Aging and the central nervous system
Edward James R. Gorgon, Rolando T. Lazaro and Darcy A. Umphred
Introduction
Changes that occur in the central nervous system (CNS) with aging can be discussed at a cellular level such as mitochondrial function, at a system level such as the size of a nuclear mass, at a functional level such as the ability to stand up, or at a social level in terms of the capacity to interact and communicate. These changes, however, do not necessarily translate into limitations in the ability to engage in preferred activities and meaningful participations among healthy older people. Maintaining good health and independent function is possible in advanced age. It has been established, however, that activity limitations and participation restrictions are common among older people (Wilkie et al., 2006). Multiple-system physiological decline in old age and the complex effects of chronic pathologies and significant acute diseases interact and result in diminished functional capacity in this population (Fried & Guralnik, 1997). It is therefore important to differentiate changes in the CNS that occur with healthy aging from those that are caused by disease or pathology (Woodford & George, 2011). Recent work investigating CNS changes in aging suggests that neuroplasticity is a strong driver in the variability of functional performance in older people. This will be discussed in more detail in the latter portion of this chapter.
As humans age, their activity level changes and their activity choices, nutritional intake and general health vary tremendously. Genetic predisposition as well as environmental factors account for how the CNS acts and reacts in an aging individual, so it becomes difficult to compare one individual with another. The accumulation of minor and major traumas, exposure to toxins and other environmental factors, and overuse and disuse of major body systems affect the function of the CNS. Therefore, difficulties arise when asking, ‘What is expected with normal aging?’ Yet, when one looks specifically at the CNS, certain changes are observed with aging. These changes may not immediately result in disease, impairments, activity limitations or participation restrictions. However, the cumulative effects of these changes may dramatically influence an aging adult’s ability to compensate and relearn once a specific pathology has created functional loss and a decrease in quality of life.
Nervous system changes with aging
Multiple cross-sectional and longitudinal studies have established that in the brain there is a decrease in regional volume and gyral thickness with an increase in ventricular size in advanced age (Long et al., 2012). Substantial changes in brain regional volumes have been demonstrated, especially for the caudate and cerebellum, and, to a similar extent, the prefrontal and inferior parietal cortices and hippocampus (Raz et al., 2005). However, in the healthy aging human, there is no conclusive research showing that these and other changes, such as those in neurotransmitters, are related to impairment in function. Loss of conduction velocity in sensory and motor nerves within the central and peripheral nervous systems as well as loss of myelin sheaths and the large myelinated fibers with advancing age have also been reported (Werner et al., 2012). Although these losses might appear to explain a propensity for falling as a result of delays in sensory information processing and motor response, a connection between a deficiency in one part of the system and the overall function of an individual has not been proven. Further, it is not understood why some individuals can function well into very old age without severe functional loss whereas others cannot.
Changes in sensation
Changes that occur in both the visual and auditory systems with aging have been documented (Stephen et al., 2010). As noted in Chapter 51, visual acuity may decline gradually from middle age onward and may decrease rapidly in the eighth decade of life (Sjöstrand et al., 2011). Traditional testing of acuity is valid for reading but not for functional tasks such as observing walking surface variations in a darkened room. Other common changes, such as altered color discrimination and light adaptation, can translate to impaired vision and may add to any visual acuity deficit. For an individual to respond appropriately, it requires receiving an input, processing that input both perceptually and cognitively at an intellectual level or automatically at a motor level, and finally selecting the motor response that best matches the environmental requirements. An individual who wears bifocal or trifocal lenses and glances down for visually augmented feedback may see a distorted image, so inaccurate information is sent to the CNS. Thus, the motor response may be appropriate for the input being received but inappropriate for the actual environment. Conversely, an individual with visual impairment may respond adequately to environmental demands and show no signs of motor limitation. The nervous system functions on consensus and will always use other sensory systems and prior learning, if available, to determine how to respond to visual information.
