Fig. 4.1
From foetal to old-age disk – macroscopy of the intervertebral disk. (a) Foetal lumbar spine (36th week of gestation) vs. (b) old-aged spine (86 years) showing the tremendous changes of the disk structure during age
The nucleus pulposus (NP) is a gelatinous mass filling a central “core” of the disk between the two end plates and the ring-like fibres of the annulus fibrosus (AF). It is made up of a particular type of cartilage which contains relatively few chondrocytic cells and an abundant matrix mainly of proteoglycans (aggrecan) along with moderate amounts of collagen molecules (mainly collagen type II). The high concentration of proteoglycans leads to significant binding of water and swelling of the highly hydrated NP the extension of which is limited by the adjacent structures. Therefore, the NP has the main function of a “shock absorber”, a cushion compression, providing both stiffness and flexibility of the motion segment.
The annulus fibrosus (AF) is comprised of dense sheets of highly oriented collagen fibres in concentric lamellae running obliquely between adjacent vertebral bodies. The angle relative to the vertebral bodies alternates from one lamella to the next resulting in a cross-woven network. The AF can be subdivided into an inner and outer part. The inner AF represents a structure close to that of the NP, while the outer AF is composed of a densely organised structure with thick bundles of collagen as the main constituent. The fibre network allows some relative movement between the bundles, thereby providing a certain degree of disk deformation during flexion and extension of the vertebral column. The main structural component of the AF is collagen (mainly made of collagen types I and III, with some type II collagen in the inner AF, but no collagen II in the outer AF). The content of proteoglycans is significantly lower than in the NP and does not serve mainly as a water adsorbent, but these proteoglycans are important for a proper interaction of the collagen lamellae and therefore for a regular function of the AF fibre network.
The normal disk is framed by two end plates (EP) forming each a sheet of hyaline cartilage adjacent to the upper and lower vertebral body which is very similar to articular hyaline cartilage. This layer of cartilage separates both the NP and the AF from the vertebral bones providing a firm attachment of AF fibres to the bone structures. Therefore, the end plate is essential for the rigidity of the motion segment. In addition, the end plate is of significance for the nutrition of discal structures, as the major part of the disk is nourished via diffusion of oxygen and nutrients from the bone marrow spaces of the vertebral bones (in “normal” adult disks, there exists only minor vascularisation of the outer AF from small capillary vessels). Structurally, the EP is composed of a fine collagenous network mainly made up by collagen type II fibrils along with proteoglycans. The orientation of the collagen fibres as horizontal layers provides significant rigidity of the motion segment. At birth, the human cartilage end plates make up approximately 50 % of the intervertebral space (compared with approximately 5 % in the adult) and have large vascular channels running through them. Soon after birth, the vascular channels of the cartilage end plate fill in with extracellular matrix such that no channels remain by the end of the first life decade.
Besides the NP, the AF and the EP, the disk is surrounded by various other structural elements that contribute to the function of the motion segment. This holds particularly true for the anterior and posterior longitudinal ligaments which provide further stiffness, but also some flexibility of the spine and which delineate important adjacent structures such as the spinal canal. The ligament zone is important not only with respect to the vascular supply but also with regard to a significant innervation which may be relevant during the induction of disk pain.
The vertebral bodies are further functional elements of the spine which intimately interact with the disks. These osseous bodies significantly stabilise the vertebral column, but they are also important with respect to the nutrition of the various disk structures since the bone marrow cavity is filled with blood with low perfusion velocity.
Under physiological circumstances, these various structures interact properly with each others. There exist, however, significant influences of structure and thus function with different stages of age. Therefore, age-related changes have to be taken into account when differentiated from pathological alterations.
4.3 The Morphology of the Disk During Development and Ageing
The disk and its various substructures undergo significant macro- and micromorphological changes with advancing age which are important for the evaluation of DDD. Again, the disk has to be considered as a whole, but alterations of the end plates seem to be particularly important.
