1 Acute Trauma and Overuse Injuries: Essentials Postnatal skeletal development unfolds in three steps leading to ossification of the bone, between which the bone undergoes periods of longitudinal growth: • Development and growth of the ossification centers in the preformed cartilaginous epiphyses of the short and long tubular bones as well as in the carpal and tarsal bones. • Development and growth of the apophyseal ossification centers. • Bony epiphyseal closure. Only two epiphyseal ossification centers are present in the mature newborn: the distal femoral epiphyseal ossification center and usually also the proximal tibial epiphyseal ossification center. The order in which other ossification centers become visible is age dependent. Once an ossification center has appeared, ossification then progresses toward the margins of the cartilaginous precursor. The physis or growth plate has a decisive role in the maturation process. Not only is it responsible for eventual height and body mass, it also contributes to resilience and elasticity of the skeleton during growth. It consists of five zones separating the secondary ossification center (the so called chondroepiphysis) from the metaphysis. The growth plate is especially prone to injury (see Chapter 1.3). The cartilaginous plate between the bony epiphysis and the shaft is obliterated and replaced by bone by the time the epiphysis closes; only the joint cartilage remains as a narrow cartilaginous layer. Apophyseal ossification centers appear shortly before or during puberty. Fusion of the apophyses with the bones starts after puberty and is usually complete by the age of 25 years. The time of appearance of the ossification centers, their growth, and fusion of the epiphysis and apophysis with the bone are constant within a certain range of variation, allowing assessment of skeletal maturity and skeletal age. During postnatal skeletal development, remodeling of the bone marrow proceeds in parallel with the ossification steps. The medullary cavity of the fetus is still filled with hematopoietic red marrow. Shortly before birth, conversion to fat marrow begins in the limbs in a centripetal direction, starting in the distal phalanges. In early adulthood, hematopoietic marrow is still mainly present in the skull, the sternum, the ribs, the pelvis, and the proximal humerus and femur; fat marrow is found in the rest of the skeleton (Chapter 5). Fig. 1.1 MRI of the knee joint of a 5-year-old child. (a) Fat marrow signal in the ossification nucleus of the epiphysis. On the T1W image, the signal intensity in the metaphysis is intermediate between fat and muscle, the classic appearance of red bone marrow. (b) On the PDW fat-saturated image, it is possible to differentiate between epiphyseal cartilage and articular cartilage. It is physiologic for epiphyseal cartilage to demonstrate somewhat heterogeneous signal intensity. The ossification centers within the preformed cartilaginous epiphyses and apophyses can exhibit numerous anatomical variations during skeletal growth; for example, two or several ossification nuclei may later fuse to form a single ossification center. During this phase, the ossification centers often demonstrate irregular margins, fragmentation and irregular mineralization on radiographs ( Among frequently encountered epiphyseal and apophyseal “variants” are the following: • Cone-shaped epiphyses of the bones of the hand are often associated with disturbances of endochondral growth of the affected phalanges ( • Pseudoepiphyses or atypical epiphyses occur in healthy children or more often in children with systemic abnormalities of skeletal development in the proximal metacarpals II–IV and the distal metacarpal I and are of no clinical significance. Fig. 1.2 Physiological irregular marginal contour (arrows) of the ossification center of the condylar epiphysis. Variant within the normal range. This most typically involves the posterior aspect of the femoral condyle. Fig. 1.3 Variant of the ossification nucleus of the distal femoral epiphysis. (a) Heterogeneous calcification of the epiphysis (arrow). (b) This MRI scan shows an area of low signal intensity in that region as a sign of increased sclerosis. Fig. 1.4 Ulnar epiphysis. This ulnar epiphysis consists of three ossification nuclei (arrow) which will later fuse together. Fig. 1.5 Cone-shaped epiphysis with brachymesophalangia (arrow), a normal variant with mild disturbance of endochondral bone growth. Fig. 1.6 A 6-year-old boy with focal epiphyseo–metaphyseal fusion. This fusion may be a pathologic variant of the cone-shaped epiphysis, which leads to in interference with growth. (a) The irregularity of the epiphyseal plate and the metaphysis on the radiograph suggest that fusion has taken place. (b) The MRI scan confirms a bony bar extending across the distal femoral physis. • Increased mineralization of the epiphyseal ossification nucleus may present in healthy children, most often in the distal phalanges. These ivory or marble-bone epiphyses ( The radiological appearance of ossification centers can vary, especially in the carpal and tarsal bones and may include: • The appearance of the ossification nuclei at different ages. • Irregular mineralization. • Fusion with an adjacent ossification nucleus. • The occasional appearance of accessory ossification nuclei. It is sometimes difficult to draw a line between a normal developmental variant and an alteration with clinical significance, especially