Fig. 18.1
66-year-old male with L1 compression fracture. (a) Sagittal CT and (b) sagittal STIR MRI demonstrating compression deformity with high T2 signal in L1 compatible with bone edema and acute fracture. (c) Unipedicular L1 vertebroplasty from a left transpedicular approach. (d) Post vertebroplasty with uniform cement distribution throughout the compressed vertebrae. (Courtesy of Perry Ng, M.D., University of Utah Health Sciences)

Fig. 18.2
51-year-old male with multiple myeloma and T7 and T9 compression fractures. (a) Frontal and (b) lateral fluoroscopic images with 15 mm Kyphon kyphoplasty balloons in the T7 and T9 vertebrae. (c) Magnified view demonstrating symmetrical cement deposition in the T7 and T9 vertebrae
VAT fell out of favor for a brief period of time shortly after studies in the New England Journal of Medicine published in 2009 by Buchbinder et al. and Kallmes et al. showed no benefit of VP over a sham procedure [62, 63]. This led the American Academy of Orthopaedic Surgeons to advise against the use of VP. There were, however, some important shortcomings of these studies. The patients were primarily outpatients, with pain scores as low as 3 out of 10. Additionally, patients with chronic fractures were included (>4 months old), which do not traditionally respond as favorably to VAT. Furthermore, the studies were not appropriately powered for subset analysis, which would have been necessary for evaluation of the subset of patients included in these studies with acute, severe pain. These are important factors in such studies as patients who typically benefit from these treatments are those with acute fractures associated with severe pain. Subsequent studies including the VERTOS I, VERTOS II, CAFE, and FREE trials have shown dramatic pain reduction after vertebral augmentation therapies using appropriately selected patients [64–67]. More specifically, patients having acute fractures with associated moderate to severe pain demonstrated a more dramatic, measurable benefit after VAT in these studies.
Although VAT are most commonly used in the case of painful osteoporotic fractures, there are several other indications. VP is used to treat painful primary bone tumors such as hemangiomas, treat painful fractures due to osteonecrosis (Kummel disease), reinforcement of the vertebrae prior to fixation surgery, and for treatment of painful vertebrae with malignant infiltration causing instability or fracture [59, 60]. The common malignancies that can affect the vertebral bodies include multiple myeloma, lymphoma and metastatic disease with breast, prostate, lung, bladder and thyroid cancers having a predilection to metastasize to the bone [58].
In the case of malignancy, indications for VAT are frequently tailored to the patient. The first and most obvious indication is pain associated with the VCFs with a common recommendation of at least 4 out of 10 on a base 10 visual analog scale (VAS) [15]. The second indication is edema on magnetic resonance imaging (MRI) or a positive bone scan (Fig. 18.3), indicating the acuity of the fracture. This indication is, however , occasionally flexible as good results have also been obtained in subacute or chronic VCFs refractory to conservative measures [67–69]. Bone scan can also indicate a recent neoplastic process at a compression fracture site [6, 15, 18, 21]. Imaging studies should also be used to rule out other possible causes of the patient’s pain. In addition, clinical examination should correspond with imaging studies to confirm fracture as the primary cause of pain and exclude alternative etiologies [9].


Fig. 18.3
(a) Sagittal STIR MRI of the thoracic spine with increased T2 signal in the T8 vertebra consistent with acute compression fracture. Also note chronic fracture at T11 with mild retropulsion. (b) Whole body Tc-99m MDP bone scan with posterior planar image demonstrating multiple compression fractures including T8, T9, and T10
Life expectancy of the patient is also an important consideration. Patients not expected to live for 6 months may not be good surgical candidates and in many cases may benefit from a VAT to improve their quality of life [70–72]. It should also be noted that when life expectancy is very short, VAT may be of limited value or in some cases may be an unacceptable risk. Ultimately this should be evaluated on a case-by-case basis balancing risks, benefits, patient values, and treatment goals.
Several absolute and relative contraindications exist for VP and KP. The most well established contraindications include overt instability and cord compression [15]. Cord compression on imaging is considered a relative contraindication by some in the field with special precautions taken during VAT in this population of patients [11, 18, 73]. A combination of VAT with laminectomy with or without additional instrumentation can also be used in appropriately selected cases [74]. Infection at the fracture site, bleeding disorder, low platelet count, allergy to contrast, and contraindications to local or general anesthesia are also contraindications to VAT [15]. A full preoperative work up should always be performed.
