
Fig. 16.2
Garage door analogy of the musculoskeletal system

Fig. 16.3
The Brachial Plexus

Fig. 16.4
Dermatomes of upper limb
This strategy can be summarized with these key points of analysis:
- 1.
What functions are missing?
For example, arm abduction
- 2.
What functions are interfering with others?
For example, inability to flex the elbow in spastic paralysis
- 3.
What functions are spastic vs. flaccid?
For example, flaccid biceps with spastic triceps, an example of “antagonistic paralysis”
- 4.
What functions can be/are desirable to be restored?
For example, pronation/supination may not be worthwhile to restore.
For example, wrist extension is only useful for power grip, if there is insufficient finger.
Flexion for power grip, it may be preferable to fuse the wrist.
- 5.
Which donors can be used to restore function?
- 6.
Which new donors can be created to restore function?
- 7.
Nonneural limitations to functional restoration?
For example, orthopedic injury, contracture, and cognitive issues
Assessment of Function
There are several different diagnostic maneuvers that give the surgeon insight into the possible utility of specific surgical interventions. For example, bimanual palpation can give clues to which distinct portion of the muscle is functioning by determining spasm or contraction. When palpating a patient’s deltoid muscle, abducting the arm in different directions may allow differentiation of individual muscle heads. With elbow extension, it is possible to feel the three distinct heads of the triceps. Muscles may also be differentiated based on their position during function. The biceps flexes the elbow with the hand in supination, while the brachialis flexes the elbow with the hand pronated. Another maneuver that may be used is temporary motor blockade with lidocaine or Botox. If the brachialis is iatrogenically paralyzed via the radial nerve, it is possible to determine how much the biceps itself is contributing to elbow flexion via the musculocutaneous nerve. It is important to note that there are limits to the physical exam. These subtle differentiations can be extremely complicated, e.g., differentiating between the ECRB (extensor carpis radialis brevis) and ECRL (extensor carpis radialis longus) muscles, and may only be performed under direct visualization in the operating room.
Assessment of Pain
The motor deficit of paresis or paralysis is the most obvious defect, but not necessarily the most important. For many patients, especially those with paralysis, the most important functional consideration is unremitting pain. This can be easily overlooked by the medical and surgical team but is often one of most central issues in patients’ life and daily functioning. It is important to remember that an injury severe enough to cause paralysis may be accompanied by pain for a variety of reasons.
Pain may be secondary to four possible cases: (1) direct nerve pain, (2) neuroma, (3) joint subluxation and orthopedic derangement, and (4) phantom limb syndrome. For example, a trapezius to deltoid transfer has been well described as a technique for improving arm abduction [20–22]. However, many patients who require this procedure are in fact more greatly aided by the repositioning of the shoulder joint resulting in reduction of glenohumeral subluxation that occurs with the transfer, which ameliorates their pain, rather than simply the improved abduction [22]. This can be tested preoperatively by reducing the patient’s shoulder in the office, which often provides immediate relief for a sensation described as “carrying a heavy piece of luggage and never getting to put it down.”
There are also multiple potentially straightforward interventions for nerve-related pain that have the ability to significantly improve quality of life. Neuroma and phantom limb syndrome can be differentiated by injection of lidocaine into the nerve stump. If the pain resolves, it is likely secondary to neuroma, which can be treated by direct excision or other means. A patient with brachial plexopathy but without a distal limb traditionally might not be offered brachial plexus grafting because of lack of functional targets. However, these grafts have the potential to improve distal pain and therefore should be considered in the overall operative approach.