when it is accompanied by transient local pain and/or a joint effusion. In these cases the presence of a developmental variant must be confirmed and a pathologic condition excluded. • Epiphyseal or apophyseal fracture in the presence of several atypical ossification centers ( • Avascular necrosis with irregular sclerosis and narrowing of the ossification nuclei ( • Osteochondritis dissecans or osteochondral fracture associated with an irregular peripheral contour of the epiphysis ( • Congenital disorder of skeletal development associated with an atypical appearance of the epiphysis ( Note Anatomical variants or disturbances in the radiological appearance of the epiphyses and apophyses can be found in all areas of the pediatric skeleton. These are dealt with in detail in the relevant literature, which should be accessed by the radiologist when an unfamiliar finding is encountered. A fracture (from the Latin: “frangere” = to break) is an interruption in the continuity of bone whether it involves cortical or trabecular bone. In everyday (surgical) language, the term is synonymous with radiographically visible fractures. However, there are fractures that are not revealed on conventional radiographs, although CT (computed tomography), MRI, and, at certain anatomical sites, even ultrasound can identify the fracture. These fractures are considered “radiographically occult.” The lowest level of traumatic bone damage is the purely trabecular fracture. It is only detectable on an MRI scan where fracture-related bone marrow edema (also known as “bone contusion” or “bone bruise”) will be evident. These lesions are indeed painful, but they generally heal spontaneously with rest and a short period of immobilization. Fig. 1.7 Increased sclerosis of the phalangeal epiphyses (known as ivory or marble-bone epiphysis), a normal variant. Fig. 1.9 Shell-like ossification nucleus posteriorly at the medial femoral condyle, a normal variant. Fig. 1.11 Irregular epiphyseal marginal contour. (a) Small subchondral lucency along the lateral condylar epiphysis. (b) Corresponding MRI shows a focus of irregular ossification. (c) Without evidence of a cartilage defect or a surrounding bone marrow reaction. Fig. 1.12 Comparison between Perthes’ disease and a normal developmental variant (Meyer’s dysplasia). (a) Perthes’ disease in the fragmentation stage. (b) Irregularly contoured epiphysis comprising several “fragments.” (c) Loss of signal of the epiphysis on the native T1W scan in a case of Perthes’ disease. (d) Normal fat signal intensity within the multiple ossification centers (= Meyer’s dysplasia). Traumatic fracture. Loading and overloading of a bone can occur directly through a blunt or penetrating injury, or indirectly. Indirect injury may result from traction, compression, shearing or torsional forces. Stress fractures. There are two types of stress fractures: fatigue fractures and insufficiency fractures. Fatigue fractures result from repeated overloading of normal bone and are distinguished from insufficiency fractures, which occur in abnormal bone that is more prone to injury, even from normal loading. The bone in the latter case is already weakened (usually by osteoporosis) and will fracture after a mild injury or with repetitive loading. Pathologic fracture. Pathologic fractures are fractures that arise as a result of preexisting cortical destruction when even an otherwise low-magnitude force is applied to the bone. These therefore represent a variant of insufficiency fracture. The most common causes are metastases, myeloma, and bone cysts. The morphology of the fracture reflects the direction, type, and extent of the forces acting upon the bone at the time of injury. In addition to the type of fracture, other important features include involvement of a joint and any associated subluxation or dislocation. Nondisplaced fracture (hairline fracture). This is a fine fracture line without any fragment displacement. It can be either incomplete or complete (passing through the whole bone). Impacted fracture. One fragment is wedged (firmly driven) into the other, e.g., in the hip. Depression fracture. This arises from the impact of a circumscribed force on the bone (e.g., on the skull). Avulsions of articular margins by opposing bones are a variation of depression fractures. Compression fracture. There is very little distinction between the terms “impacted fracture” and “compression fracture,” especially when the spine is involved. Avulsion fracture. These are fractures in which a small fragment of bone is torn away at the site of attachment of a ligament or tendon. Typical sites are the base of the fifth metatarsal bone, the tibial tubercle (patellar tendon), the superior pole of the patella (quadriceps tendon), the lesser trochanter (iliopsoas tendon), and the proximal phalanx of the first ray of the hand. These are more common in the skeletally immature patient. Dislocation. Dislocation involves complete loss of congruency between the articulating joint surfaces. An additional intra- or periarticular fracture may also be present. Subluxation is a joint malalignment where the articular surfaces still have some degree of contact with one another. Open fracture. In an open fracture, the soft tissue covering the bone has been violated. Fracture classification systems have practical purposes: • To determine the severity of the injury. • To select appropriate treatment. • To assess prognosis. • To have easy application and