4.3.1 Embryonal and Foetal Development
The vertebral column is already determined during very early embryogenesis (Fig. 4.2). At about 4 weeks gestational age, the human spine can be identified as a typical series of vertebral bodies. These form under the combined influence of the notochord and neuronal tube. The disk grows initially in an environment with only few blood vessels and is surrounded by a perichondrial layer which forms the future longitudinal ligaments. Between the vertebrae, the notochord expands as local aggregations of cells, the notochordal cells, within a proteoglycan-rich matrix, forming the gelatinous centre which results in the NP (Fig. 4.2). The circularly arranged fibres surrounding the NP finally form the AF which is derived from the perichordal mesenchyme. With ongoing development, the notochordal cells are replaced by chondrocytic cells, while the cells of the AF region have a more fibroblastic phenotype. As yet, the exact role and interaction of notochordal, chondrocytic and fibroblastic cells within the developing disk remains unclear. Recent studies suggest, however, that there exists a significant communication between those cells leading to the final disk structure [4]. This synergy may be of importance in maintaining a normal, “non-degenerated” disk. Interestingly, the notochordal cells disappear from the human disk during early infancy. This is in contrast to various species, such as cat, pig, mouse, rat, rabbit and others where notochordal cells persist in the nucleus pulposus into adulthood. As yet, it remains speculative whether those animals with persisting notochordal cells have a much lesser degree of disk degeneration or if other factors may be the cause therefore. In summary, the loss of notochordal cells may be seen as an important, initial step towards ageing of the disk.


Fig. 4.2
Anatomy of the intervertebral disk. (a) Schematic presentation of the motion segment and the disk. (b) Macroscopically the central nucleus pulposus (NP) is surrounded by the annulus fibrosus (AF). (c) Histologically the very different fibre structure of the various structures is evident
A further significant structural peculiarity of foetal (and early infantile; see below) disks is the presence of blood vessels within the disk (Fig. 4.3). These are mainly formed by large vessel “loops” which originate from bone marrow vessels, perforate the EP and extend into the inner AF close to the NP. These vessels have thin walls such as seen in venules. Due to the dimension, it can be assumed that they provide a major blood supply to all disk structures. Only to a significantly fewer part, small vessels of the capillary type extend from the longitudinal ligaments into the AF, but not into more distant structures. Accordingly, they are restricted to the outer AF.


Fig. 4.3
Embryonal vertebra (11th week of gestation). (a) The overview shows the typical segmentation; in the centre (b) islands of notochordal cells are visible (arrow) which can selectively be labelled by immunohistochemistry (brown reaction product, cytokeratin 8, c)
During the postnatal development, three major time periods can be separated with respect to morphological age-related changes. These are infancy and early adolescence (c. 0–17 years), young and medium adulthood (c. 18–60 years) and advanced age (more than 60 years). These time periods are characterised by significant structural changes affecting the various anatomic sub-settings differently but leading to a progressive loss of “normal” structure and thus function (Fig. 4.4). Any distinction between “physiological” ageing and “pathological” degeneration is highly problematic and should be oriented at the clinical situation, i.e. at the presence/absence of pain and/or disabling loss of function.


Fig. 4.4
Changes in disk vascularisation. The foetal end plate contains large blood vessels (a) which are obliterated during the first postnatal months (b). There, small islands of irregular cartilage indicate the residues of the previous vasculature
4.3.2 The Disk of Infants and Adolescents
Besides the loss of notochordal cells which gradually is seen between the prenatal period to an age of approx. 4 years, the most important structural change is a complete loss of disk vascularisation (see above, also Fig. 4.3). This is closely related to changes in the EP structure and is seen in infants of few months of age presenting with regressively obliterated vessel residues. In a series of autopsy cases of lumbar spines, we did not find intradiscal vessels originating from the bone marrow space as early as 4 years of age [1]. Coincidently with these two features, even the infantile and early adolescent lumbar disk provided focal, minor and very initial morphological changes which suggest initial “degeneration”. Likewise, we observed foci of granular matrix alteration, beginning clonal chondrocyte proliferation and initial small disruptions of the matrix even in this age group, mostly restricted to the NP without alterations of the AF. In the EP mainly those areas with presumably obliterated blood vessels show a disturbance of the EP structure. The extent of all these changes correlated well with the increasing age [1].