Assessment of Donors
Nerves
For the reconstructive surgeon, not all nerves are created equal. There are a number of key considerations in selecting a donor nerve. What loss of function will occur after nerve harvest? One strategy to minimize this loss is using a branch of the nerve after arborization of its crucial branches. Another is to use a nerve to a muscle that is either synergistic with intact muscles or no longer functional after the patient’s injury. For example, if a patient is unable to move their arm, the stabilization of the scapula by the serratus anterior via the long thoracic nerve is no longer a significant consideration. In this situation, the long thoracic becomes an excellent donor.
What is the length of the defect? This will determine whether additional nerve graft interposition is required with the neurotization, which unfortunately will decrease the success rate. For example, the spinal accessory nerve will reach the suprascapular nerve for neurotization but will require additional graft to reach the axillary nerve. Where is the injury relative to the motor end organ? If the nerve has to travel a long distance after neurotization, the likelihood of a successful result diminishes considerably. A classic example of this is the “baby sitting procedure,” performing an anterior interosseous nerve transfer for distal ulnar nerve palsy to prevent claw hand. A more proximal neurotization would be much less likely to reinnervate the small interossei within a reasonable time frame prior to motor end plate degeneration and muscle atrophy.
Another key concept in choosing donors is that of axonal density. It is important to choose donor nerves that will allow the patient to obtain meaningful movement, which is more than simply movement against gravity. Part of the selection process for nerve donors must take into account axonal density, essentially a relatively high number of axons for the nerve diameter. The surgical result will be optimized when the donor and recipient nerve are matched in terms of total diameter and average axonal density [23–25]. In order to maximize power of nerve transfer, the donor nerve axons need to fill up as much of the cross section of engrafted nerve as possible. A loose analogy to better understand this concept is that of voltage. Much more voltage – and wiring to transmit that voltage – is needed to power the air conditioner of a house than a stereo. Similarly, if a large muscle needs to be powered, the donor nerve used to power it must have high axonal density [26].
A clinical example is a comparison between the spinal accessory and the intercostal nerve. The spinal accessory nerve has much greater axonal density than does the intercostal nerve. A rule of thumb to maximize axonal density is to choose a small nerve – like the spinal accessory nerve – that powers a large muscle, e.g., the trapezius. The intercostal nerve is similar in diameter to the spinal accessory nerve, but the intercostal muscle is much smaller than the trapezius is. A single intercostal nerve with its low axonal density would not be sufficient to power the biceps, and nerve transfer via intercostals to the biceps typically requires multiple intercostal nerves. It is also useful to consider other less, common donors that historically have been overlooked. For example, the nerves to the levator scapulae and sternocleidomastoid have good axonal density and are also synergistic. Another option to maximize axonal density is the use of cadaver nerves or living-related sources of donor nerve in order to perform multiple cable grafts [26, 27]. Muscles that require strength like the biceps or the deltoid may require the use of these adjuncts in order to maximize function, whereas a less dense nerve may be sufficient for a smaller muscle like the flexor pollicis longus (Figs. 16.5, 16.7, 16.8, 16.10)







