Road events
Track events
• Road race (men and women)
• Individual time trial (men and women)
• Handcycling team relay (men and women, mixed event)
• Tandem sprint (men and women)
• Team sprint (men and women, mixed event)
• 500 m time trial (men and women) or 1 km time trial (men and women)
• Individual pursuit (men and women)
• Scratch race (men and women)
The Paralympic Movement website (Paralympic.org) details the different classifications of para-athletes that may compete in adaptive cycling. The four main classes of adaptive cycling include handcycling, tricycling, bicycling, and tandem cycling depending on the degree and type of impairments. Of the ten eligible impairments for the Paralympics, eight qualify for inclusion in adaptive cycling: impaired muscle power, impaired passive range of movement, limb deficiency, leg length difference, athetosis, hypertonia, ataxia, and visual impairment. Those with intellectual impairment or short stature are excluded from competitive adaptive cycling.
Handcycling has five classes (H1–H5) (Table 10.2) with lower numbers indicative of more severe impairment(s). H1 athletes are tetraplegic (C6 or above) and generally have severe upper limb impairment with no ability to use their trunk or leg. H2 athletes are also tetraplegic (C7/C8) with severe athetosis, ataxia, and/or dystonia. H3 athletes may have spinal cord lesions anywhere from T1–T10 (or equivalent injury) with limited trunk stability. H4 athletes will have impairment from T11 down, with normal or almost normal trunk stability, but be unable to kneel. Those competing in classes H1–H4 compete in a reclined position and compete on a recumbent handcycle, whereas H5 class athletes are paraplegics (T11 or below) and amputees who distinguish themselves from H1–H4 by being able to kneel and compete on a kneeling handcycle. Those competing in classes H1–H4 compete in a reclined position, whereas H5 competitors are capable of sitting on their knees and may use both their arm and trunk. Examples of H1–H4 athletes include those with spinal cord injury, cerebral palsy, or multiple sclerosis. H5 athletes may have leg amputation or congenital limb deficiency, paraplegia, or ataxia [4].
Table 10.2
Classification of adaptive cyclists
Handcycling (H1–H5) | H1 | Tetraplegic (C6 or above) |
H2 | Tetraplegic (C7/C8) | |
H3 | T1–T10 injury | |
H4 | T11 down—reclining | |
H5 | T11 down—kneeling | |
Tricycling (T1–T2) | T1 | Severe athetosis, ataxia, dystonia |
T2 | Moderate athetosis, ataxia, dystonia | |
Bicycling (C1–C5) | C1 | Grade 3 spasticity |
C2 | Grade 2 spasticity | |
C3 | Less spasticity in upper limb (grade 1) | |
C4 | Grade 1 spasticity | |
C5 | Minimal impairment | |
Tandem cycling (B1–B3) (for visually impaired) | B1 | No sight |
B2 | Visual acuity 2/60, visual field <5° | |
B3 | Visual acuity between 2/60 and 6/60, 5° < visual field angle <20° |
Tricycling has two classes (T1–T2) (Table 10.2), again with the lower number corresponding to a higher degree of impairment. Adaptive cyclists will use a tricycle if their coordination or balance is affected enough to require the tricycle for stability while riding. T1 tricyclists have severe athetosis, ataxia, or dystonia and generally with grade 3 spasticity or higher. T2 tricyclists have more moderate athetosis, ataxia, or dystonia with more fluent movements, grade 2 spasticity for hemiplegic and quadriplegic patients, and grade 3 spasticity for diplegic athletes.
Adaptive cyclists capable of riding a traditional upright bicycle will compete in one of five classes (C1–C5) (Table 10.2), once again with lower numbers corresponding to the highest degree of impairment. C1 cyclists have grade 3 spasticity in upper and/or lower limbs with poor strength in the trunk and/or extremities and can have single or double amputations. C2 cyclists have grade 2 spasticity throughout with greater lower limb involvement. C3 cyclists will have less spasticity in the upper limb (grade 1) versus the lower limb (grade 2) and with less athetosis, ataxia, or dystonia than C1 or C2 cyclists. C4 cyclists generally have grade 1 spasticity with the lower limb more involved and mild to moderate athetosis, ataxia, or dystonia. C5 cyclists have minimal impairment such as monoplegia spasticity with clear neurologic signs or single amputation. Classes C1–C4 may be amputees, whereas C5 athletes may not and have minimal impairments compared to the other classes. Examples of adaptive bicyclists include those with amputations, impaired muscle power or range of motion, and coordination deficits.