Vertebroplasty is performed under sedation or general anesthesia with the guidance of biplane fluoroscopy or CT. Polymethylmethacrylate is the most common cement that is used. A needle is placed into the vertebral body prior to cement preparation. A transpedicular approach is typically used for the lumbar and thoracic levels due to inherent safety, but a parapedicular or infrapedicular route can be used if the pedicles are too small or destroyed. An anterolateral approach is often used in the cervical vertebrae. A bipedicular approach is frequently used, although in many cases, a unipedicular approach can just as effectively be utilized depending on the patient’s anatomy (Fig. 18.1) [75]. The cement is injected in the polymerization phase to reduce risk of it entering the venous circulation or leaking outside of the vertebra. Injection is done under imaging, which allows early detection of epidural and lateral leaks. The anterior two-thirds of the vertebral body are filled evenly with cement, and the needle is removed prior to cement setting.
In kyphoplasty , bone needles are inserted into the vertebral body after which a balloon is inserted through the bone needle and inflated prior to cement injection (Fig. 18.2). A bipedicular approach is typically utilized. The goal is to create a cavity within the vertebral body and also to attempt to restore or improve vertebral body height. Cement is then injected to fill the cavity, typically starting from the anterior third of the vertebral body in a retrograde fashion as the needle is slowly retracted into the middle third of the vertebral body. Cement injection is stopped when it reaches the posterior third of the vertebral body. Because a cavity has been formed, injection of cement is under lower pressure than during injection with VP [18].
Significant pain relief has been described in many previous studies and can be expected in the appropriately selected patient population [20, 21, 65–67, 76]. Pain relief is more pronounced in VAT done in acute fractures although some improvement has been shown in more subacute and chronic fractures [67, 76]. Vertebral body height restoration of up to 34–36 % with 3–7.6° of improved sagittal alignment has been described [3, 6, 9, 15, 18, 19, 67, 75]. This has been shown to encourage upright posture, reduced future fractures, and reduced flexion movements of the involved vertebrae [77, 78]. Furthermore, multiple levels can be done simultaneously. No significant increase in operative time or morbidity rate has been seen with 3–4 levels augmented at one time [15]. The number of augmented levels per procedure should be planned on a patient-by-patient basis.
The most frequent complication of VP and KP is leakage of cement with the greater majority of cases being asymptomatic. This is particularly more risky into the posterior canal given tumors frequently involve or destroy the posterior cortex of the vertebra (Fig. 18.4). For hematogenously spread tumors, this is likely facilitated by the vascular anatomy, with blood supply entering through the basiverteral foramen posteriorly [42]. Extravasation of cement is less frequent in KP, likely due to the lower pressure during cement injection [15, 18, 76]. Many less frequent complications have been reported with the most notable being fatal penetration into vital structures; however, the rate of serious complication is very low [75, 79, 80]. Adjacent fractures can also occur although the incidence is similar or reduced compared to conservative treatment [16].


Fig. 18.4
40-year-old male with metastatic colon cancer to the spine. (a) Sagittal T1, (b) T2 STIR, and (c) gadolinium enhanced T1 fat-suppressed MRIs demonstrating innumerable spinal metastases with a larger lesion at L1 extending through the posterior cortex and compressing the ventral thecal sac (Courtesy of Roderick Willmore, M.D., University of Utah, HSC)
Initially it was hypothesized that pain resolution after VAT was that PMMA destroys pain fibers due to the exothermic effect of cement polymerization or direct toxicity from the monomer [81–84]. Other studies challenged this due to only minimal osteonecrosis, no evidence of intraosseous neural tissue necrosis, and similar pain reduction seen with calcium phosphate cement which crystallizes at room temperature [85–87]. PMMA may also simply affect vertebral body nerve fibers by mechanical disrupt during balloon inflation or the filling of the central vertebral body with cement. Cement may also simply provide internal fixation preventing pain fiber irritation [85, 86].