4.3.3 The Disks of Young and Medium-Aged Adults
With further proceeding age, the extent and degree of the aforementioned changes increases (Figs. 4.4 and 4.5). The most dramatic rise in morphological signs for tissue degeneration is seen at the end of puberty when the rapid growth process has led to a significant increase of diffusion distances within the disk. This impairment of the disk nutrition has previously been made responsible for most degeneration-associated morphological changes. However, more recent concepts – that will have to be discussed below more in detail – suggest that several other factors seem to play an essential deleterious role during DDD. As the most typical morphological signs for disk degeneration, we previously identified significant tears and clefts of the disk matrix, an increasing amount of clonally growing “proliferating” chondrocytes, granular and mucoid matrix changes and the occurrence of decaying cells (Fig. 4.6) [1]. The changes are seen first in the NP, but frequently extend to the inner AF and to lesser extent to the outer AF. Then the outer AF provides focal clefts which – when in connection with similar tears and clefts of the inner AF and the NP – may lead to protrusions and even prolapse of the disk into the spinal canal. Those changes are then often associated with an “inflammatory” reaction as seen by the in-growth of capillary blood vessels and histiocytic cells into the affected AF areas.



Fig. 4.5
Macroscopic aspects of the intervertebral disk with increasing age. This figure shows typical macroscopic examples of age-related changes of the disk. The alterations with increasing age are used in the classification by Thompson in five grades: I (a), II (b), III (c), IV (d) and V (e)

Fig. 4.6
Histology of complete motion segments of intervertebral disks with various age. (a) The section through the complete disk of a young adult individual shows regular features of disk morphology. However, despite the young age, the disk reveals already some clefts within the nucleus pulposus. (b) The disk of a 62-year-old individual in contrast demonstrates a much more irregular shape of the disk with extensive clefts and tears of NP and AF. Furthermore, the disk shows reduced height and a lack of anatomical distinction between AF and NP. (c) The disk of a 77-year-old individual with very extensive alterations (when compared to b) with highly significant reduction in disk height and loss of disk tissues (a Masson’s trichrome; b, c alcian blue-PAS)
4.3.4 The Disks of Adults of Advanced Age
Finally, the age-related changes in the old-age group are characterised by even more pronounced morphological signs of disk degeneration. While part of those cases that have been investigated as yet have shown extensive clefts, a loss of disk height, chondrocytic proliferation and extensive mucoid matrix degeneration (Fig. 4.6b, c), we identified individuals with a transition into a scar-like morphology with loss of the chondroid matrix and replacement by a more or less “fibrous” tissue. Those disks frequently reveal a complete loss of the disk tissue structure and can be termed as a “burnt-out” appearance.
4.3.5 Particular Morphologic Changes of the End Plate
Since the end plates form a very unique structure, in the context of this chapter, a particular view on its anatomic and developmental structure might be presented.
Within the thin cartilage end plates, collagen fibres run horizontal and parallel to the vertebral bodies along with the fibres continuing into the disk. This separates both the NP and the AF from the vertebral bones and provides a firm attachment of AF fibres to the bone structures. Therefore, the end plate is essential for the rigidity of the motion segment. At birth, the human cartilage end plates make up approximately 50 % of the complete intervertebral space (compared with approximately 5 % in the adult). They have large vascular channels running through them. Soon after birth, the vascular channels of the cartilage end plate fill in with extracellular matrix such that no channels remain by the end of the first life decade. This change gains even more significance as the disk grows strongly in size during puberty, and therefore, the distances between the vascular supply and the disk cells elongate substantially (see below).