Tandem cyclists are typically visually impaired and ride with a sighted “pilot” cycler to their front. Visual impairments are classified from B1–B3 (Table 10.2), and they all typically compete together in the same event(s). B1 athletes have no sight. B2 athletes have the ability to recognize the shape of a hand up to visual acuity of 2/60 and/or visual field of less than 5°. B3 athletes have visual acuity of 2/60 up to 6/60 and/or visual field of more than 5° and less than 20°.
Adaptive Cycle Design and Technology
Initially designed for impaired military veterans after World War I to help them navigate more difficult terrain, the adaptive handcycle has evolved into a popular mode of cycle that allows adaptive cyclists to participate in cycling both recreationally and competitively. Four basic designs of handcycles exist: (1) an arm-crank add-on unit which attaches to the athlete’s own wheelchair, (2) upright arm-crank units where the athlete is positioned similar to that of being in their own wheelchair, (3) cycles where athletes kneel with their torso straight up or leaning forward over the cranks, and (4) recumbent cycles in which the athlete lies supine while manning cranks above their chest. The main two designs used in the Paralympics are the latter “kneeling” and “recumbent” handcycles (Fig. 10.1). Road racing handcycles are usually three-wheeled with the front wheel chain controlled and operated by the adaptive athlete using their upper limb(s) and/or torso, contingent on their disability. Handcycles are more efficiently driven than standard pushrim wheelchairs, making them more ideal for road racing [2].


Fig. 10.1
Recumbent and kneeling position handcycles (adapted from Cooper and De Luigi, PM R 2014, S37)
Tricycle and bicycle design and technology generally echo the standard design for able-bodied individuals with specific, limited adaptations (i.e., attachment for lower limb prosthesis to foot pedal, etc.). General considerations with regard to bicycle and tricycle design include the cyclist’s body segments and bicycle/tricycle components, as well as the interface points of the body with the cycle (i.e., the perineum contact on the seat, the handgrip point on the handlebars, and shoe/pedal interface), which can all affect the upper and lower body reach of the cyclist. Changes in rider body position will directly affect aerodynamics and frontal surface area resistance. UCI regulations also only allow a standard diamond-shaped frame for upright bicycles during competition with various more aerodynamically designed frames outlawed [6].
A tandem cycle will generally take the form of a “two-seat” bicycle with the sighted “pilot” cycler seated in the back behind the visually impaired adaptive cyclist. Mechanical design of the tandem cycle must enable the riders to be as close as possible in order to optimize aerodynamics. The pilot controls steering, gear changing, and tactics, whereas the visually impaired cyclist must instantly react to cadence increases as well as changes from standing to seated positions on verbal cue. The visually impaired cyclist must also be able to remain calm and composed during sudden movements of the tandem cycle and changes in road slope or other race conditions [6].
Important variables when designing any adaptive cycle include saddle height, saddle setback, saddle tilt, the length of handlebar/hand control reach from the saddle, the handlebar height drop from the seat, crank length, and the saddle tube angle. Changes in cycle setup can influence trunk angle, which in turn can influence muscle recruitment and intermuscular dynamics throughout the body. Ideal adjustment of these variables in relation to one another will optimize power output for and race performance by the adaptive cyclist [6].
