Fig. 12.1
Comparison of T2* with SWI in the detection of hemorrhagic lesions. A 45-year-old man fell down from a staircase and then visited ER with a severe headache. Both T2* GRE and SWI detected hemorrhages on the right side of the inferior temporal lobe. However, in a small area with mixed blood and edema, T2* GRE only detected edema (bright signal) and SWI detected hemorrhage (dark signal) (see arrow), which suggests methemoglobin at the acute stage
Quantitative Susceptibility Mapping of Bleeding and Blood Oxygenation
Despite its improved sensitivity in the detection of micro-hemorrhages, SWI is still a qualitative instead of quantitative approach. Theoretically, blood products at different stages, from deoxyhemoglobin, to extracellular methemoglobin and then hemosiderin, has different susceptibilities. As a natural extension, susceptibility-weighted imaging and mapping (SWIM), also called quantitative susceptibility mapping (QSM), is the next generation of SWI development, which could quantify the susceptibility values of blood products. Ideally SWIM/QSM could have the following potential usage in mTBI:
(a)
Differentiating new from old hemorrhage. Fresh blood at the acute stage of injury with deoxyhemoglobin has relatively low susceptibility, subacute blood with extracellular methemoglobin has relatively higher susceptibility, and chronic blood with hemosiderin has the highest susceptibility. Therefore, the susceptibility signal intensity may differentiate the stage of the bleeding.
(b)
Improving the detection of micro-hemorrhages. One difficulty in clinical radiology is to differentiate microbleed from veins, especially at the cortical surface, where the brain is enriched with venous structure and therefore prone to bleeding. A cross section of a cortical vein or pial vein could be easily misinterpreted as a micro-hemorrhage, and a micro-hemorrhage buried in the surrounding venous structure could be easily overlooked as a vein. By using minimal intensity projection (mIP), the dark venous signal on adjacent slices can be projected onto one image to see the continuity of the venous structure. Even so, a radiologist still needs to navigate through several images to check the morphology, including the continuity of the black dots and smoothness of a potential vessel wall, to verify a vein or bleed. With the addition of SWIM, chronic bleed (hemosiderin) will likely have higher susceptibility than the surrounding veins. The high signal intensity would easily distinguish itself from the veins (see Fig. 12.2 for a chronic bleeding case).
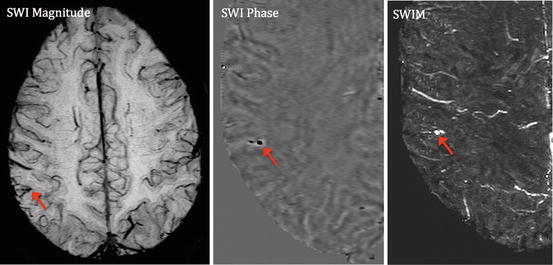
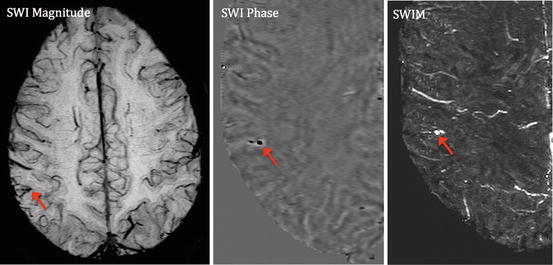
Fig. 12.2
Quantitative susceptibility mapping of chronic bleeding after mTBI. A chronic mTBI case with a microbleed embedded in a pial vein on the cortical surface. The SWI phase image demonstrates that its shape looks more like a pial vein. The SWIM result demonstrates very high susceptibility value (2,200 ppm), which is much higher than a normal vein (usually around 200 to 300). This is suggestive of chronic bleeding in the form of hemosiderin rather than a venous structure
Another challenge in clinical radiology is to differentiate hemorrhage from calcification. Empirically, calcification tends to happen in a symmetrical manner in the basal ganglia and choroid plexus in the lateral ventricles. However, it could be misinterpreted as a bleed if it is in brain parenchyma because both calcification and hemorrhage have low signal on SWI images. From the physics point of view, hemorrhage and calcification have opposite susceptibility behaviors: hemorrhage is paramagnetic and shows high signal on SWIM images in contrast with the low signal of calcification, which is diamagnetic. With SWIM, they can be easily differentiated [35].