be easily reproducible. The classification systems for fractures in individual anatomical regions are dealt with in detail in Chapter 2. The AO Classification (AO Foundation, Switzerland; see http://aofoundation.org), is one of the best known and will be highlighted in the subsequent chapters. The AO classification distinguishes the following criteria: • Anatomical region (which bone is involved?). • Part (which segment of the bone is involved?). • Fracture type (letters are used to indicate either joint involvement or the complexity of shaft fractures; the digit after the letter defines the fracture more precisely). The AO-OTA classification combines the AO classification and the 1996 OTA classification. The OTA classification adopts the principles of the AO classification and completes it by coding some of the bones hitherto not classified by the AO system. The most important anatomical difference between the adult and the pediatric skeleton is the open growth plate in the skeletally immature patient. Growth plate injuries can result in disturbances of bone development, from premature physeal closure (arrest). The thicker and stronger periosteum found in the developing skeleton also allows the child to readily form callus, which can in some cases be very exuberant. The elasticity of pediatric bone allows for greater deformation before a complete fracture occurs and the strong periosteum often prevents any major displacement of the fracture fragments. Because the growth plate is weaker than the adjacent bone and supporting ligaments, epiphyseal separation occurs more frequently than do nonphyseal fractures or joint dislocations. From a physiological view, the skeleton of a child has the tendency for more rapid healing and excellent remodeling such that the development of nonunion is extremely uncommon. The normal classifications for adult fractures cannot usually be applied to the pediatric skeleton. Specific classifications, such as the PCCF–AO classification (see http://aofoundation.org), were accordingly developed for fractures in children. A simple classification according to location is usually sufficient for everyday clinical purposes: • Diaphyseal. • Metaphyseal. • Epiphyseal. • Combined injuries. Buckle or torus fracture. This is caused by compression in the region of the metaphysis. The cortex bulges out on one side. Changes on the opposite side of the bone are either absent or very subtle ( Greenstick fracture. This is an incomplete fracture that often involves only one side of the bone ( Bowing fracture. This refers to a plastic bending of the bone due to its increased elasticity in childhood, without the presence of a true fracture line ( Transition fracture. This involves injury to the growth plate that is in the process of closing ( Note The term transition fracture is not used in some countries (e.g., USA). This fracture is just described and should be considered a subtype of the Salter–Harris classification. The Salter–Harris classification system is most commonly used for describing injuries involving the growth plate ( • Type I: Epiphyseal separation. • Type II: Metaphyseal fracture with involvement of the growth plate. • Type III: Epiphyseal fracture with involvement of the growth plate. • Type IV: Fracture through the epiphysis and metaphysis, crossing the growth plate ( • Type V: Compression of the growth plate (crush injury) (see Fig. 1.15 Greenstick fracture in a 13-year-old boy (asymmetric cortical disruption). (a) Transverse sclerotic line in the trabecular bone with disruption of the lateral cortex. (b) The cortex is disrupted only on the palmar aspect. Fig. 1.16 Bowing fracture (arrows) of the ulna with subluxation of the radial head. There is no evidence of a fracture line. This is a case of a Monteggia-type injury (Chapter 2.8.3). Fig. 1.17 Salter–Harris III fracture in a partially closed physis. Fracture at the transition (arrow) between the closed and open epiphyseal plate. Battered-child syndrome (synonym: child abuse) encompasses all types of harm to a child, such as physical and mental traumatization, neglect, and sexual abuse. Usually neonates, babies, and infants have been subjected to physical abuse. Despite figures for suspected cases that are alarmingly high, only a small percentage of cases are brought to court, but the radiologist plays a decisive role in those cases in diagnosing physical abuse, which may be life-saving for the child. • Hematomas, abrasions, and burns of varying age and at unusual sites. • Inadequate history for the type of injury and delayed presentation of the child to the doctor. • Multiple skeletal injuries of varying age and fracture types that are “pathognomonic” for child abuse. • Subdural hematomas; cerebral contusions with or without skull fracture. • Patient age usually under 2 years. Skeletal injuries may present as single or multiple fractures in varying stages of healing and associated with marked periosteal reaction and callus formation. Older fractures are typically asymptomatic by the time the child presents. Fractures of the limbs present as metaphyseal and diaphyseal lesions in equal numbers. Brain injuries occur in the form of subdural hematomas and brain contusions as a result of direct force or hyperextension–hyperflexion injury with or without skull fracture. Shaking injuries result in subdural hematomas secondary to tears in bridging veins. Retinal hemorrhages are concomitant features in shaking injuries. If a head