As discussed in Chapter 52, hearing loss is also common among older people, the causes of which are either peripheral or central deficits generally associated with disease or biological aging (Chisolm et al., 2003). Loss of auditory acuity translates to difficulty in hearing and word recognition, or difficulty carrying on a normal conversation within a noisy environment, which may result in isolation and self-exclusion from participation in group activities. Although hearing loss itself does not impair movement, often, when the auditory portion of the eighth cranial nerve is involved, the vestibular portion is also affected (Møller, 2000). This can potentially result in vertigo and impaired balance, and increase an individual’s risk of falling (Ishiyama, 2009).
Changes in cognitive function and the limbic system
The processing of information in the cognitive and emotional areas of the CNS cannot be ignored when considering CNS changes with aging, with or without pathology. Subtle non-pathological declines do occur with aging in key cognitive domains and correlate with, but not necessarily impair, the ability to carry out daily function (Vance et al., 2010). Changes across cognitive domains and across older individuals are not uniform, with some cognitive functions demonstrating greater extents of change than others (Glisky, 2007). This underscores the need to consider the complexity of cognitive decline in clients undergoing rehabilitation. Healthcare providers must remember that when the processing or learning of cognitive materials becomes a problem, procedural learning of motor programs will become the only avenue to regain functional control over movement. Principles of motor learning then become paramount in optimizing the therapeutic environment for client improvement (Umphred et al., 2013: 69).
The healthcare provider must consider the emotional (limbic) system in all clients, especially when looking at CNS function. Emotions are controlled and modified by the limbic system. This system has extensive connections to the hypothalamus, so emotion is often expressed through regulation of the autonomic nervous system and in the tone of the striated muscle system (Groenewegen & Uylings, 2000). The hypothalamus regulates the areas of the brainstem that control the heart, lungs, internal organs and immune system.
Many behavioral syndromes have been attributed to this area of the brain. The most pertinent to older people, the ‘general adaptive syndrome’ (GAS), was described by Hans Selye in 1956 (Stojanovich, 2010; Umphred et al., 2013: 111). Today, it is considered a response to stress and can be observed in any frail individual, including older persons with fragile bodily systems. The GAS response is paradoxical to the anticipated response. Under stress, an individual generally has a sympathetic response, with an increase in heart rate and blood pressure and a fight–flight reaction. In the GAS, the same environmental conditions initially cause a sympathetic response but, over time and sometimes quickly, individuals may experience a switch to a parasympathetic reaction. Blood pressure drops, heart rate decreases, blood pools in the periphery and level of consciousness can drop. The GAS is a survival response to stress because, without such a response, the increase in heart rate and blood pressure would cause heart failure or vascular rupture. Treatment based on the GAS signs and symptoms may be aimed at increasing the sympathetic response, which may cause an even greater paradoxical reaction.
Research since 1956 has shown that hormone levels increase with stress (Lupien et al., 2009) and the amount of hormones elicited increases with age (McEwen, 2002). By virtue of advanced age, a client may be very close to multiple-system failure and would be considered frail. A client may have recently developed a disease that adds stress to an already frail system. If the corresponding treatment creates more stress in the individual, it may result in a GAS, which has the potential of evolving into a life-threatening situation. The client’s response may be to withdraw while the healthcare provider’s reaction might be to increase the level of input to motivate or heighten arousal in the client. With further withdrawal, the client can potentially die from heart failure from diminished heart rate and blood pressure. The healthcare provider should thus evaluate the client’s emotional response to the environment and try to keep a homeostatic autonomic balance. This requires being sensitive to body functions controlled by the limbic system such as respiration, blood pressure and level of alertness, as well as specific motor responses to interventions (Umphred et al., 2013: 111).
Changes in the motor system
The motor system, with the guidance of prior learning and experience as well as analysis by the CNS of current needs, modulates the state of the motor pools in the brainstem and spinal cord. This modulation drives peripheral nerves and orchestrates synergistic interactions of muscle groups to create functional behavior. Ultimate control of human behavior is the result of the consensus of a variety of areas within the motor system. Understanding how this motor system controls movement is the key to identifying motor impairments and understanding activity limitations.