There is also some disagreement among experts in the field regarding the appropriate amount of cement used with no definitive amount established. Some studies suggest that smaller cement volumes may restore vertebral body strength and stiffness with adequate pain control [88]. Others propose larger amounts produce better biomechanical results. Larger volumes of cement have been shown to better correct deformities and maintain vertebral body height [89, 90]. One study specifically found that cement volume was the most important predictor for pain alleviation in a dose-dependent pattern [91]. The exact mechanism by which VP/KP provide pain relief remains somewhat controversial.
Appropriate prophylactic use of VP and KP is currently a point of disagreement. Prophylactic VP and KP have been used in vertebral bodies adjacent to the level augmented for fracture to reduce stress on those adjacent levels and prevent subsequent fractures [92]. A specific example of this is performing prophylactic augmentation in between two augmented vertebrae as this vertebra is exposed to increased forces on either side (Fig. 18.2).
Discussion more recently has been directed towards the treatment of metastatic disease prior to fracture. There is some controversy as to whether this will help reduce future fractures and patient morbidity or cause tumor spread. Combining VAT with conventional radiation is one approach, providing bone stabilization with additional local control [46]. Newer techniques such as radiofrequency ablation and cryotherapy combined with cement augmentation and radiation seem to be the next step in the treatment of metastatic spinal disease [10, 93, 94], which are discussed further in this chapter.
Thermal Ablative Therapies for Bone Tumors
Thermal ablation of bone tumors currently involves the use of radiofrequency or cryoablation probes to induce thermal necrosis in a bone tumor. Ablative technologies have been around for several years, primarily used for treatment of soft tissue tumors [24–28, 31–33]. Radiofrequency ablation has also been used for many years in the treatment of osteoid osteomas, with the first published study of this technology by a radiologist, Daniel Rosenthal, M.D. [95]. The treatment has since been performed extensively and has been shown to be effective with a good safety profile [96–100].
In the past several years, numerous studies have also evaluated thermal ablative treatments of metastatic bone tumors for patients with non-operative malignancies with favorable results [2, 35–38]. Technologies for this have primarily focused on radiofrequency ablation and cryoablation. The rationale for ablative therapies is primarily to relieve tumor pain and to also provide local tumor control. Cement augmentation can also be performed afterwards to stabilize the tumor/ablation cavity and reduce the risk of pathological fracture. As noted above, thermal ablation causes rapid local tissue necrosis, which kills tumor tissue as well as pain fibers simultaneously. Pain control is thought to be primarily due to thermal necrosis of nerve fibers. When ablation is followed by cement augmentation, pain control is also augmented by bone and/or fracture stabilization.
The goal of either ablation technology is to kill both neural pain fibers and tumor cells. This is achieved by creating a 5–10 mm treatment margin beyond the tumor borders based on the preoperative imaging. This can often be done with a single probe but at times may require multiple probes or single probe repositioning. Indications for this procedure are similar to those for VAT, with pain, local tumor control, and fracture prevention as the most common indications. Contraindications are similar to those for VAT. Additional risks to consider include integrity of the posterior wall of the vertebrae and pedicles, predicted ablation zone, and proximity to adjacent neural and vital structures.
Physicians performing these ablation procedures should also be exceptionally familiar with device placement under CT or fluoroscopic image guidance as precise probe placement within the tumor is crucial. Additional familiarity with cross-sectional imaging anatomy and interpretation is paramount for pre-procedure planning, intra-procedural evaluation and monitoring, and post-procedure interpretation. Clear knowledge of the neural anatomy is also critical when performing these therapies to reduce the associated risk of permanent neurological injuries. Appropriate training and experience in these ablative therapies is therefore critical to patient safety.
Radiofrequency Ablation Technology
Radiofrequency ablation uses high frequency alternating electrical current (200–1200 kHz) produced by the electrode. With earlier RF technology, radiofrequency ablation required grounding pads to complete the electrical circuit and prevent soft tissue burning. Many of the newer RFA devices utilize bipolar technology, eliminating the need for grounding pads. The alternating electrical current causes ionic agitation with subsequent frictional heat resulting in ionic agitation. The heat generated causes coagulative necrosis, with irreversible cell damage typically occurring between 60 and 100 °C. Above this temperature, charring and tissue vaporization occurs which can impede the flow of the current [101].