The cartilage end plate in humans functions in early life as a growth plate for the adjacent vertebral body; its structure is typical of that seen in the epiphyseal growth plates of long bones. This structure is lost during skeletal maturity. By adulthood, the cartilage end plate is a layer of hyaline cartilage (approximately 0.6 mm thick) with calcified cartilage adjoining the bone. In adults, the end plate occupies the central 90 % of the interface between the disk and the vertebral body, encompassed by a ring of bone that forms via the epiphysis fusing with the vertebral body in the rim region. The end plate is totally avascular and aneural in all healthy adults.
Within the cartilage end plates, typical lacunar cartilage cells are surrounded by a small territorial matrix (thereby forming the typical functional unit of cartilage called “chondron”) which in turn is enveloped by the inter-territorial matrix. The latter is somewhat more “compact” than the territorial matrix which is reflected on the molecular level by differences in the collagen type distribution. The biomechanical properties of the end plate are defined by its extracellular matrix composition which includes collagen types II, III, V, VI, IX and X and major proteoglycans and glycoproteins. The composition of those constituents alters by age [5].
Functionally, the end plate is involved in two important mechanical functions [6]:
I.
Preventing the nucleus pulposus from bulging into the vertebral bodies
II.
Partially absorbing the hydrostatic pressure dissipated by the nucleus pulposus under loading. Similar to the disk, the ability of the end plate to withstand mechanical forces depends on the structural integrity of the matrix. In addition, the end plate is of significance for the nutrition of discal structures, as the major part of the disk is nourished via diffusion of oxygen and nutrients from the bone marrow spaces of the vertebral bones.
Age-related changes of the EP have been identified to comprise [7] fissure formation, fractures of calcified cartilage, horizontal cleft formation, death of chondrocytes (apoptosis), increased vascular penetration, extension of calcification and ossification. These changes start to occur in the third decade of life. A study of cadaveric human vertebrae demonstrated that the number of vascular channels perforating the osseous vertebral end plate diminishes drastically between 6 and 30 months of age [8]. Analyses on the microscopic level revealed that the abundance of obliterated blood vessels in the end plate gradually increases between 1 month and 16 years of age. The decrease in blood vessels is paralleled by an increase of cartilage disorganisation, end plate cell density, cartilage cracks and microfractures (Fig. 4.7).


Fig. 4.7
Typical microscopic alterations of disk degeneration. This figure shows characteristic microscopic features of disk degeneration: (a) Extensive clefting, originating in the NP, extends with ongoing degeneration to the AF. (b) Focal, “cluster”-like cell proliferation. (c) Small areas with typical mucoid matrix indicate substantial changes in the matrix composition; this can selectively be made visible in the alcian blue-PAS staining. (d) In late-stage degeneration tissue defects occur (a, c alcian blue-PAS; b H d Masson’s trichrome)
These changes, especially the loss of blood vessels, can cause nutritional consequences for the intervertebral disk (Fig. 4.8). With advanced degeneration and markedly reduced disk height, further changes of the end plate are induced resulting in complete end plate disarrangement and dense sclerosis of the adjacent vertebral bodies.