The areas typically considered to be part of the motor system are the premotor and motor areas of the frontal lobes, basal ganglia, cerebellum, brainstem, spinal cord and all the interneurons that link these systems. The thalamus plays a key role as a relay station and modulator whereas the limbic system has the ability to alter the state of motor responses both directly and indirectly (Kandel et al., 2012). The sensory areas guide and alter existing motor programs. Where the motor system begins and ends is not clear because there are so many interdependent systems that loop between one area and another. Therefore, a linear analysis is not appropriate. There is not one specific area that controls motor output, yet certain nuclear masses are responsible for specific aspects of motor function. When any one of these areas is impaired, specific clinical symptoms manifest. Motor control over base tone in striated muscles is regulated by many areas, so a deficit does not automatically reflect the involvement of a specific area. A deficit in one loop of many interconnected neuronal loops might present a clinical problem that would make it appear as if a nuclear mass or system was damaged.
Research does show that the motor system changes with age (Mora et al., 2007), although such changes cannot account entirely for the functional changes observed in older people (Mahncke et al., 2006). For example, it is accepted that there is an age differential when looking at modulation of the H-reflex which may be due to the aging neuromuscular system (Koceja & Mynark, 2000). Similarly, control over the Ia fibers as a readiness state for spontaneous movement decreases with aging (Mankovsky et al., 1982) and may be associated with a decrease in the ability to modulate inhibitory function (Fujiyama et al., 2009). Moreover, proprioceptive input has less influence on the motor cortex excitability (Degardin et al., 2011). These suggest that an aging CNS no longer has the refined regulating ability over preprogrammed synergistic patterning. Comparing performance of younger adults to older adults indicates that older people possibly begin to lose some movement automaticity and compensate through increased brain network activity (Wu & Hallett, 2005). Whether these changes are due to disuse or aging is not clear. As there are central, muscle and mechanical changes with aging, a decrease in functional ability is considered multifactorial (Degardin et al., 2011). The significance of these changes may be more meaningful following injury or disease. If the aging CNS loses some of its plasticity or ability to adapt, then it may take more time to learn new programs or alter existing ones. This might explain why aging adults seem to exhibit synergistic patterns very quickly following injury, whereas younger adults may take more time to develop the same abnormal patterns, given appropriate conditions.
A complete explanation of the specifics of the motor control system (Shumway-Cook & Woollacott, 2012) is not within the scope of this chapter, but a brief overview of the system might help the reader to understand and appreciate its complexity. The frontal lobe of the brain not only helps process the motivation to move (prefrontal) but also modulates information that travels between primary motor centers such as the basal ganglia and cerebellum (Kandel et al., 2012). In addition, it plays a primary role in regulating, but not dictating, fine and gross motor function through the corticospinal and corticobulbar systems. Normal movement results from the coordinated work of multiple areas that influence the final common pathways of motor neurons. After summating and modulating messages from other areas of the CNS, the frontal lobe sends messages concurrently to the basal ganglia and cerebellum (Kandel et al., 2012). In turn, these centers formulate new motor plans or draw upon existing plans to correctly modulate the motor system. If either a center or the loops connecting the centers is damaged or diseased, then motor function may be incoordinated and unsuited to the environmental context of the activity. The cerebellum, unlike the basal ganglia, is simultaneously aware of the peripheral kinematics through input from proprioceptors in the limbs and trunk, and from the vestibular system based on the position of the head in space. Similarly, the cerebellum is constantly updated on the existing states of the motor pool through ascending tracts that send that information directly to the anterior cerebellar lobes. Thus, the cerebellum is considered a synergistic programmer and plays a key role in feed-forward regulation of movement.