(c)
Quantifying blood oxygenation. After injury, the damaged cerebral vascular structure may present with reduced cerebral blood flow, venous stenosis, resulting from even venous thrombosis. Both animal data and recently reported clinical data identify venous thrombosis after TBI [36]. There is even an ongoing clinical trial for venous thrombolysis after TBI [36]. The reduced blood oxygenation or thrombosis will manifest as an increased susceptibility signal on SWIM images. We have developed SWIM to quantify susceptibility as a means to estimate blood oxygenation in major veins [37]. With SWIM, researchers could quantify the degree and extent of venous blood oxygenation. See Fig. 12.3 as a sample to show quantitative susceptibility signal of a transmedullary vein after blast-induced TBI.
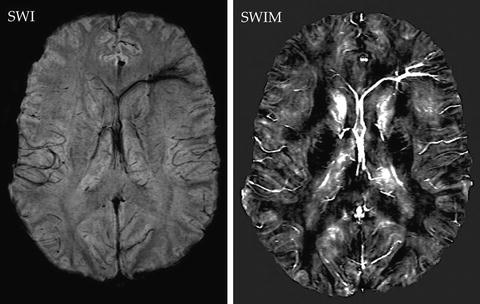
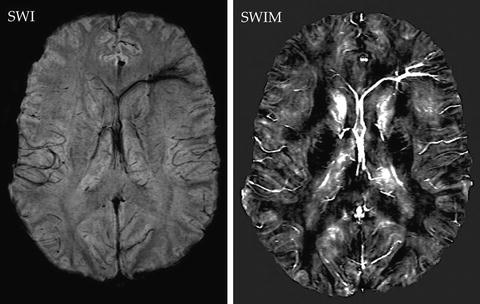
Fig. 12.3
Decreased venous blood oxygenation in septal vein after blast-induced TBI. SWI image (left) shows abnormal signal on left anterior septal vein but short of quantitation. SWIM (right) shows the decreased blood oxygenation on the vein with signal intensity linearly proportional to the concentration of deoxyhemoglobin
(d)
Quantify disease progression or treatment effect. The availability of a quantitative signal would allow researchers to track the progression of blood product transformation over time, which could be a biomarker for disease progression. This could be very useful for bleeding and re-bleeding cases. Chronically, the hemosiderin deposit after hemorrhage may stay in the brain tissue for a long time and become toxic to the brain by inducing edema [38] or hydrocephalus [39, 40]. Iron chelation drug treatment could be a treatment to remove these iron deposits and is being reported in correlation with outcome improvement in animal models of intracerebral hemorrhage [41, 42]. The availability of SWIM will certainly provide a means for in vivo assessment of iron chelation treatment.
In summary, there is still a need to investigate TVI in a large number of mTBI patients by using SWI/SWIM. This may represent a promising direction for mTBI research in the coming years.
Diffusion Tensor Imaging of Axonal Injury
Diffusion imaging sequences are sensitive to TAI secondary to stretch and shear forces. DTI measures the bulk motion of water molecular diffusion in biological tissues. It is most useful when diffusion is anisotropic, i.e., when diffusion is not equivalent in all directions, such as in skeletal muscle or axons in the white matter of the CNS. Histological studies have validated DTI’s sensitivity to brain injury in both focal injury [43] and DAI [44] models. Two parameters derived from DTI, the apparent diffusion coefficient (ADC) and fractional anisotropy (FA) [45, 46], have been extensively studied in TBI. ADC is an estimate of the average magnitude of water movement in a voxel (regardless of direction), while FA is an index of the directional nonuniformity, or anisotropy, of water diffusion within a voxel.