Fig. 4.8
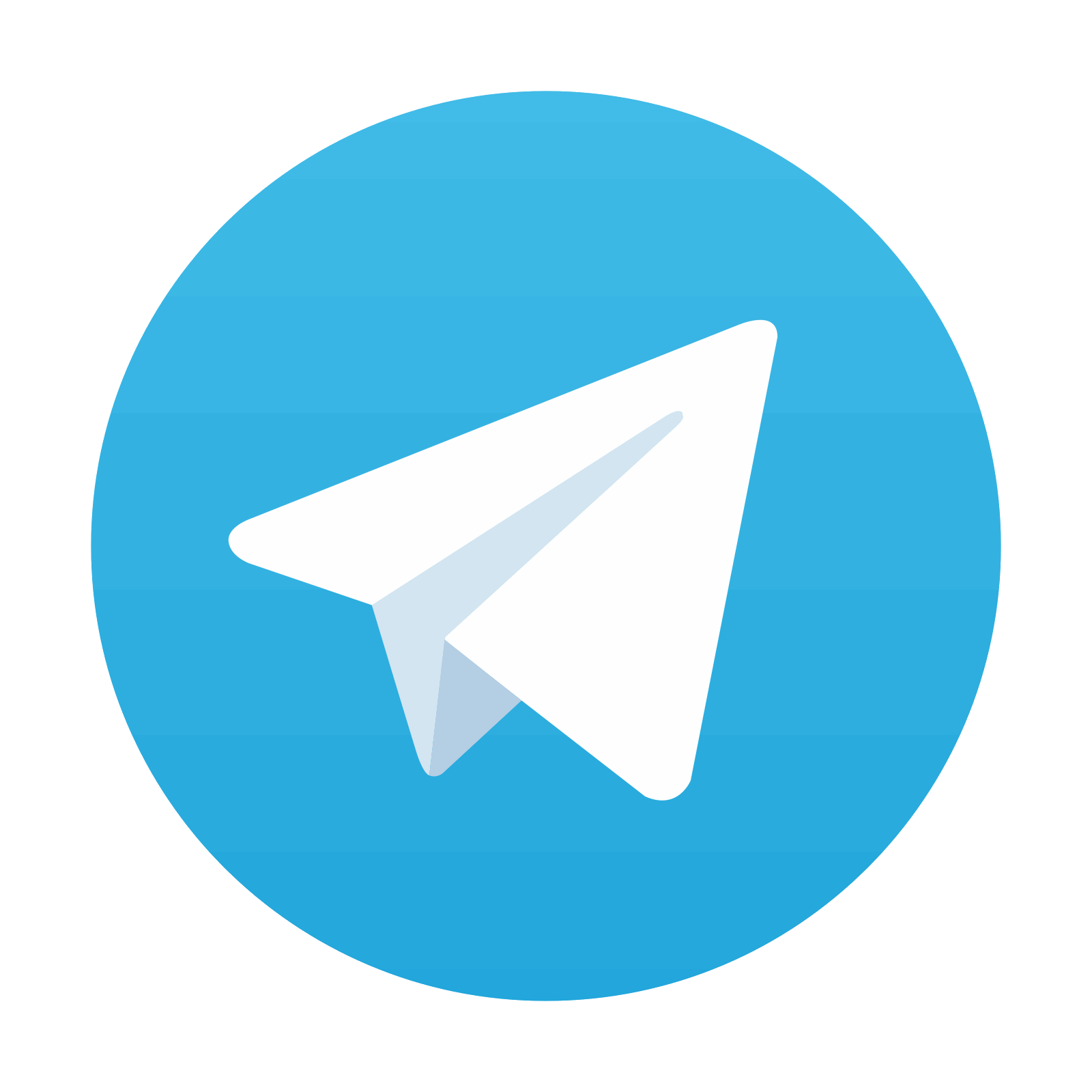
Biomechanical and nutritional conditions of the intervertebral disk. While the nucleus pulpous has high water-binding properties resulting in a cushion-like structure, the annulus fibrosus and the end plates frame the nucleus. However, the avascular disk structure requires