Fig. 1.1
Illustration showing the static and dynamic constraints to instability. The three primary static constraints to elbow instability are the ulnohumeral articulation, the anterior medial collateral ligament (AMCL) , and the lateral collateral ligament, especially the ulnar part of the lateral collateral ligament, which is also referred to as the lateral ulnar collateral ligament (LUCL) . The secondary constraints include the radial head, the common flexor and extensor tendon origins, and the capsule. Dynamic stabilizers include the muscles that cross the elbow joint, F-P (flexor-pronator), and produce compressive forces at the articulation. Used with permission of Mayo Foundation for Medical and Educational Research. All rights reserved [47]
Primary Stabilizers
Ulnohumeral Joint
The highly congruent bony architecture of the ulnohumeral joint makes it an inherently stable articulation, particularly at the extremes of flexion and extension. The distal humerus is composed of two columns that flare out from the diaphysis and include the medial and lateral condyles and epicondyles, which serve as important sites of origin for other stabilizing structures of the elbow (See Fig. 1.2). Positioned between the two columns are the hemispherical capitellum on the lateral side and the adjacent trochlea on the medial side, its name derived from a Latin word meaning “pulley.” The trochlea is covered by cartilage over an arc of 300–330° and articulates with the semilunar notch of the ulna [1, 2]. The medial contour of the trochlea is more prominent and projects more distally than the lateral portion. The center of rotation of the trochlea lies collinear with the anterior cortex of the humerus, rotated about 30° anteriorly with respect to the long axis of the humerus . In the transverse plane, the axis of rotation is rotated internally approximately 5° and in the frontal plane it is in approximately 6° of valgus.


Fig. 1.2
Anatomic features of the distal humerus. This figure republished with permission from The Elbow and Its Disorders, 4th Ed, Morrey BF, 2009, Copyright Elsevier [3]
The proximal aspect of the ulna contains the semilunar notch (also known as the greater sigmoid notch or incisura semilunaris ), the guiding ridge, and the coronoid process [3]. The trochlear groove on the humerus mates with the guiding ridge of the semilunar notch with a high degree of congruity. To match the anterior position of the trochlea on the humerus, the semilunar notch on the proximal ulna has a posterior inclination of about 30° that promotes bony stability of the elbow in full extension [1]. The ulnohumeral articulation ultimately has its greatest stability at terminal extension and flexion, due to the high degree of conformity between articular surfaces. In extension, the olecranon articulates with the conforming olecranon fossa, while in flexion, the coronoid articulates with the coronoid fossa and the radial head articulates with the radial fossa. In a study designed to remove sequential sections of the ulna while testing the stability of the articulation in extension and at 90° of flexion, it was determined that valgus stress is primarily resisted by the proximal half of the sigmoid notch, whereas varus stress is resisted primarily by the coronoid portion of the articulation [4].
In normal circumstances the range of motion through the ulnohumeral joint is from 0°, or slightly hyperextended, to 150° of flexion [3]. There have been several published analyses of the hinge-like motion of the elbow demonstrating that its rotation occurs through the center of the arcs formed by the trochlear sulcus and capitellum [5, 6]. In several three-dimensional studies of elbow motion, it has been revealed that the elbow has a “helical” type motion with a varying axis of rotation [5, 7]. For practical purposes, the ulnohumeral joint can be assumed to move as a uniaxial articulation.
The coronoid process of the ulna plays a significant role in ulnohumeral stability, and the understanding of its stabilizing role continues to evolve through several recent investigations. Three structures insert on the coronoid: the anterior bundle of the MCL , anterior joint capsule , and the brachialis [8]. The “critical portion” of the coronoid needed for stability has been debated and information on the amount of the coronoid required for stability with or without ligamentous integrity and with and without the radial head is now emerging [9]. As sections of the coronoid are sequentially removed, the elbow becomes progressively unstable. An investigation by Hull et al. has shown that significant varus instability exists after 50 % resection of the coronoid process, particularly in lower flexion angles [10]. Recent attention has been given to the anteromedial facet of the coronoid. O’Driscoll et al. has proposed a new variation to the classic fracture classification of Regan and Morrey attributing more significance to anteromedial facet involvement owing to the facet’s significant stabilizing function [11]. Anteromedial facet fractures can involve injury to the anterior bundle of the medial collateral ligament if they involve the sublime tubercle, and are typically associated with disruptions of the lateral ulnar collateral ligament and posterior band of the MCL , leading to varus and posteromedial rotational instability. The importance of the anteromedial facet with regards to varus stability was confirmed in a biomechanical study by Pollock et al. [12].