FA has been used to detect alterations in directional diffusion resulting from tissue damage. FA in white matter is highest when fibers are long (relative to voxel dimension) and oriented uniformly (collinear) within a voxel and lowest when fibers are not collinear (e.g., “crossing fibers”) or have been damaged. When axons are injured, as in acceleration/deceleration injuries (such as MVCs), diffusion anisotropy typically decreases. Loss of diffusion anisotropy is the result of a number of axonal changes after injury including: (1) increased permeability of the axonal membrane; (2) swelling of axons; (3) decreased diffusion in the axial (long axis) direction; and (4) degeneration and loss of axons in the chronic stage. In general, any pathological alteration of white matter fibers will result in FA decrease, because one or more of these axonal changes occur in disorders of white matter. Not surprisingly, most clinical studies of moderate and severe TBI have shown FA to be more sensitive than ADC to traumatic injury. On the other hand, ADC, FA, and directionally selective diffusivities (principal, intermediate, and minor components of diffusion) can help to better characterize brain injury pathologies. Trace and mean diffusivity (MD) are two other measurements similar to ADC and vary similarly. Changes in FA in association with ADC changes can differentiate the type of edema. For example, in the acute stage, decreased FA in association with increased ADC suggests vasogenic edema, while increased FA in association with decreased ADC suggests cytotoxic edema. Decreased FA in association with decreased ADC and decreased longitudinal (parallel to the long axis of the axons) water diffusivity suggests axonal transport failure as occurs in degenerative neurological diseases such as in ALS.
Regarding the location of brain lesions detected by DTI, Niogi and Mukherjee [47] summarized that the frontal association pathways, including anterior corona radiata, uncinate fasciculus, superior longitudinal fasciculus, and genu of corpus callosum (CC), are the mostly frequently injured WM structures in mTBI patients. Single subject results were not reported but it can be assumed that significant interindividual variability for location and extent of WM injury exists due to varying injury mechanisms (and forces) and biological (neural and non-neural) differences across patients.
Imaging at Different Pathological Stages
Interestingly, despite the higher incidence of milder TBI compared with more severe TBI in western countries, there are many fewer mTBI imaging studies reported in the literature. One reason is the fact that recruitment of mTBI patients is more difficult, since they are typically outpatients. Another reason is the common conception that mTBI is a transient problem from which virtually all who are afflicted will recover fully. Therefore, some question the clinical importance of studying mTBI. Certainly, insurance reimbursement for MRI scanning of mTBI is rare and even rarer in the acute setting, so that “adding on research sequences” is not possible.
With these limitations in mind, a growing literature on DTI in TBI has begun to address the DTI findings at different stages.
Acute Stage
There are currently conflictive findings for FA and ADC in mTBI at the acute stage. Chu et al. [48] and Wilde et al. [49, 50] from Baylor College of Medicine scanned 10–12 adolescents with mTBI within 6 days of injury and reported increased FA, reduced ADC, and reduced radial (short axis) diffusivity in WM regions and the left thalamus. Similarly, Bazarian et al. [51] studied six mTBI patients within 72 h and reported increased FA in the posterior CC and reduced ADC in the anterior limb of the internal capsule (IC). Similar to Chu et al. [48] and Wilde et al. [49, 50], Mayer et al. [52] studied 22 mTBI patients within 12 days of injury and reported increased FA and reduced radial diffusivity in the CC and left hemisphere tracts.
Inglese et al. [53], in contradiction to Chu et al. [48], Wilde et al. [49, 50], Bazarian et al. [51], and Mayer et al. [52], found reduced FA in the splenium of CC and posterior limb of IC in 20 mTBI patients imaged up to 10 days after injury (mean = 4 days). Manually drawn regions of interest were used to assess the genu and splenium of the CC, the centrum semiovale, and the posterior limb IC bilaterally. In the same line as Inglese et al., Arfanakis and colleagues [54] studied a handful of mTBI patients at the acute stage and reported FA decrease in major WM tracts.
Most recent data on sports injury further reported that DTI FA changes could be bidirectional, which means the coexistence of both increased and decreased FA in different locations of the same brain [55]. Kou et al. also demonstrated this bidirectional change of FA in mTBI patients in the acute setting (within 24 h after injury) [56].
Subacute Stage
Many studies reported reduced FA and/or increased diffusivity, i.e., ADC, trace or mean diffusivity (MD), at this stage. Messé et al. [57] studied 23 mTBI patients at the subacute stage and found significantly increased MD in mTBI patients with poor outcome [57]. Lipton et al. [58] scanned 20 mTBI patients in the subacute stage and demonstrated reduced FA and increased MD in frontal subcortical WM. Miles et al. [59] studied 17 acute and subacute mTBI patients and found reduced FA and increased MD. All of these studies at the subacute stage reported a similar profile of DTI measures except for different locations of injury.