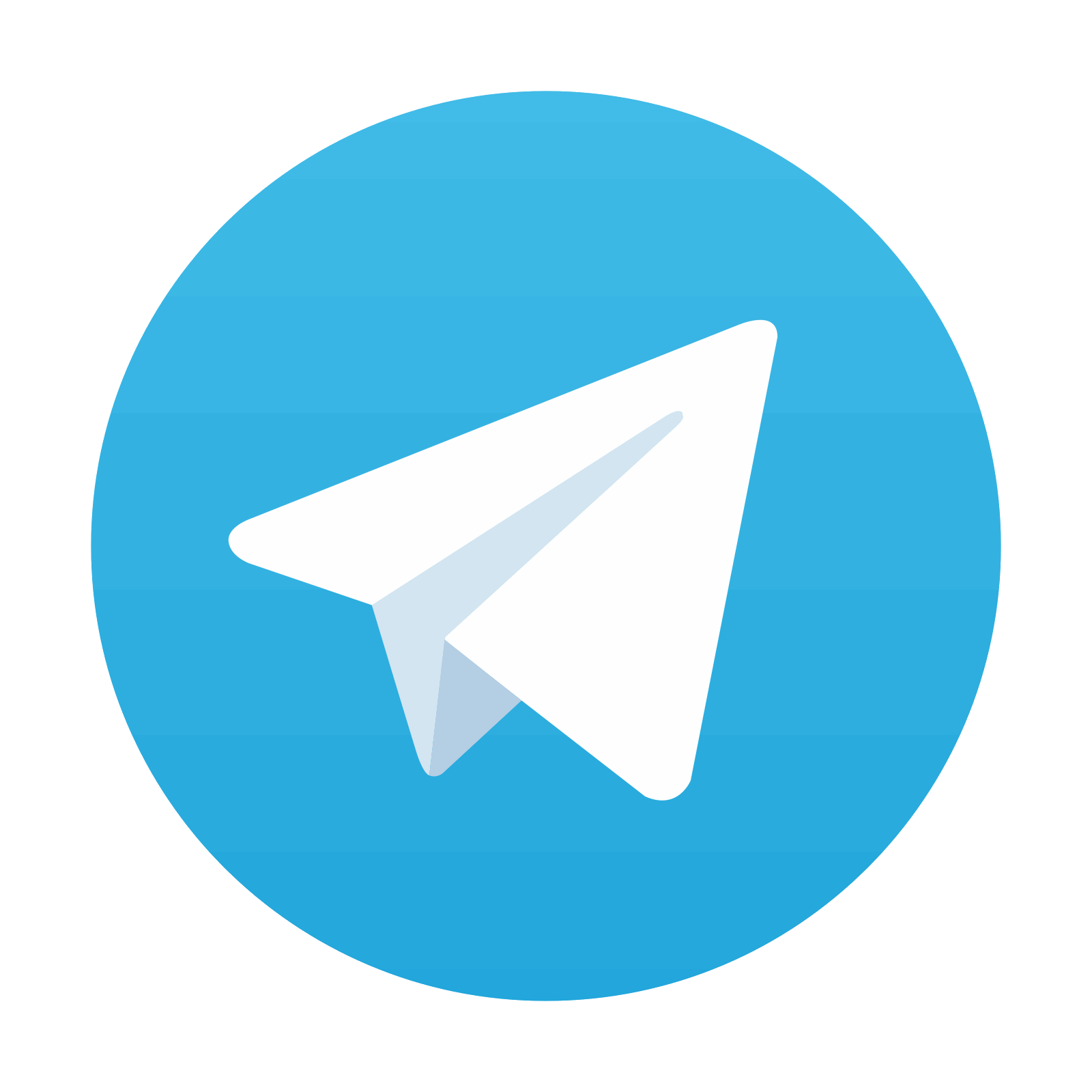
Stay updated, free articles. Join our Telegram channel

Full access? Get Clinical Tree
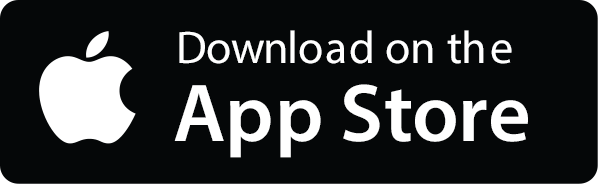
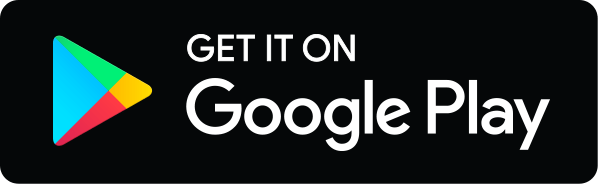