injury is suspected, ultrasound up to the first year of life and cCT (cranial CT) or MRI are the diagnostic methods of choice ( Rib fractures, especially posteriorly, are rare in early childhood. They are therefore very suspicious for physical abuse, as are fractures of the scapula, sternum, and spinous processes of the spine. Metaphyseal corner (chip) fractures are pathognomonic for the use of physical force. The radiological appearance comprises small rim fragments (“corner sign”), often with a fine, shell-like fragment along the metaphyseal ossification zone. This type of injury is caused by an abrupt jerk or twist of the limb. The periosteum and the insertion of the joint capsule in the region of the ossification zone are very firmly attached to the metaphysis, so that a sudden traction force will result in a metaphyseal fragment breaking away, along with the epiphysis ( Periosteal new bone formation—even without a visible fracture—is a common finding. In early childhood, the periosteum of the diaphysis is only loosely attached to the cortex, unlike the periosteum of the metaphysis. An injury can therefore easily produce a subperiosteal hematoma. In babies and infants, periosteal new bone formation becomes visible on radiographs between days 6 and 8, after injury ( Multiple bone lesions of varying age are indicative of abuse ( If child abuse is suspected, then a full skeletal survey is obligatory up to the age of 2 years, and each limb should be imaged in two planes. Fig. 1.20 Actual course of the fracture line in the case of a radiologically visible, metaphyseal corner or chip fracture. Fig. 1.24 Battered child. Same child as in Fig. 1.25 Battered child. Periosteal new bone formation encasing the diametaphyseal region after a distal metaphyseal fracture with a subperiosteal hematoma, 3 weeks after injury. If the suspicion of child abuse is substantiated, a complete diagnostic imaging examination is necessary for the protection of the child (for detailed review see the consensus recommendations by the American College of Radiology, 2011, referenced in the web material); this has both therapeutic and forensic implications. Injury to the central nervous system in particular requires prompt treatment, while findings of skeletal injury patterns indicative of abuse provide critical forensic evidence. • Obstetric and accidental fractures, e.g., sustained during very boisterous play with parents and relatives. • Neurological disorders, e.g., meningomyelocele, associated with fractures sustained during physiotherapy or otherwise. • Osteogenesis imperfecta, achondroplasia. • Metabolic disorders (rickets, Menkes’ syndrome). • Prostaglandin therapy. • Infantile cortical hyperostosis. Fractures of the articular surfaces are confined to the cartilage; those of the subchondral plate are confined to the cancellous subchondral bone. They run more or less parallel to the joint surface, and should be distinguished from fractures of the underlying bone which also extend into the joint (fractures with joint involvement). They are B or C fractures according to the AO classification (Chapter 1.2.3). Note Cartilage is more elastic than the subchondral trabecular structure of the bone. Subchondral bone therefore tends to fracture under abnormal force where cartilage rapidly recovers and “bounces back” to a normal state. As a rule, a distinction can be made between four stages of injury to articular surfaces ( Classification of fractures involving joint surfaces ( • Subchondral fractures. • Chondral fractures. • Osteochondral fractures. Location: The tibiotalar joint and the knee joint, including the patellofemoral compartment, are most commonly involved, but other locations, such as the femoral head and humeral head, may be affected. Note A radiographic examination has a relatively high rate of false-negative findings in fractures involving joint surfaces, despite imaging in more than two planes. In the case of the tibiotalar joint, for example, missed fractures are to be expected in up to 30% of cases. • Simple longitudinal or transverse fissures. • Stellate type. • Chondral flap. • Full-thickness cartilage defect secondary to a punched-out fragment with relatively sharply defined margins. Fig. 1.31 Osteochondral fracture of the proximal pole of the scaphoid as seen on CT arthrography. (a) Scapholunate (SL) dissociation. Focal defect of the articular cartilage at the proximal scaphoid pole. (b) There is a subchondral fracture (arrow) extending through the subchondral plate into the joint space. In the case of a subchondral injury, the articular cartilage is intact and the joint surface not depressed. Edemalike signal intensity is evident in the subchondral bone as a vague area of decreased signal on T1W (T1-weighted) images and is even more conspicuous as high signal intensity on fluid-sensitive (T2W) MR sequences, especially when fat saturation is applied. Associated fracture lines may be detected as linear or curvilinear areas of low signal intensity within the marrow edema ( A chondral fracture may be associated with a cartilage defect with relatively sharply defined margins secondary to a displaced fragment ( A very important type of chondral pathology known as delamination occurs when the hyaline cartilage separates from the subchondral bone plate. If there is an associated vertical fissure, a cartilage “flap” will occur, but if not, the delamination may not be detectable at arthroscopy unless the area is probed ( Abnormal signal intensity, with or without thickening of the cartilage, is sometimes the only indication of chondral pathology. In this case, chondromalacia is the most appropriate term. Osteoarthritis, rather than trauma, should be considered as an etiology for chondral abnormalities in older people, but the distinction is sometimes difficult. These injuries involve damage to the articular cartilage and subchondral bone and are often detected on MRI because of associated edema within the underlying marrow ( Fig. 1.33 Chondral fracture. (a) Focal cartilage defect. The absence of other signs of osteoarthritis suggests that this is related to an acute chondral fracture. (b) The cartilage fragment (arrow) is located in the lateral joint compartment. Fig. 1.32 Subchondral fracture of the lateral tibial plateau. (a) Hypointense trabecular fracture lines. (b) A fracture line is definable within the marrow edema. Fig. 1.35 Chondral delamination injury of tibial plateau. Note the high signal intensity extending between the cartilage and subchondral plate. Fig. 1.36 Serial scans of an osteochondral fracture of the tibial plateau. (a) Immediately after injury. (b) Five months later. The marrow edema is no longer evident but the depression of the articular surface persists. Fig. 1.38 Osteochondral fracture of the lateral femoral condyle. Only discrete step-off in the lateral cortex at the periphery of the articular surface (arrow). It is not fully understood how stress causes the bone to adapt to its mechanical function, but there are indications that this adaptation occurs primarily by way of microfractures. The initial response to increased stress is osteoclastic resorption. Osteoclastic resorption cavities are subsequently filled with lamellar bone over the course of weeks to months. In the meantime, if the excessive stresses continue, an imbalance between bone resorption and formation occurs and results in a temporary weakening of the bone. Periosteal and endosteal new bone formation provides some support for the temporarily weakened bone, but if the bone is not given sufficient rest, fatigue fractures of the trabecular and/or cortical bone may occur. Stress fractures are divided into two categories, depending on the initial state of the bone: Fatigue fractures. Bone density and structure are normal in these patients. A (reversible) weakening of the bone develops in response to increased activity. These are most common in athletes. Insufficiency fractures. In bone of abnormal density or structure, a fracture may develop even with only the stresses of normal daily activities. Osteoporosis is the most common etiology. A pathologic fracture (Chapter 1.5.3) is a type of insufficiency fracture that occurs in bone that has been weakened by underlying tumor. The most common type of insufficiency fracture is the osteoporotic vertebral compression fracture (Chapter 8.1.3). Note Insufficiency fractures of the pelvis are commonly the result of pelvic radiotherapy. • Classic fracture line (rare). • Lamellar to solid periosteal reaction as a sign of the repair process (see • Ill-defined line of density between cancellous bone and cortex (late stage; • Callus formation (late stage; Note Radiographic findings are often absent in the early stages of a stress or insufficiency fracture and may never develop if the causative activity is curtailed. Fig. 1.41 Insufficiency fracture of the tibial plateau in a case of disuse osteoporosis secondary to a distal femoral fracture. Fig. 1.43 Metatarsal insufficiency fracture with marked callus formation in an elderly patient with osteoporosis. Note If no fracture line is detectable on the MRI scan in cases of stress or insufficiency fractures, then this is referred to as a stress reaction ( Osteoid osteoma. This lesion typically demonstrates intense cortical/periosteal thickening on radiographs with a central, rounded radiolucency (that may only be detectable on CT or MR images). Evidence of a linear structure is absent in an osteoid osteoma. Other tumors rarely mimic a stress injury. Osteomyelitis. Osteomyelitis (acute, subacute, and chronic) can also produce significant periosteal new bone formation and cortical thickening and even a central lucency (Brodie’s abscess). An associated sinus tract and clinical findings of infection may help distinguish infection from stress injury or osteoid osteoma. Diabetic foot. A warm, swollen foot in a diabetic patient may be secondary to osteomyelitis or an insufficiency fracture. In both cases, radiography may reveal only osteopenia, while MRI demonstrates diffuse marrow edema within the bone. In the absence of an adjacent skin ulceration, cortical destruction, sinus tract or abscess, marrow edema alone (with or without a demonstrable fracture line) is more suggestive of a stress injury than of infection (Chapter 3.1). Fig. 1.46 Insufficiency fracture of the calcaneus in a patient with a history of steroid therapy for long-standing rheumatoid arthritis. (a) Sclerotic fracture line in the trabecular bone on a CT scan; the cortical bone is intact. (b) Hypointense fracture line and adjacent edema on a T1W scan. (c) Prominent enhancement is present around the fracture, which does not take up contrast. Fig. 1.47 Stress fracture of the left femur secondary to excessive sports activity. (a) Bone marrow edema and discrete periosteal edema. (b) The CT scan confirms the fracture (arrow) and excludes inflammatory or tumorous differential diagnoses.