Chronic Stage
Three studies report either reduced FA or increased diffusivity or both and one study reported increased FA at this stage. Cubon et al. [60] studied ten collegiate athletes with concussion at the chronic stage and found increased MD in left WM tracts, internal capsule, and thalamic acoustic radiations. Lo et al. studied ten mTBI patients and reported reduced FA and increased ADC in the left genu of the CC and increased FA in internal capsule bilaterally [61]. Niogi et al. [62] studied 43 chronic mTBI patients and reported reduced FA in a large number of WM tracts. In contrast, Michael Lipton et al. [63] reported bidirectional changes (both increase and decrease) of FA in chronic mTBI patients.
All DTI studies of moderate to severe TBI patients [18, 64–66] and most subacute/chronic mTBI patients [53, 62, 67–69] report increased FA which are correlated with clinical or neuropsychological measures. However, the seemingly contradictory findings in mTBI in the acute stage do not fit this pattern. It has been suggested that decreased FA may reflect vasogenic edema at the acute stage. In contrast, increased FA acutely may reflect cytotoxic edema [50], which would shunt extracellular fluid into swollen cells. This could have the effect of reducing inter-axonal free water and therefore increasing anisotropy. The bidirectional changes of FA in recent studies further suggest the coexistence of both types of edema and clinical data could be much more complicated than we thought [55, 56].
Longitudinal Studies
Only a few investigators have followed FA over time in the same patients. Sidaros et al. [70] studied 23 severe TBI patients at 8 weeks and again at 12 months and found that partial recovery of initially decreased FA values in the internal capsule and centrum semiovale predicted a favorable outcome. Kumar et al. [71] studied 16 moderate to severe TBI patients at 2 weeks or less, 6 months, and 2 years and found persistently reduced FA except in the genu of the corpus callosum where there was partial normalization by 2 years. Recently, two studies by Mayer et al. [52] and Rutgers et al. [68] reported that a normalizing FA may reflect recovery. This evidence suggests that a systematic investigation of a large number of mTBI patients at acute, subacute, and chronic stages is warranted to reveal the evolution of pathophysiology in mTBI.
Correlation Between DTI-Derived WM Injury Topography and Neuropsychological Deficits
Mild TBI patients often develop a constellation of physical, cognitive, and emotional symptoms that are collectively known as PCS. In terms of neurocognitive symptoms, there are four key domains implicated in chronic neuropsychological impairment after mTBI. These domains include: (1) higher-order attention, (2) executive function, (3) episodic memory, and (4) speed of information processing. To date, several studies have demonstrated typical mTBI cognitive profiles and association with DTI findings. Niogi and Mukherjee summarized the topographic and neurocognitive deficits [47].
Damage to the frontal WM has been reported to be associated with impaired executive function. Lipton et al. [58] studied 20 acute to subacute patients and reported that reduced FA in WM of dorsolateral prefrontal cortex (DLPRC) is correlated with worse executive function.
Frontal WM injury is also associated with attention deficit. Niogi et al. [72] reported that reduced FA in the left anterior corona radiata is correlated with attention control in chronic mild TBI patients.
Injury at the temporal WM tracts or cingulum bundle may cause memory problems. Niogi et al. reported that reduced FA in the uncinate fasciculus correlated with memory performance [72]. Wu et al. [49] reported that an FA measure of left cingulum bundle correlated with delayed recall.
Injury of the callosal fibers has been reported to be associated with PCS scores. Wilde et al. [50] studied ten adolescent mTBI patients in the acute stage and reported that increased FA and decreased ADC and MD in corpus callosum is correlated with patients’ PCS score. Bazarian et al. [51] studied six mild TBI patients in the acute stage and reported a lower mean trace in the left anterior IC and a higher FA in the posterior CC. FA values correlated with patients’ 72 h PCS score and visual motor speed and impulse control.