Fig. 16.5
Intraplexal nerve grafting

Fig. 16.6
Traction injury to the brachial plexus

Fig. 16.7
Transfer of the right triceps branch to the axillary nerve

Fig. 16.8
Transfer of the right triceps branch to the axillary nerve

Fig. 16.9
Ulnar fascicular transfer to the biceps motor branch (Oberlin’s method)

Fig. 16.10
Commonly used techniques for end-to-end flexor tendon technique
Muscles
There are five main considerations for evaluation of muscle donors: (1) strength, (2) excursion, (3) length, (4) direction of pull, and (5) available resources, i.e., what has been spared vs. what can be created as a new donor. The surgeon needs to evaluate potential donors based on strength. Muscle strength is evaluated based on the MMT (manual muscle testing) grading system [28]. Any muscle less than M4 (holding an antigravity position against moderate resistance) is not suitable to be used for transfer. For example, the palmaris longus is a weak muscle but would be appropriate for transfer after median nerve palsy in an elderly patient who does not require much strength for thumb opposition. There is also a balance and inverse relationship between excursion length and strength, and the muscle used for transfer will depend on the requirements for function. Unipennate muscles tend to have good excursion but poor strength, while bipennate muscles have better strength but poor excursion. The direction of pull and the existing and fabricated pulleys also must be evaluated.
This muscle evaluation also must occur in the context of a larger understanding of patient function, especially planning out multiple operations in the future. One muscle that is nearly always spared, even in relatively high spinal cord injuries, is the trapezius. Its innervation, the spinal accessory nerve (CN XI), and contributions from C3 and C4 travel with the cranial nerves [22]. An injury severe enough to compromise these nerves is typically fatal. Because of this near universal availability, trapezius transfer is the most common reported transfer in adult palsy [22].
Creation of New Donors and Donor “Disease”
Using the multistaged approach, it is also possible to expand the pool of donor muscles. Muscles subsequently used as donors may not have been initially spared by the injury. For example, during an initial surgery to reconstruct arm abduction, the latissimus can be engrafted. This allows this muscle, which may not have initially been functional, to be used as a tendon transfer for biceps or triceps reanimation. It is always important to consider what new deficits or “donor disease” will be created when a donor muscle or nerve is used. What is acceptable in one patient may present too great a disability in another. For example, a patient who cannot flex their elbow is better served by using their latissimus muscle as a donor than would be a professional pitcher. The procedure needs to be personalized for the patient and their specific, unalterable limitation, e.g., inability to perform manual labor.
Some donors, although classically acceptable, create too large a deficit when appropriately understood and should not be used. The phrenic nerve has been previously recommended as a donor for restoration of elbow flexion and restoration of shoulder abduction [29–31]. However, the loss of this nerve has measurable negative consequences. Unilateral diaphragmatic paralysis has been associated with exertional dyspnea, orthopnea, sleep disturbances, and gastrointestinal reflux, all symptoms causally linked to a decrease in resting and active diaphragmatic tone [32]. The phrenic nerve should never be used as a nerve donor for transfer. In fact, we advocate phrenic nerve reconstruction in appropriately selected patients, e.g., patients’ status post cardiac surgery or other iatrogenic causes with the symptoms described above. Patients who have undergone reconstruction of the phrenic nerve via neurolysis, neurotization, or interpositional nerve grafting have significant improvements in pulmonary function testing and report better scores on quality of life surveys [33].
Electromyography and Imaging
The clinical exam, however, has its own limits. It is difficult to discern muscles that (1) are synergistic, (2) are grouped, or (3) have duplicated function. For example, the deltoid needs to work synergistically with the biceps in order to stabilize the shoulder girdle during elbow flexion. Grouped muscles are those considered often as one muscle that functions together but has distinct sections or heads that may have different levels of activity after injury, like the three sections of the trapezius or three heads of the triceps. Although certain clinical maneuvers can help, muscles with duplicated function – like the extensor digitorum communis to the index and the extensor indicis proprius – may be difficult to differentiate.
Additional testing serves as a necessary adjunct to any complete physical exam but especially in situations of synergistic, grouped, or duplicated muscles. Electromyography, as mentioned above, is the cornerstone of any careful musculoskeletal evaluation. It is helpful to have a dedicated electromyographer who not only has experience in brachial plexus and peripheral nerve injuries but also an appreciation for the subtlety involved in diagnosis [9]. Minor muscles that perform the same function may have been spared by the brachial plexopathy or spinal cord injury and may function as useful donors. For example, the brachioradialis and brachialis flex the elbow, just as the biceps does. However, if the surgeon and the electromyographer do not think to evaluate the brachialis, its suitability for use as a donor cannot be determined. Another example is determining if the muscle keeping a patient’s arm in spasm is the triceps or the anconeus, which can be difficult to determine without an experienced electromyographer. In fact, the assessment of a skilled electromyography may be predictive in determining the outcome of nerve transfers [34].