1.1 Normal Skeletal Development, Variations, and Transitions to Pathologic Conditions
1.1.1 Normal Skeletal Development
Anatomy. Normal skeletal development involves growth and maturation of the epiphyseal and metaphyseal growth zones as well as changes involving the medullary cavity. Of all imaging modalities available, magnetic resonance imaging (MRI) is best suited for demonstrating these processes. It allows imaging of the epiphysis (which is formed entirely as a cartilaginous precursor or anlage), the conversion of epiphyseal cartilage into bone, the growth plate (= physis, epiphyseal plate), and the age-related conversion of the bone marrow (
Fig. 1.1).
1.1.2 Variations and Disturbances of Skeletal Development
Figs. 1.2 – 1.4).
Fig. 1.5). In contrast, cone-shaped epiphyses and disturbances of epiphyseal and metaphyseal growth of the long bones are always regarded as pathologic, likely the result of early epiphyseal and metaphyseal disturbances of circulation of various etiologies. A common feature is fusion between epiphysis and metaphysis (
Fig. 1.6).
Fig. 1.7), as they are called, must be distinguished from other skeletal disorders with increased sclerosis, such as osteopoikilosis.
1.1.3 Transitions to Pathologic States
Radiography. Epiphyseal or apophyseal variants can create difficulties in accurate diagnosis, and include:
Figs. 1.8 and
1.9).
Fig. 1.10).
Fig. 1.11).
Fig. 1.12).
US. Differentiation between a traumatic epiphyseal dislocation in infancy and a normal bone is possible by identifying the ossification center within the epiphyseal cartilage and its position relative to the ossified metaphysis. If a subchondral fracture, osteochondritis dissecans, or avascular necrosis is suspected in early to mid-childhood, an associated effusion—which is always present with these conditions—combined with a history of pain will prompt further diagnostic investigation. If no joint effusion is present, then true pathology is unlikely.
MRI. MRI findings in most cases allow a distinction to be made between traumatic injury and a variant or disturbance of ossification. Today, MRI is routinely used as a supplemental modality in cases with equivocal findings on ultrasound, and is the diagnostic method of choice for evaluating the bone marrow. Knowledge of age-related bone marrow conversion is a prerequisite for evaluating pathologic bone marrow findings (Chapter 5.1).
1.2 Fractures: Definition, Types, and Classifications
1.2.1 Definition and Classification
1.2.2 Fracture Types
Fig. 1.13 provides a simple overview of the most important fracture types.
Definitions
1.2.3 Classifications
References for Chapter 1.2.
1.3 Fractures in Children
1.3.1 Special Features of Fractures in Children
Special Fracture Types in Children
Fig. 1.14).
Fig. 1.15). The special feature of this fracture is that the periosteal sleeve remains intact.
Fig. 1.16).
Fig. 1.17; Chapter 2.14.1).
Growth Plate Injuries
Fig. 1.18):
Fig. 1.19).
Fig. 1.18).
1.3.2 Battered-Child Syndrome
Clinical presentation. Significant grounds for suspicion:
Fig. W1.1).
Radiography. Conventional radiograph is usually the first imaging examination.
Figs. 1.20 – 1.23).
Figs. 1.24 and
1.25).
Fig. 1.26). They are also of forensic significance since the radiological appearances can be used for estimating when the abuse occurred.
US. The extent of damage, especially in cases of metaphyseal corner fractures, is difficult if not impossible to visualize radiographically as the epiphyses are largely cartilaginous in early childhood. The position of the already ossified metaphysis relative to the cartilaginous epiphysis is recognizable on ultrasound, which allows the extent of damage and any possible epiphyseal dislocation to be assessed (see
Fig. 1.22). Joint effusions are easily detected. Additionally, subperiosteal hematomas can be detected at an early stage, prior to periosteal new bone formation.
NUC MED. A bone scan is a reliable screening method, even for clinically silent or uncertain bony lesions. Skull fractures and fractures older than 3 to 5 months cannot be detected with certainty, however.
MRI. In individual cases an MRI scan allows a better differentiation between Salter–Harris II and Salter–Harris IV fractures involving complex joints, such as the elbow. Its broader overview provides an advantage over ultrasound for preoperative planning. In addition, a whole-body MRI allows the detection of soft tissue lesions which may also be of forensic importance. Whole-body MRI is not associated with radiation exposure and therefore, as a screening method, has an advantage over skeletal survey radiography and radionuclide scanning; however, it has low sensitivity with a high degree of specificity, particularly for metaphyseal lesions.
Fig. 1.23. Three weeks later. Obvious periosteal new bone formation as a reaction to bilateral metaphyseal corner fractures.