The overall burden or extent of WM injury is associated with both speed of information processing and overall functional outcome. Niogi et al. [62] studied 34 subacute to chronic mTBI patients and reported that FA decreased in several WM regions, including anterior corona radiata, uncinate fasciculus, CC genu, and cingulum bundle. They demonstrated that the number of damaged WM regions is correlated with patient’s reaction time. Miles et al. [59] studied 17 mTBI patients at the acute stage and followed them up to 6 months after injury. They reported that, at the acute stage, the increased mean diffusivity (MD) in the central semiovale, the genu and splenium of CC, and the posterior limb of IC tended to correlate with a patient’s response speed at 6 months after injury. Regarding the overall outcome, Messé et al. [57] divided mTBI patients into two outcome groups: poor outcome (PO) vs. good outcome (GO). PO patients showed significantly higher mean diffusivity (MD) values than both controls and GO patients in the corpus callosum, the right anterior thalamic radiations, the superior longitudinal fasciculus, the inferior longitudinal fasciculus, and the fronto-occipital fasciculus bilaterally.
Interestingly, injury or reduced blood supply in the thalamus, which is the relay station of neuronal pathways, may cause a constellation of symptoms in the speed of information processing, memory, verbal, and executive function. Grossman et al. studied 22 subacute to chronic mTBI patients by using diffusion kurtosis imaging, which is a more advanced form of diffusion analysis, and demonstrated that overall cognitive impairment is associated with the diffusion measurement in the thalamus and internal capsule [73]. This work is along the same line of a perfusion study by the same group, which demonstrated that reduced blood flow in the thalamus correlated with patient’s overall neurocognitive function [74]. See Fig. 12.4 as an example of axonal injury. In summary, significant progress has been made by researchers in recent years regarding the prognostic value of DTI in the form of FA for mTBI patients’ neurocognitive outcome. However, more and more evidence suggests that a tensor model may not be the ideal approach to the complicated pathophysiological process in TBI. Particularly, despite its high sensitivity, DTI suffers low specificity to trauma. Numerous medical reasons could cause water diffusivity changes and lead to changed FA; examples include neurodegenerative diseases, stroke, cancer, multiple