long distance diffusion of oxygen and nutritional substances from the bone marrow to the central portions of the disk
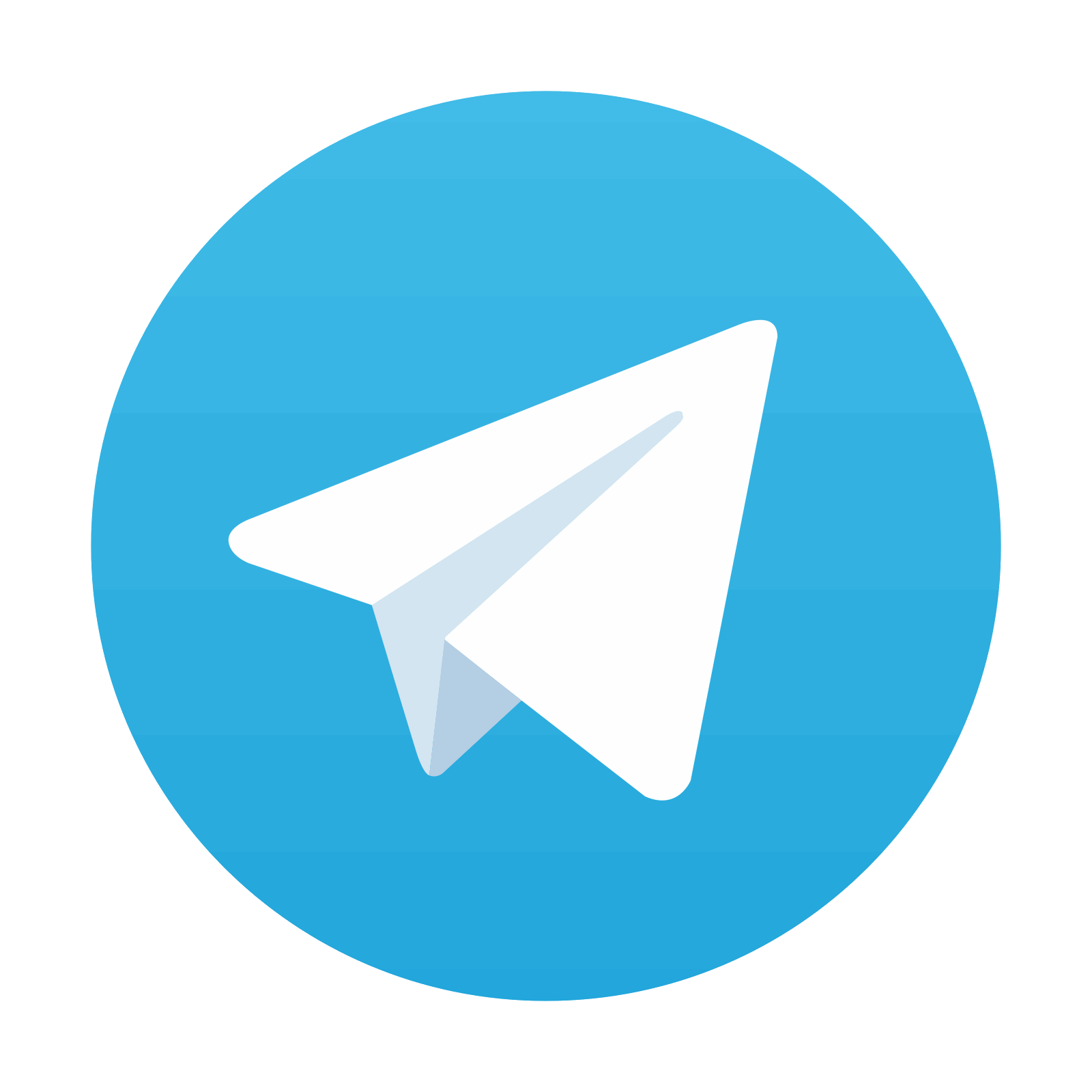
Stay updated, free articles. Join our Telegram channel

Full access? Get Clinical Tree
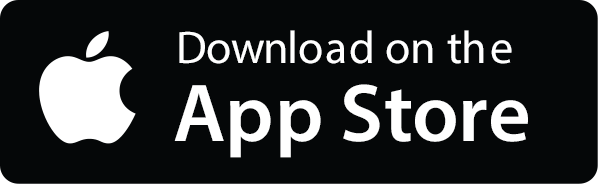
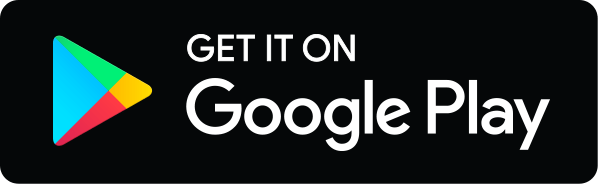