DD. The diagnosis is not difficult to establish in cases demonstrating typical skeletal changes. Disorders with a similar appearance must be excluded, however, given the consequences of this grave diagnosis. Similar bony lesions are seen in the following disorders:
1.4 Fractures of the Articular Surfaces: Subchondral, Chondral, and Osteochondral Fractures
Anatomy. The important biomechanical properties of hyaline cartilage include its load-bearing capacity, its elasticity, smoothness of its gliding surface, and its durability. Hyaline cartilage is arranged in various layers. The deep calcified zone and subchondral bone are closely interlinked. Avulsion of the cartilage (traumatic separation) occurs between the deep calcified part of the cartilage and the noncalcified part adjacent to the joint. Traumatic injury to the joint surface is caused by sudden abnormal contact of the articular surfaces with one another and may take the form of shearing forces, rotational forces, or vertical impaction, depending on the mechanism of injury.
Figs. W1.2 – W1.5).
Fig. 1.27):
Clinical presentation. Clinical symptoms are typically nonspecific in nature. A hemarthrosis is often associated with an osteochondral fracture.
Radiography. A search should be made for contour irregularities and abnormal subchondral densities. Small circumscribed areas of radiolucency are suspicious for an “empty” fragment bed after dislodgement of the fragment; in those cases, radiopaque loose bodies should be looked for (
Figs. 1.28 – 1.30). Views in two planes are not always sufficient, so oblique views (of the knee, elbow, fingers, and foot) or axial views (of the patella) will often increase the diagnostic yield.
CT. CT is a very sensitive method for establishing the diagnosis of an osteochondral fracture of the joint surface. In some centers, CT arthrography, a very sensitive method, has become an established technique for diagnosing fine chondral injuries (
Fig. 1.31). CT is also the technique of choice for detecting intra-articular loose bodies.
MRI. MRI is the imaging modality of choice for making a definitive diagnosis of osteochondral injuries. Common forms of injury to cartilage and subchondral bone include:
1.4.1 Subchondral Fracture
Fig. 1.32); in the absence of a fracture line the diagnosis of a bone marrow contusion is made. Occasionally, a subchondral contusion may present as a very circumscribed hypointense area on T1W scans, reminiscent of a focus of osteonecrosis, but ultimately heal.
1.4.2 Chondral Fracture
Fig. 1.33). Chondral fissures may be longitudinal, oblique, or transverse and may extend to the subchondral bone plate (
Fig. 1.34). Associated subchondral edema is very often, but not always, seen below a defect.
Fig. 1.35).
Figs. W1.6 – W1.10 show a number of different cartilage injuries as seen on 3-tesla (3 T) MRI.
1.4.3 Osteochondral Fracture
Figs. 1.36 – 1.39). It is important to look for a fracture or depression of the subchondral plate. In some cases an osteochondral fragment may be displaced into the joint.
1.5 Stress and Insufficiency Fractures
Pathology. “Stress” refers to a force or a load exerted on a bone that is the result of muscular activity and/or body weight. Bone is a dynamic tissue that needs stress for normal development. If normal stress is removed from the bone, a rapid osteoclastic absorption of cancellous and cortical bone will automatically ensue; in addition, osteoblastic activity ceases, resulting in disuse osteoporosis.
1.5.1 Classification
Clinical presentation. The clinical hallmark of a stress fracture is activity-related localized pain and soft tissue swelling that is relieved with rest. Insufficiency fractures of the femoral neck or spine may remain asymptomatic for a very long time.
Radiography.
Fig. 1.40).
Figs. 1.40 and
1.41).
Figs. 1.42 and
1.43).
NUC MED. A bone scan is well suited for detecting stress and insufficiency fractures in the presence of negative radiological findings. A false-negative finding is a rarity in adults.
CT. CT is suitable for detecting the early findings of periosteal and/or endosteal new bone formation as well as any fracture lines (
Figs. 1.44 and
1.45). Identification of a fracture line, together with reactive sclerotic bony changes, usually allows a specific diagnosis (see
Fig. 1.47b).
MRI. MRI is an extremely sensitive method for detecting stress and insufficiency fractures, primarily because of the associated bone marrow edema that is very conspicuous on fat-saturated fluid-sensitive sequences (
Figs. 1.46 – 1.49). A fracture line may also be evident (see
Figs. 1.46,
1.48, and
1.49). It may be hypointense on T1W, T2W, or gadolinium-enhanced images or, occasionally as a hyperintense line on T2W images (see
Fig. 1.46). The low–signal intensity marrow edema may be relatively inconspicuous on T1W scans, such that more confluent low signal intensity should raise the possibility of tumor or osteomyelitis. Stress fractures may be associated with markedly increased edema within the surrounding soft tissues.
Figs. 1.50 and
W1.11).
DD.