sclerosis, hypertension, and diabetes, to name a few. Any preexisting conditions in the brain could affect the diffusivity and FA changes. Conflicting data on FA changes at the acute stage suggest that the field needs a better approach to characterizing the injury pathology than the current DTI model. This approach should be more specific to a TBI pathological process. It could be used to evaluate the neural basis of patients’ injury to recovery process in longitudinal studies over a large number of patients.
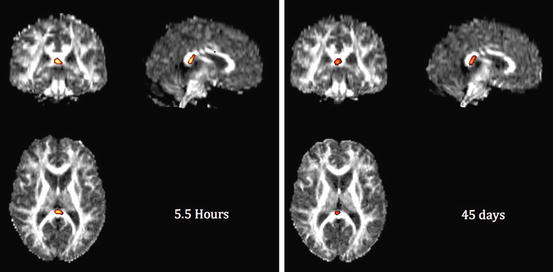
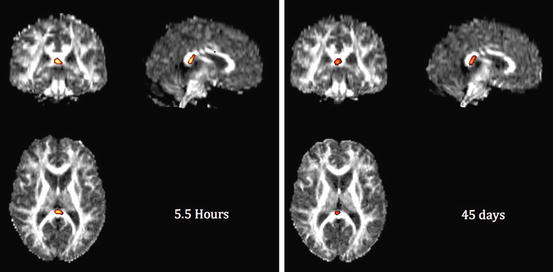
Fig. 12.4
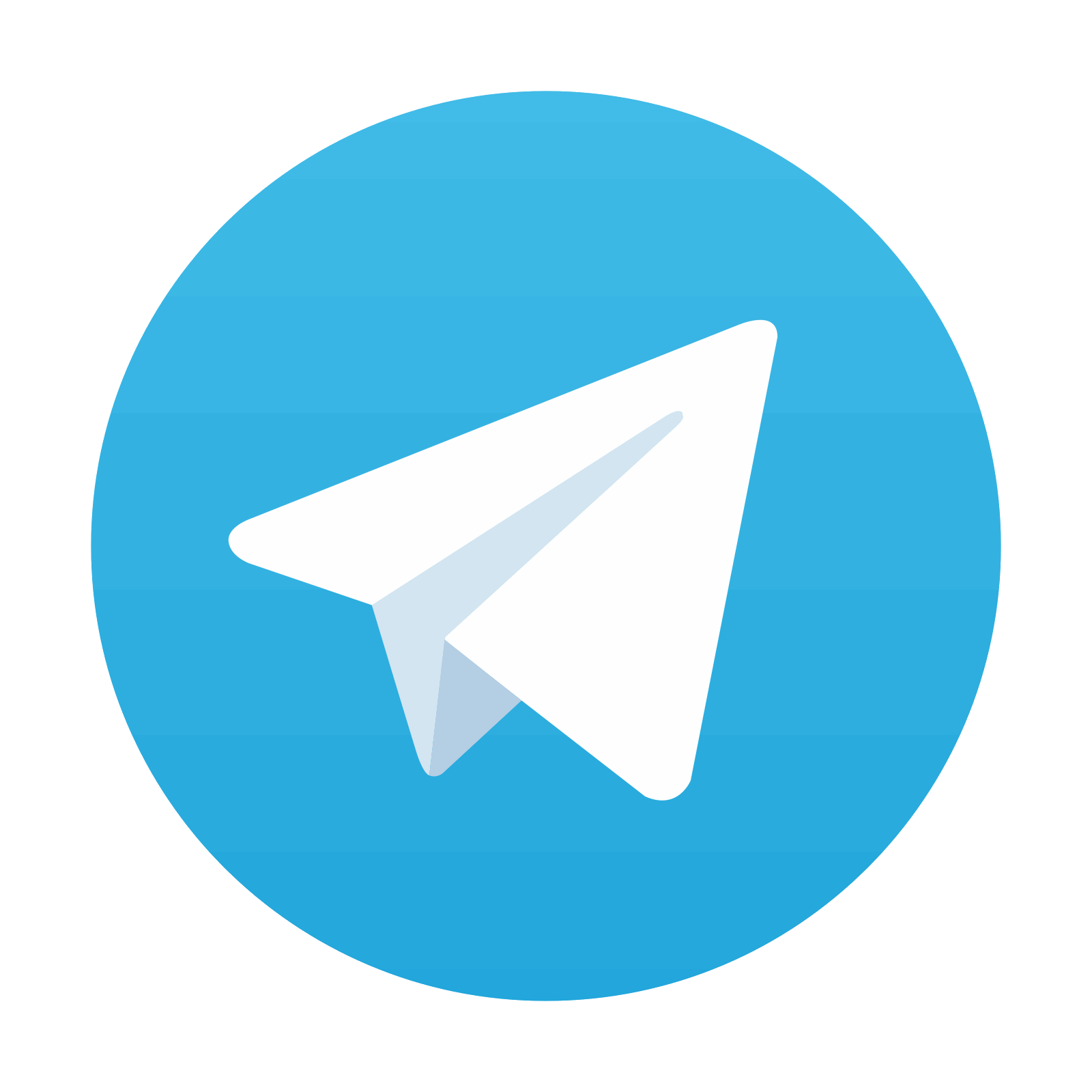
DTI analysis of an mTBI patient scanned on the day of injury (5.5 h after injury) and 6 weeks later. A 31-year-old male fell 10 ft. off a ladder striking the back of his head with brief loss of consciousness and confusion. The patient developed persistent mild cognitive symptoms after 6 weeks of injury. Note the same location of reduced FA in left corona radiata. Global WM FA mean was within normal
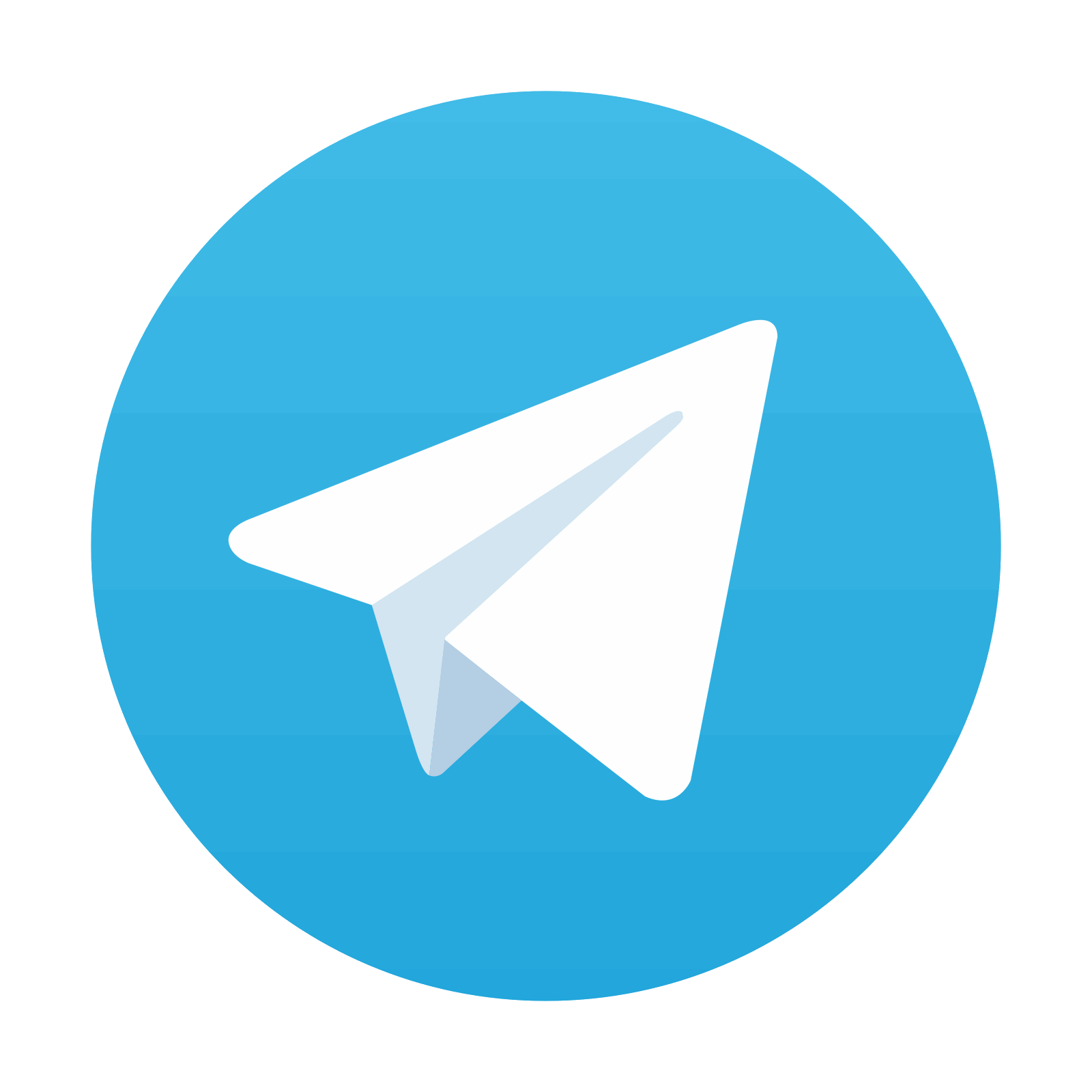
Stay updated, free articles. Join our Telegram channel

Full access? Get Clinical Tree
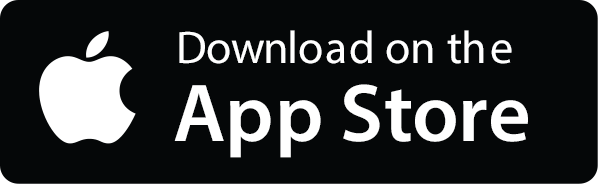
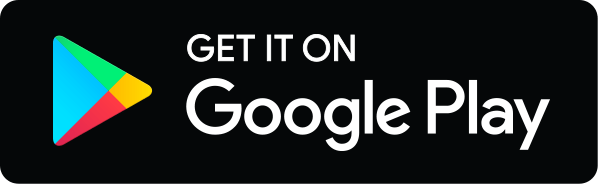