Medial Ligaments
The medial collateral ligament (MCL) is a complex of ligamentous structures that together serve as primary stabilizers to valgus and internal rotatory stresses on the joint [13] (See Fig. 1.3). The MCL is composed of three parts: an anterior bundle (anterior oblique), posterior bundle (Bardinet ligament), and a transverse segment (ligament of Cooper) [3, 13–16]. Of these three parts, the anterior bundle is felt to be the component most consistently identified in cadaveric studies while the posterior bundle and transverse bundle are occasionally absent or indistinguishable from the joint capsule [17]. The anterior bundle originates from the anteroinferior medial epicondyle and inserts immediately adjacent to the articular surface of the ulna at the sublime tubercle [1, 14, 17]. The average area of the origin has been measured as 45.5 mm2, and the average area of the insertion has been measured as 127.8 mm2. The edge of the insertion is separated from the ulna articular margin by an average of 2.8 mm [18]. Biomechanical studies have led to further subdivision of the anterior bundle into an anterior band, a posterior band, and more central isometric “guiding bundle.” [19] The posterior bundle is generally described as a fan-shaped thickening of the capsule originating from the inferior medial epicondyle, inserting along the mid-portion of the medial margin of the semilunar notch [3] and forming the floor of the cubital tunnel [14]. The transverse ligament is composed of horizontal fibers from the coronoid to the tip of the olecranon [17]. Of the aforementioned structures, the anterior bundle has been identified as the most important structure for valgus elbow stability with the posterior bundle a distant second. The transverse ligament has no measurable impact on elbow stability [14, 20].


Fig. 1.3
Medial collateral ligament complex with anterior bundle, posterior bundle, and transverse ligament. Used with permission of Mayo Foundation for Medical and Educational Research. All rights reserved [3]
The anterior bundle of the MCL does not originate precisely at the center of rotation of the ulnohumeral joint, thus the tension within the ligament varies from flexion to extension (See Fig. 1.4). Further research into this concept has lead to the description of the separate anterior and posterior bands [21]. The anterior band is under tension in extension while the posterior band is under tension in flexion [14]. The intermediate segment (or guiding bundle) is a small group of fibers that are functionally isometric sitting between anterior and posterior bands [17, 22]. Based on these observations, the anterior and posterior bands appear to have biomechanically different roles.


Fig. 1.4
Dynamic representation of the medical collateral ligament complex at different points of elbow flexion. Areas of tension highlighted by the shaded areas in the figure. Within the anterior bundle, the bands tighten in reciprocal fashion. The anterior band is taught in extension and relaxed in flexion while the posterior band is taught in flexion and relaxed in extension (represented by the shaded areas). Tension in the posterior bundle increases with elbow flexion (Modified with permission from Callaway et al. Biomechanical evaluation of the medial collateral ligament of the elbow. J Bone Joint Surg Am. 1997;79:1223–31 [14])
These three parts of the MCL have been studied in several biomechanical investigations designed to determine their relative contributions to stability. In general, these studies have utilized cadaveric specimens and materials testing instrumentation designed to apply a specific amount of force and then measuring the resultant displacement and angulation. In these models, the ligamentous structures are sequentially cut as force is applied in order to determine relative contributions to valgus stability [13, 14, 23–25]. These investigations agree that the anterior bundle of the MCL is the most important contributor to valgus stability. In an early study by Morrey et al., the MCL was shown to contribute roughly 31 % of total elbow valgus stability in extension and 55 % of the stability in 90° flexion [24]. Follow-up studies by Morrey et al. and Hotchkiss et al. showed that it acts as a primary valgus stabilizer between 20 and 120° of flexion supplying up to 78 % of valgus stability [13, 23]. In similar fashion, Callaway et al. showed that the anterior band of the anterior bundle serves as the primary restraint to valgus rotation at 30, 60, and 90° of flexion while the posterior band is the co-primary restraint at 120° flexion. The posterior bundle is a secondary restraint at 30° only [14]. An intact MCL provides essentially full stability in the absence of the radial head, which becomes significant in surgical decision-making in the setting of isolated comminuted fractures of the radial head where excision may be considered an option with intact ligaments [13]. However in the setting of elbow instability and compromised ligaments, radial head excision is not an option and repair or replacement should be performed.