The role of the cerebellum in higher-center regulation of motor programming interfaces with the limbic system as well as the frontal lobe and basal ganglia. Deficits within the frontal lobe, limbic system or basal ganglia can affect cerebellar function and have been shown to trigger, what appears to be, cerebellar neuronal damage (Umphred et al., 2013: 601, 631–7). The cerebellum is not only responsible for helping write new programs, it also ensures that the programs being used at any one moment match the environmental context. If there is a mismatch between the desired movement sequence and environmental stimuli, the cerebellum will try to readjust the synergistic patterns and run the program that matches the environmental demands. For example, if an individual is walking on a level cement surface and suddenly the surface changes because of a crack or a hole, the cerebellum will adjust balance strategies in order to regain the center of gravity and hence allow the person to keep walking. If the perturbation created is too large, such that the cerebellum cannot correct the patterns being run, consensus of the CNS will determine what new program should be initiated.
The basal ganglia are responsible for changing the plan of movement and initiating new programs, whereas the cerebellum, in preparation for the changes to take place, regulates the state of the motor generators (base tone) and controls the force, speed and direction of movement. For example, if an individual is rising to a standing position and goes beyond vertical and starts to fall, the basal ganglia change the motor setting from rising to vertical to falling. Both the basal ganglia and cerebellum play roles in modulating posture but the specific roles are different. The basal ganglia and aspects of the cerebellum contribute in the development of new motor programs and refinement of existing ones. Both relay specific motor programming to the frontal lobes through the thalamus and down to the motor generators of the cranial and spinal neurons through the brainstem. Therefore, changes in any of these structures or in the pathways between them that occur with aging could have critical effects on normal movement. Along with pathology, such changes can become cumulative and eventually result in altered motor performance and loss of function. For example, a reported loss with age of Purkinje cells in the cerebellum has not been related to movement impairment (Rajput et al., 2011). However, this change along with cerebellar degeneration or a cerebellar stroke would be cumulative and the result might be a greater deficit than would occur in a young adult without Purkinje cell loss who develops a similar pathology.
Healthy aging and pathological aging in the CNS
Various studies have endeavored to describe changes in the CNS that occur with healthy aging by differentiating those with changes that mark pathological aging. Longitudinal studies have reported brain loss and multiple-domain cognitive decline over time in healthy older people, with significantly extensive changes observed on follow-up measurement in those who eventually developed mild cognitive impairment or dementia, compared to those who did not (Johnson et al., 2009). Evidence suggests that in healthy aging, brain shrinkage can be minimal and does not appear to correlate with any important limitation in functional ability. In terms of neuropathologic changes, such as the development of senile plaques or neurofibrillary tangles, even in the ‘oldest old’, or people who were at least 85 years of age, such change can be minimal and cognitive ability can remain stable (Green et al., 2000). These normal aging-related changes are in stark contrast to the severe neuronal loss found in people with neurodegenerative conditions such as Alzheimer’s disease, frontotemporal dementia and Parkinson’s disease (Yankner et al., 2008), and the extensive neuropathologic changes and steep cognitive decline seen in oldest old people with Alzheimer’s dementia (Green et al., 2000). Literature supports the concept that older people can be healthy and functionally stable into advanced age, and pathological conditions precede and determine functional loss. The aging process may affect the potential of the nervous system to adapt, but age is only one factor that influences that potential. Aging should never be considered the primary cause of functional limitations or restrictions in normal life activities.
Models of rehabilitation for older people
It is important for clinicians to follow a rehabilitation model that allows them to understand the relationship between the health condition, consequent abnormalities in body structure and function, and the impact of these on, as well as interactions with, an individual’s physical and societal functioning. In this chapter, the International Classification of Functioning, Disability and Health (the ICF model) will be briefly discussed and supplemented by the Behavioral model for evaluation and intervention of human movement performance.