Some of the currently used RF devices for bone include OsteoCool RF Ablation System (Baylis Medical, Burlington, Massachusetts), Dfine STAR ablation (Dfine, San Jose, California), and UniBlate RFA (Angiodynamics, Latham, NY) (Fig. 18.5). Dfine STAR also has a unique tip deflection technology, facilitating directional guidance of the ablation tip into hard to reach places, such as the central or posterior portion of the vertebral body (Fig. 18.6) or other challenging locations such as the acetabulum (Fig. 18.7). OsteoCool and Dfine STAR are both bipolar devices, which is a more recent technology essentially eliminating the need for grounding pads. UniBlate and Dfine STAR also have thermocouples built into the tip provide immediate temperature feedback, which can also be utilized for ablation zone prediction (Fig. 18.8).





Fig. 18.5
Bone radiofrequency ablation systems. (a) Baylis Medical OsteoCool bipolar RFA instrument. (b) Angiodynamics Uniblate unipolar RFA instrument. (c) Dfine STAR bipolar RFA instrument (Images reprinted with the permissions of Baylis Medical, Inc.; Angiodynamics; and Dfine, Inc. 2014)

Fig. 18.6
47-year-old female with multiple myeloma with L2 compression fracture. (a) Axial, coronal, and sagittal CT images demonstrating lytic L2 lesion. (b) Dfine STAR RFA instrument via left unipedicular access of L2 with tip deflected into the central portion of the vertebrae. (c) Sagittal and (d) coronal intra-procedural spin DynaCT 3D reconstructed images demonstrating before and after cement deposition within the vertebrae to fill the ablation/tumor cavity

Fig. 18.7
(a) Axial and (b) sagittal oblique CT images of the hip demonstrating superior acetabular lesion with Dfine STAR ablation instrument directed into the lesion (Courtesy of Jack Jennings, M.D., Ph.D., Washington University)

Fig. 18.8
Dfine STAR distal and proximal thermocouples (circled) for real-time monitoring of ablation zone
Ablation cycles are typically for 10–15 min, depending on the size, shape, location, and intrinsic characteristics of the tumor. RFA also has a cauterizing effect which reduces the risk of bleeding. This quality is particularly advantageous for hyper vascular tumors or coagulopathic patients. RFA has also been shown to have a synergistic effect with radiation therapy, thereby improving the therapeutic effect and potentially survival.
One of the challenges with RF ablation is the inability to clearly visualize the treatment zone during ablation. The exception to this is with ultrasound, where gas formation during ablation is clearly seen. However, the gas also creates posterior acoustic shadowing, which obscures visualization of the tumor. As mentioned above, some of the newer devices have thermocouples attached on the device (Dfine STAR, San Jose, California) to more accurately monitor the treatment zone by tracking the temperature during treatment rather than relying on imaging. Additionally, the active tips of the RF probes can be difficult to see on CT due to streak (beam hardening) artifact, which can make exact placement within the tumor challenging.
Cryoablation Technology
Cryoablation is another current technology that is being used more frequently for treatment of bone tumors with good reported outcomes [102–105]. It is actually an older technology, being first used to treat breast and uterine cancers in the 1840s. It gained more traction in the 1960s when trocar-type probes were designed, primarily for treatment of liver tumors [106, 107]. The technology takes advantage of the thermal properties of highly pressurized gases, typically argon or nitrous oxide. As the gas travels through the thermal probe to the tip, the gas expands at the applicator tip causing the temperature to rapidly drop. This is known as the Joule-Thompson effect, with temperatures of −80 °C to as low as −160 °C possible [101]. A temperature between −20 and −40 °C is necessary and needs to persist 1 cm beyond the tumor periphery [107–109]. Most current probes also use Helium to thaw the tip, which heats the probe tip when the gas expands. Freeze cycles are typically 10 min followed by an 8 min thaw cycle, with ablations frequently requiring two freeze/thaw cycles. Some of the cryoprobes currently used for cryoablation are produced by Endocare (Healthtronics/Endocare Incorporated, Irvine, Calif) and Galil Medical (Galil Medical, Arden Hills, Minnesota) (Fig. 18.9).


