resection in the dog. In general, compared to lateral meniscectomy models in rodent and rabbit, pathology develops more slowly in partial medial meniscectomy models in the dog [29]. In the sheep, resection of the anterior horn of the medial meniscus leads to filling of approximately 25 % of the defect with fibrous tissue, and evidence of PTA, particularly on the tibial plateau within 6 weeks of surgery [101].
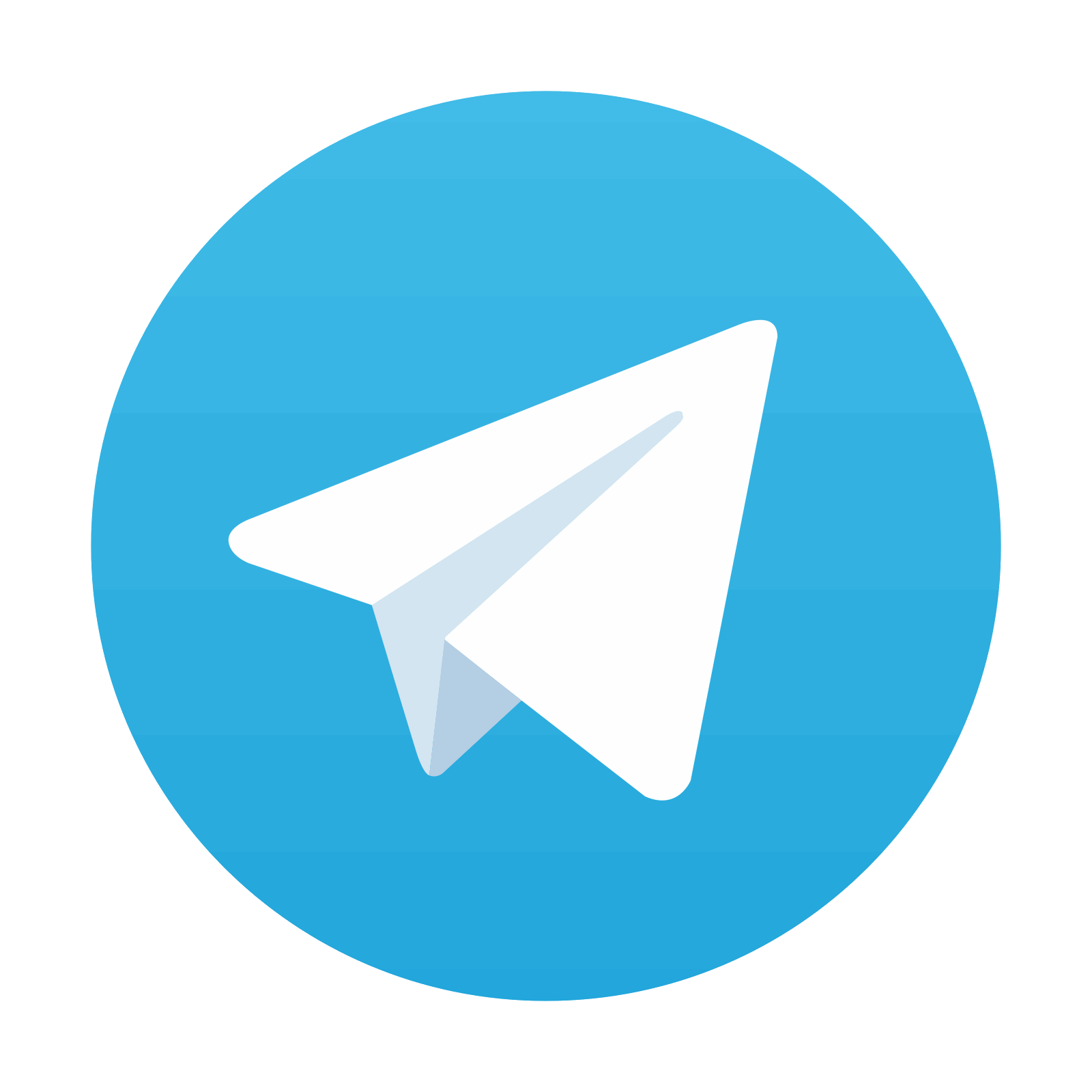
Stay updated, free articles. Join our Telegram channel

Full access? Get Clinical Tree
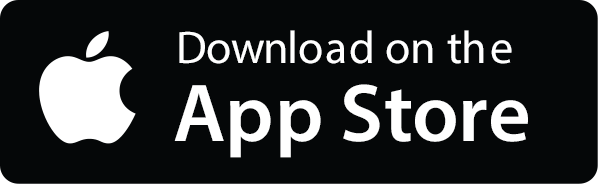
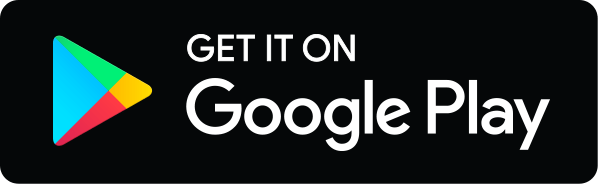