Lateral Ligaments
The lateral collateral ligament complex (LCL) contributes to varus and posterolateral stability. The LCL consists of 3 main parts that have been shown to contribute in some measure to elbow stability: the lateral ulnar collateral (LUCL) , radial collateral (RCL) , and annular ligaments (see Fig. 1.5). Also included in the description of the LCL is the accessory LCL, which functions to stabilize the annular ligament during varus stress [3]. These structures have more anatomic variability and are sometimes difficult to distinguish compared to their medial counterparts [17]. The Lateral Ulnar Collateral Ligament (LUCL) serves as the primary restraint to posterolateral rotatory instability (PLRI) and varus forces [26–28]. Overall LCL complex remains taut throughout the range of motion of elbow flexion and extension [1].


Fig. 1.5
Lateral collateral ligament complex with lateral ulnar collateral, radial collateral, annular and accessory collateral ligaments. Used with permission of Mayo Foundation for Medical and Educational Research. All rights reserved [3]
The RCL originates from the lateral epicondyle and is a complex of several components that insert on the annular ligament. It is approximately 20 mm long and 8 mm wide. Its superficial surface is the location of the supinator muscle origin [3]. It is nearly isometric throughout elbow flexion and extension with its greatest contribution to stability in extension [29]. Morrey et al. demonstrated that the RCL contributes 14 % of varus stability in extension and 9 % in 90° flexion [24]. More recent anatomical studies have attributed a more significant role to the RCL in preventing PLRI [30].
The LUCL was originally described by Morrey and An [17]. It is a thickening of the ligamentocapsular complex that originates from the lateral epicondyle, blends into the fibers of the annular ligament and terminates on the supinator crest, deep to the fascia surrounding the extensor carpi ulnaris and supinator muscles [3, 31]. The humeral attachment of the LUCL is a well-defined area on the lateral side of the elbow at the isometric point [32]. Its biomechanical role is to stabilize the elbow during varus stress as well as serving as a posterior buttress to prevent radiocapitellar subluxation [31]. O’Driscoll described the role of the LUCL as preventing ulnohumeral rotation along the long axis of the ulna [28]. Investigations have shown that isolated lateral ulnar collateral or radial collateral ligament injuries do not result in instability but rather, combined injuries are necessary to create functional instability [30, 33].
Lastly, the annular ligament is an important stabilizer of the proximal radioulnar and radiocapitellar joints. It originates and inserts on the anterior and posterior margins of the lesser sigmoid notch, encircling the radius but not attaching to it [3, 31]. The more distal aspect of the ligament has a smaller radius to more tightly contain the radial neck [31]. It serves as the site of origin for the supinator muscle, with deep muscle fibers intimately fused with the ligament [34]. Transection of the annular ligament results in medial-lateral and anterior-posterior translation of the radial head by 44 % and 24 %, respectively [35]. Dunning et al. demonstrated that when the annular ligament is intact, either the RCL or LUCL can be transected without creating significant PLRI, demonstrating the annular ligament’s role as a primary static stabilizer [33].