International classification of functioning, disability and health (ICF) model
The ICF model (Fig. 5.1) is the second World Health Organization (WHO) model, introduced in 2001 in the hope of forging a universal language for functioning, disability and health (WHO, 2001). This model is considered to be an empowerment model and based on the strengths of the individual. It replaces the previous disablement model (the International Classification of Impairments, Disabilities and Handicaps, or ICIDH), which identified the healthy individual from one who had a disability associated with a health condition. The new model replaces the terminology of impairment, disability and handicap with positive words that define bodily functions and structures, activities and participation, and highlights the impact of personal factors and of the environment and society on disablement (Guralnik & Ferrucci, 2009). The ICF is underpinned by concepts similar to those of the Nagi model and, by virtue of support from a worldwide consensus, this model is expected to be used universally (Jette, 2009).
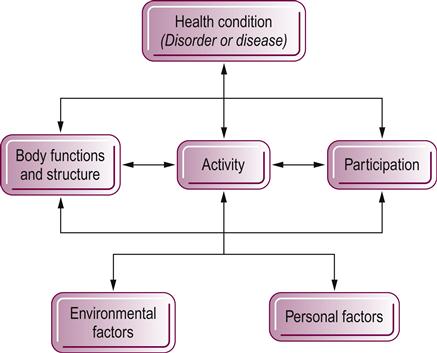
In this model, the identification of functional bodily structures and functions relates to those systems and subsystems that are normal; abnormalities in these systems are also called impairments. The ICF defines activities as tasks, functional behaviors and activities of daily life that can be executed by the individual as well as those activities that are limited (‘activity limitations’). Participation in life identifies the social or family engagements of the individual and the restrictions in those engagements (‘participation restrictions’). Often therapists begin their examination at either an activity or life participation entry point. The client presents with changes in normal activities and the desire to return to a better quality of life motivates participation in the rehabilitation process. The therapist conducts a comprehensive assessment covering the multiple relevant body functions and structures that contribute and interact to produce the activity limitations. As the therapist examines the functional activities category, environmental and personal variables that may affect activity and function must be considered within the framework of the whole person (Gordon et al., 2006). Intervention might include strategies aimed at ameliorating impairments and activity limitations, and addressing personal and contextual barriers, to optimize functioning and participation. However, as the client starts to re-engage in valued activities and participations, positive changes in body functions and structures as well as activities may also be driven by such engagement. Thus, the arrows within Figure 5.1 can go in either direction and the model posits that an improvement in participation can have a positive impact on the other dimensions of health (Guralnik & Ferrucci, 2009).
The use of the ICF as a client-oriented problem-solving model has been described previously through frameworks and instruments developed for analyzing client problems, identifying specific rehabilitation goals and establishing links between disabilities and relevant and modifiable variables that form the basis for intervention and outcome measurement (Rauch et al., 2008). The ICF shifts focus from the cause to the impact of disease and disability (Jette, 2006). The deleterious effects of health conditions on older people pervades beyond activities and extends into household, community, leisure or sports participations (Alma et al., 2011) and quality of life (Marengoni et al., 2011). Participation restrictions are common among community-dwelling older people and onset and persistence increase with age (Wilkie et al., 2008; Wilkie et al., 2006). The goal for therapists therefore is always to guide the client toward the desired participations and therefore an enhanced quality of life. Interventions need not always be aimed at the level of impairments and activity limitations. Using the ICF can provide therapists with a broader view of how clients live with disease and disability, which, in turn, can be the basis for more holistic interventions.
The behavioral model
Therapists are expected to identify the specific components that have led to functional problems, predict how long it will take to correct or develop compensation for the problem, and establish a plan of care that will allow the client to become functional in the shortest time possible. This responsibility of differential diagnosis is closely interrelated with the ICF concepts of specific system impairments, functional strengths or activity limitations, and adaptation that allows for participation in life and retention of the highest quality of life possible, as shown in Figure 5.2. The relationships are multifactorial and do not necessarily proceed in a linear or unidirectional fashion. Many risk factors both inherent and external to the individual need to be considered as part of this diagnostic process. Inherent risk factors include all bodily systems that may be changing due to age, such as the nervous or cardiopulmonary systems, and may or may not have anything to do with pathology or disease. External risk factors are environmental and can be based on structural limitations, such as stairs or tri-level dwellings, or more socially driven, such as family beliefs regarding aging. As movement specialists, therapists need to look at motor behaviors and how those movement patterns assist in creating the ability to perform functional activities and independence that leads to life participation. Similarly, therapists need to simultaneously recognize how movement limitations restrict performance of both function and participation and determine whether those limitations can be reduced, corrected or adapted to give the individual a higher quality of life. The consumer determines what he or she wants to participate in and defines what quality of life is. The therapist always needs to remember and not assume that all individuals regain function similarly or that the function the therapist values is the same as the client’s. These movement diagnoses are behavior driven and not disease or pathology driven, as is a medical diagnosis.