Fig. 18.9
(a) Endocare and (b) Galil Medical bone cryoablation instruments. (a) Provided with permission of Endocare, Inc. a wholly owned subsidiary of HealthTronics, Inc. © 2013 HealthTronics, Inc. All Rights Reserved. (b) Used with permission. ©2014 Galil Medical
Cryoablation is thought to work through multiple mechanisms. Ice crystal formation disrupts cellular membranes and denatures intracellular proteins interrupting cellular metabolism. It also coagulates blood, interrupting blood flow to the tissue resulting in cell dehydration and ischemia. This cascade of events promotes apoptosis and subsequent cell death. Additionally, there is the potential for immunomodulation with stimulation of the immune system, leading to immunological targeting of tumor cells. This is believed to occur by an immune response against sub-lethally damaged or untreated tissue, being first seen in treatment of prostate cancer in the 1970s. This is currently a matter of debate as there is also evidence for a paradoxical immunosuppressive effect [106, 107, 110, 111].
Cryoablation has the advantage of smaller ablation probes, with probes as small as 17 gauge (Galil Medical, Arden Hills, Minnesota). It also has the added benefit of a clearly visible ablation zone (ice ball) during the ablation, which can be seen on CT, MR, or ultrasound. It is also thought to have less post-procedural pain. Caution, however, must be used near neurological structures as it can also cause permanent neurological injury, and patients may have no physical signs of this during the procedure. This is of particular concern in areas such as in the vertebral body pedicle with the adjacent nerve roots or in lesions abutting the spinal cord.
More recently, Callstrom et al. have measured neurological response during cryoablation to reduce the risk of neurological injury [105]. This is achieved by monitoring motor evoked potentials during stimulation of involved musculature during treatment. This method has helped to reduce this risk of neurological injury dramatically. Additionally, in contrast to RF ablation, cryoablation does not have a cauterizing effect on ablated tissue. Therefore, caution should also be used with hypervascular tumors or in patients with underlying coagulopathy due to the risk of bleeding post ablation. More recently, newer probes offer post-ablation cauterization (IceRod CX, Galil Medical, Arden Hills, Minnesota) to reduce the risk of post ablation bleeding. Another potential drawback of cryoablation is the amount of time it takes to perform the ablation, at time requiring upwards of 30–50 min depending on the size of the tumor and the number of freeze and thaw cycles.
Post ablation Osteoplasty
After ablation, the residual necrotic cavity frequently benefits from cement augmentation to stabilize the necrotic bone cavity, thereby reducing the risk of future compression fracture (Fig. 18.10). This risk is particularly high for lesions involving >40 % of the vertebrae and below T10, especially for mixed or lytic lesions.


Fig. 18.10
72-year-old male with metastatic lung cancer to the thoracic spine. (a) Axial, coronal, and sagittal reconstructed DynaCT images demonstrating lytic T3 lesion as well as prior kyphoplasty changes at T5. (b) Sagittal and coronal 3D reconstructed images demonstrating Dfine STAR ablation device within T3. (c) Sagittal fluoroscopic images demonstrating polymethylmethacrylate (PMMA) cement injection into T3. (d) Axial, coronal, and sagittal reconstructed DynaCT images demonstrating PMMA cement within the ablation cavity
One of the potential drawbacks of VAT without pre-ablation is theoretical tumor displacement into adjacent normal/healthy tissue by either the kyphoplasty balloons or injected cement during vertebroplasty. One of the benefits of ablation prior to cement augmentation is the destruction of malignant tissue, which reduces the risk of local tumor displacement and possible extension into normal healthy tissues. Ablation also likely creates an ablation cavity due to tissue dehydration and vaporization, creating a potential space for bone cement deposition.