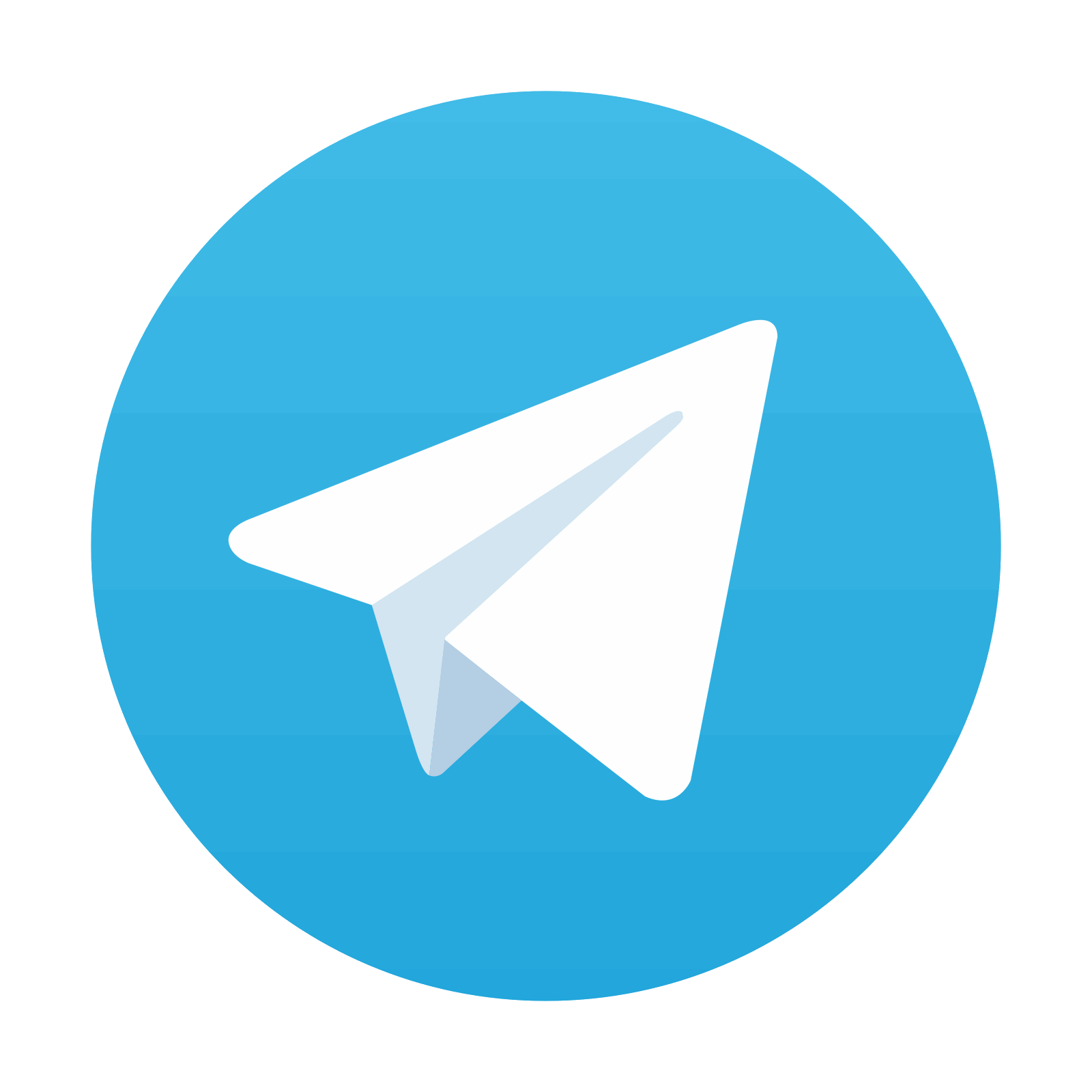
Stay updated, free articles. Join our Telegram channel

Full access? Get Clinical Tree
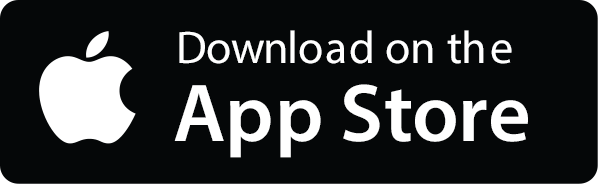
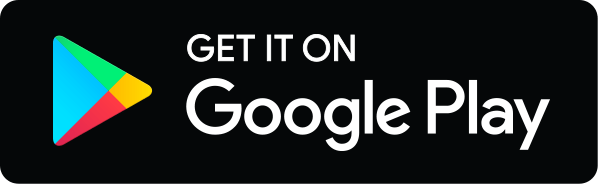