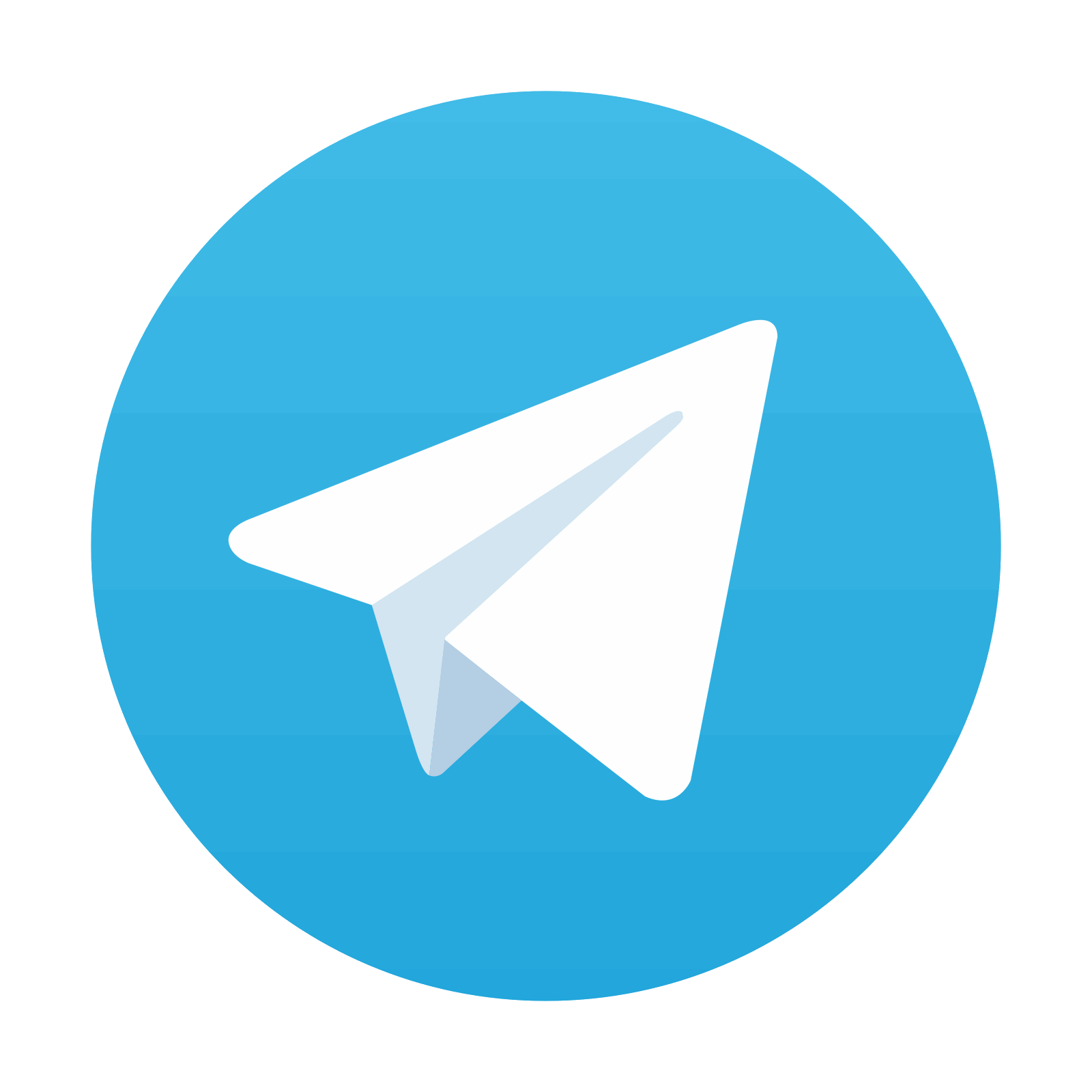
Stay updated, free articles. Join our Telegram channel

Full access? Get Clinical Tree
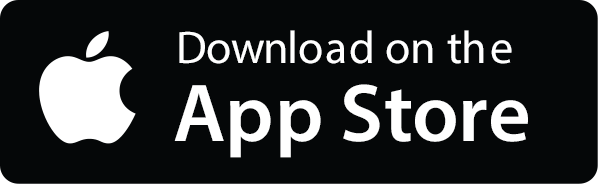
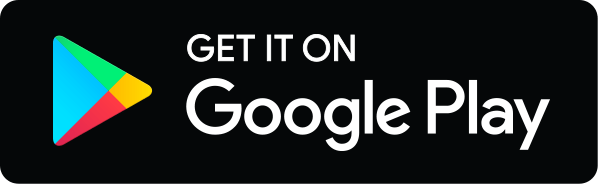