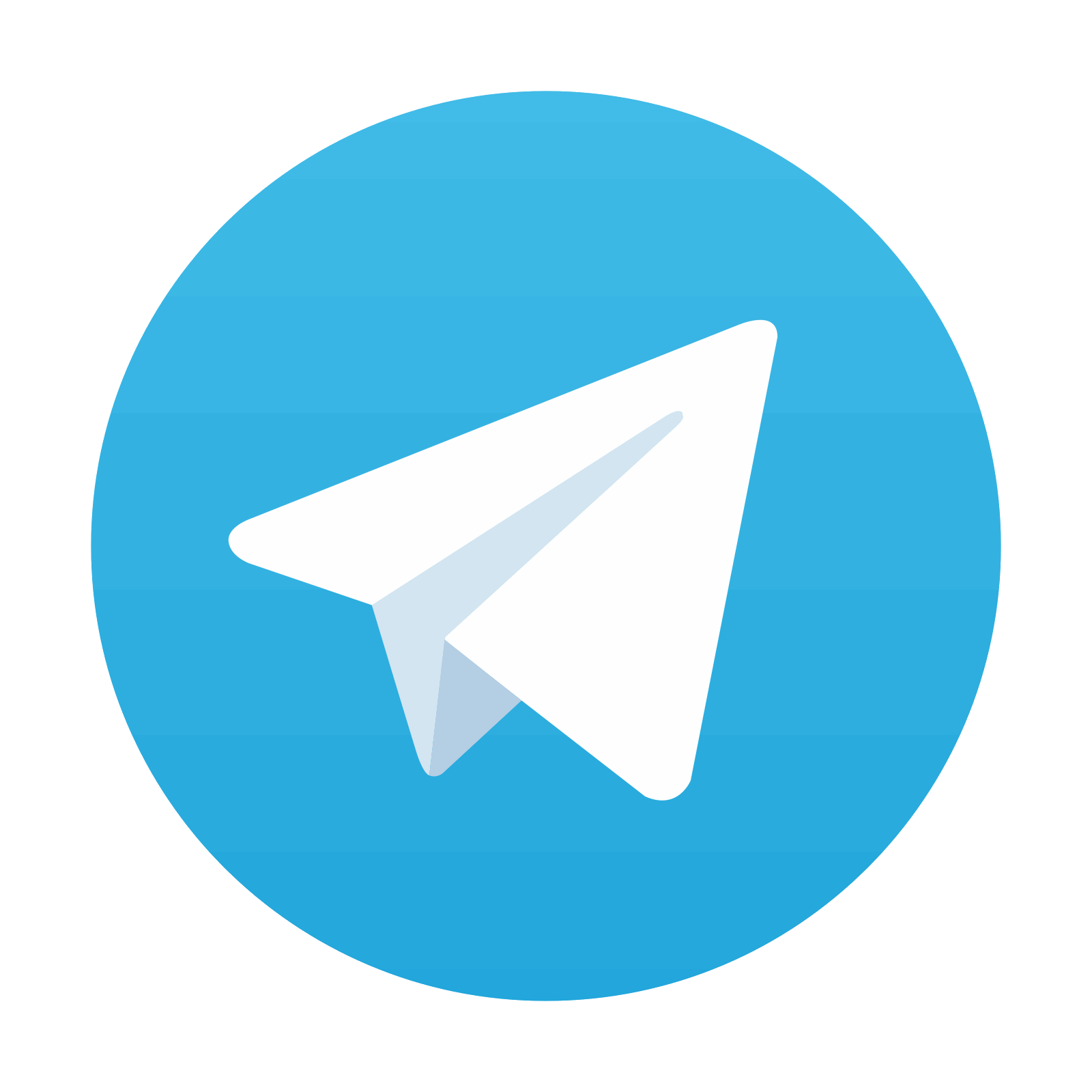
Stay updated, free articles. Join our Telegram channel

Full access? Get Clinical Tree
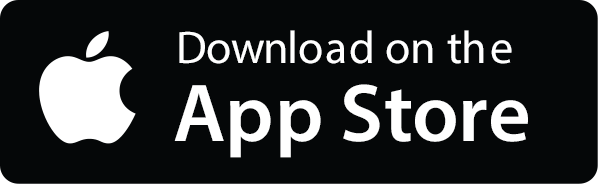
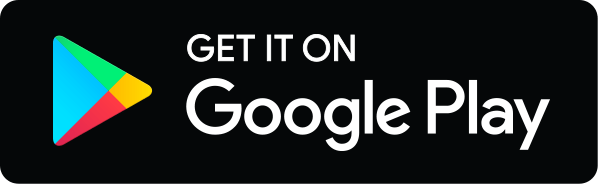