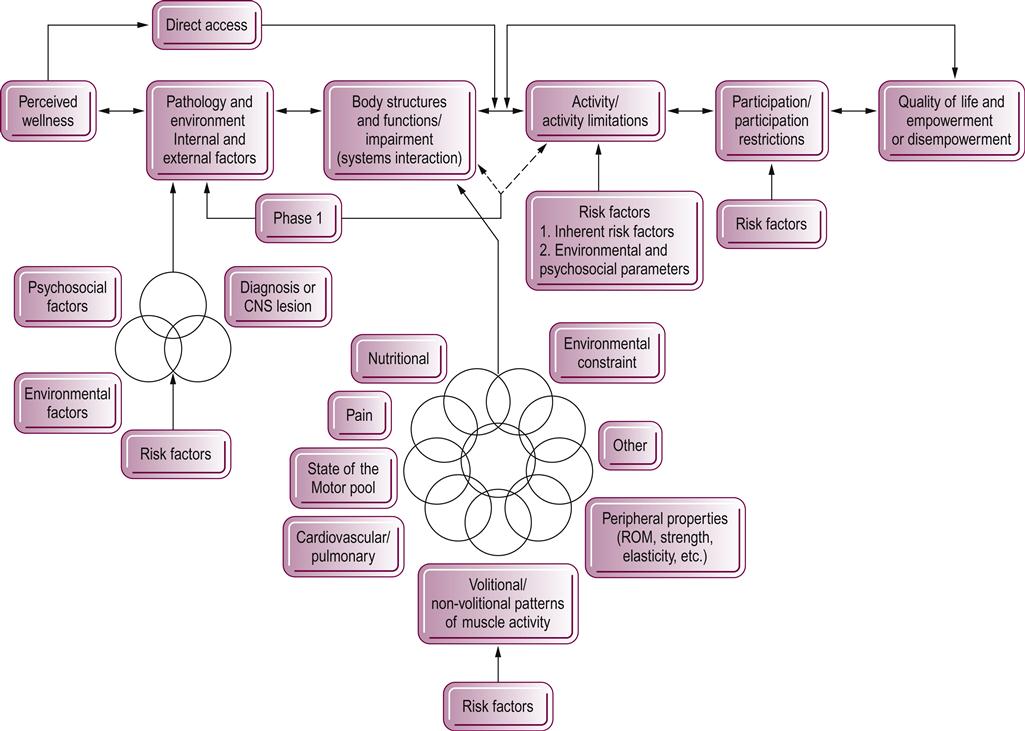
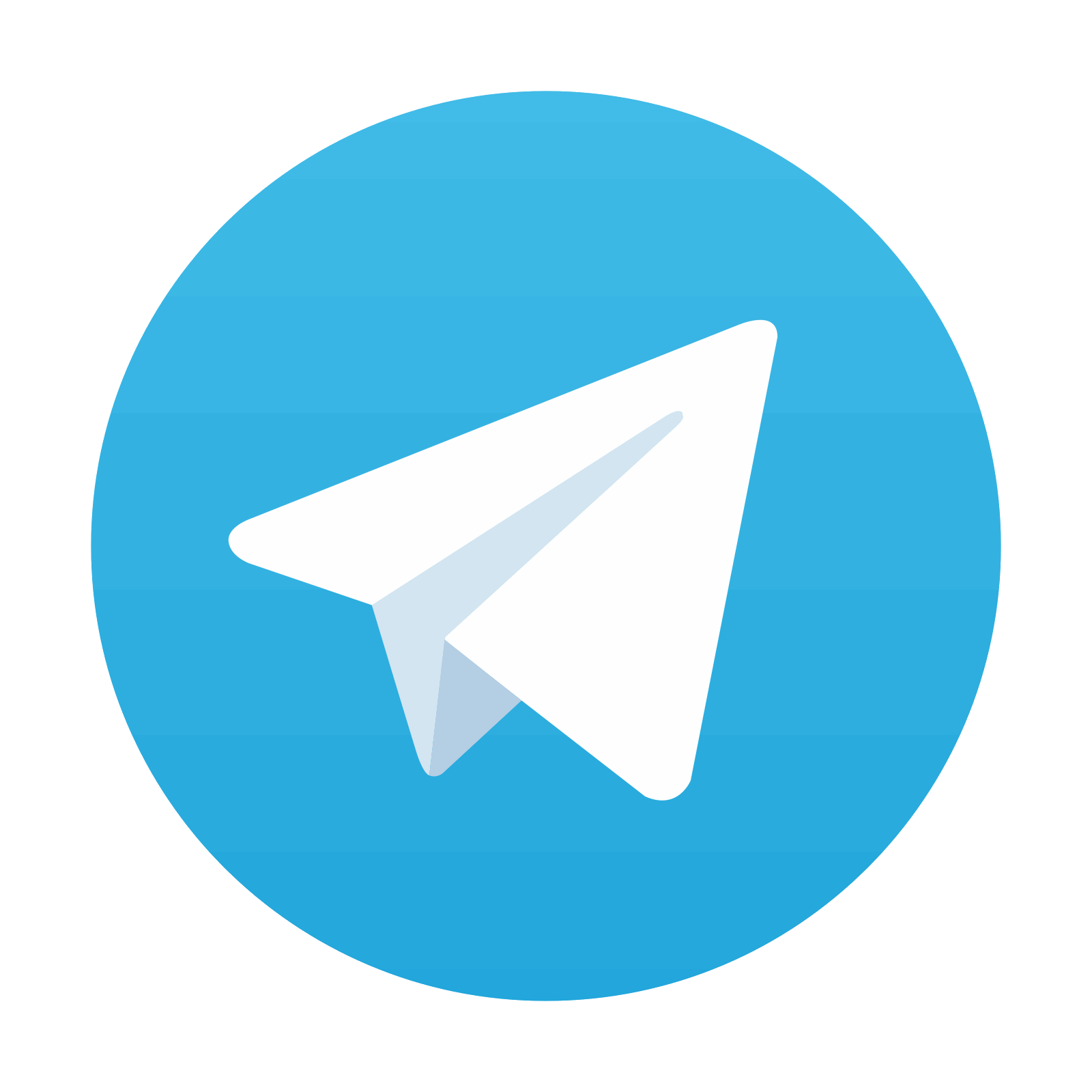
Stay updated, free articles. Join our Telegram channel

Full access? Get Clinical Tree
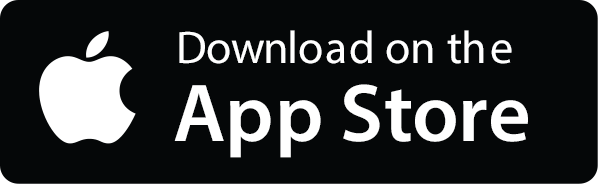
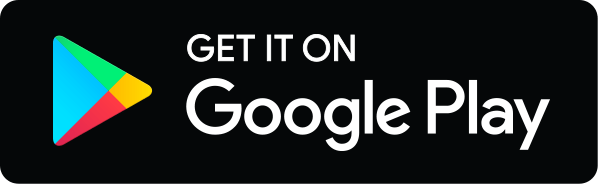