Further imaging is also required. A basic chest x-ray can determine if the patient has fracture of ribs, possibly eliminating the intercostal as a donor, the clavicle or scapula. An x-ray can also demonstrate phrenic nerve paralysis with deep inspiratory and expiratory views [9]. Additional assessment of neuromuscular function using pulmonary function testing and electrodiagnostic testing can be used to further elucidate the degree of phrenic nerve dysfunction [32]. A CT myelogram should be performed in addition to an MRI of the affected brachial plexus. Traction that disrupted the brachial plexus at the initial trauma may damage the subclavian vessels or cause pseudoaneurysm formation [9]. An MRA and MRV of the subclavian and axillary arteries should also be performed to rule out any vascular injury or malformation (Fig. 16.6).
Introduction to Surgical Planning
Surgical Timing
Another difficult aspect to approaching any patient with a nerve deficit is determining the correct time for operative intervention. Many patients will improve without intervention. However, by 12–18 months after the injury, all the motor end plates will have undergone irreparable atrophy [35, 36]. How should the correct patient and correct timing of the operation be determined? If a patient has a sharp injury, e.g., a knife wound to the brachial plexus, the intervention should be performed as soon as possible. If the injury involves a gunshot wound or blunt mechanism, it can often be unclear whether the deficit is secondary to neuropraxia or a true, irremediable injury. Another complicating factor is that many injuries are mixed and may contain elements of several different Sunderland grades of nerve injury [37].
We believe that early and aggressive surgical treatment is the optimal approach in order to restore function [26]. Our practice in these injuries is to obtain a baseline electromyography (EMG) at 6 weeks, with a repeat exam at 3 months. If there has been no improvement over 3 months, the operation is performed immediately. Typically, these more severely injured patients will also have a clear, non-advancing Tinel’s sign where neuropraxia patients often have no overt Tinel’s [38]. What to do if some improvement is observed yet the patient has not returned to normal, baseline nerve function? If the patient is only 95 % improved by 6 months or if there is anything more than a subtle difference remaining by 9 months, we also recommend surgical intervention. These patients also require ongoing physical therapy from the time of their initial diagnosis. Patients who have inadequate therapy may develop contractures that create functional deficits [9].
The Surgical Planning Process
The most critical aspect of preoperative evaluation and surgical planning is the ability to think ahead regarding all the procedures the surgeon must perform to recreate function for the patient and minimize pain. Furthermore, the patient must understand that the surgeries themselves are a process and not an event. This process will require multiple surgeries that are necessarily fraught with trial and error without sure guarantees about what function will return. There are also practical rehabilitation rationales for operating in multiple stages. The therapy required for a flexor or extensor transfer or for a joint function will be completely different. Attempting to combine surgeries with opposing actions into one procedure necessarily prohibits optimal rehabilitation, a key component of the best possible outcome. It is also necessary to pay attention to the patient’s entire medical and surgical condition, not focusing on the injury alone. Their injuries may occur at multiple levels on the extremity in addition to being one of the multiple systems injured. For example, a patient with a brachial plexus injury combined with an orthopedic shoulder injury or a head injury will not be treated in the same way as one with the brachial plexus injury alone.
Any intervention must be planned as part of a cohesive and coherent whole. There are many different modalities that can be used to improve these patients’ function, including neurotization, nerve transfer, tendon transfer, arthrodesis, and several other types of procedures. Care for these patients must be provided by either a single surgeon who is experienced and capable of performing all of these modalities or by a coordinated team of surgeons who, as a unit, serve the same purpose. It is a disservice to the patient to perform a single-nerve transfer without placing this intervention within the larger patient goals. This represents a paradigm shift in how we as physicians approach the problem of upper extremity reanimation. The specific surgical treatments will be addressed in a subsequent paper.
Targeted Muscle Reinnervation
Almost 2 million people are living in the United States with limb loss with approximately 185,000 amputations happening each year [39, 40]. Loss of the use of an upper extremity can be devastating. Prostheses have provided a solution both cosmetically and functionally for these patients. The early pioneers of the prosthesis were the Egyptians [41]. Writings as early as 424 BC describe a man amputating his own foot and replacing it with a wooden filler to continue to walk [41]. In the first century AD, tradesmen designed and created artificial limbs, even adding intricate functions using springs and gears [41]. The US Civil War forced an entrance into the field of prosthetics in America as the number of amputations increased [41]. After World War II, the United States government collaborated with military companies to improve the function of existing prostheses [41].
New advances in targeted muscle reinnervation (TMR) have enabled prostheses to function at a much higher level. Previously, patients with above-the-elbow amputations only had the options of body-powered and myoelectric prostheses that rely on functioning muscles at the level of or just proximal to the amputation level [42]. TMR allows for more coordinated movement as multiple joints can move at the same time [42]. TMR has not only allowed improved control of prostheses but also has the potential to relieve post-amputation neuroma pain [43]. The mechanism behind TMR is the use of a series of nerve transfers to provide “intuitive prosthetic control to upper extremity amputees” [43]. TMR uses transfer of transected peripheral nerves to reinnervate intact muscles and the overlying skin [44]. A pattern recognition algorithm is used to interpret the surface electromyographic signals from the reinnervated muscle [44]. These signals are used to provide control signals for the prosthetic device [44]. TMR is most often utilized in the transhumeral and shoulder disarticulation patient population [43].
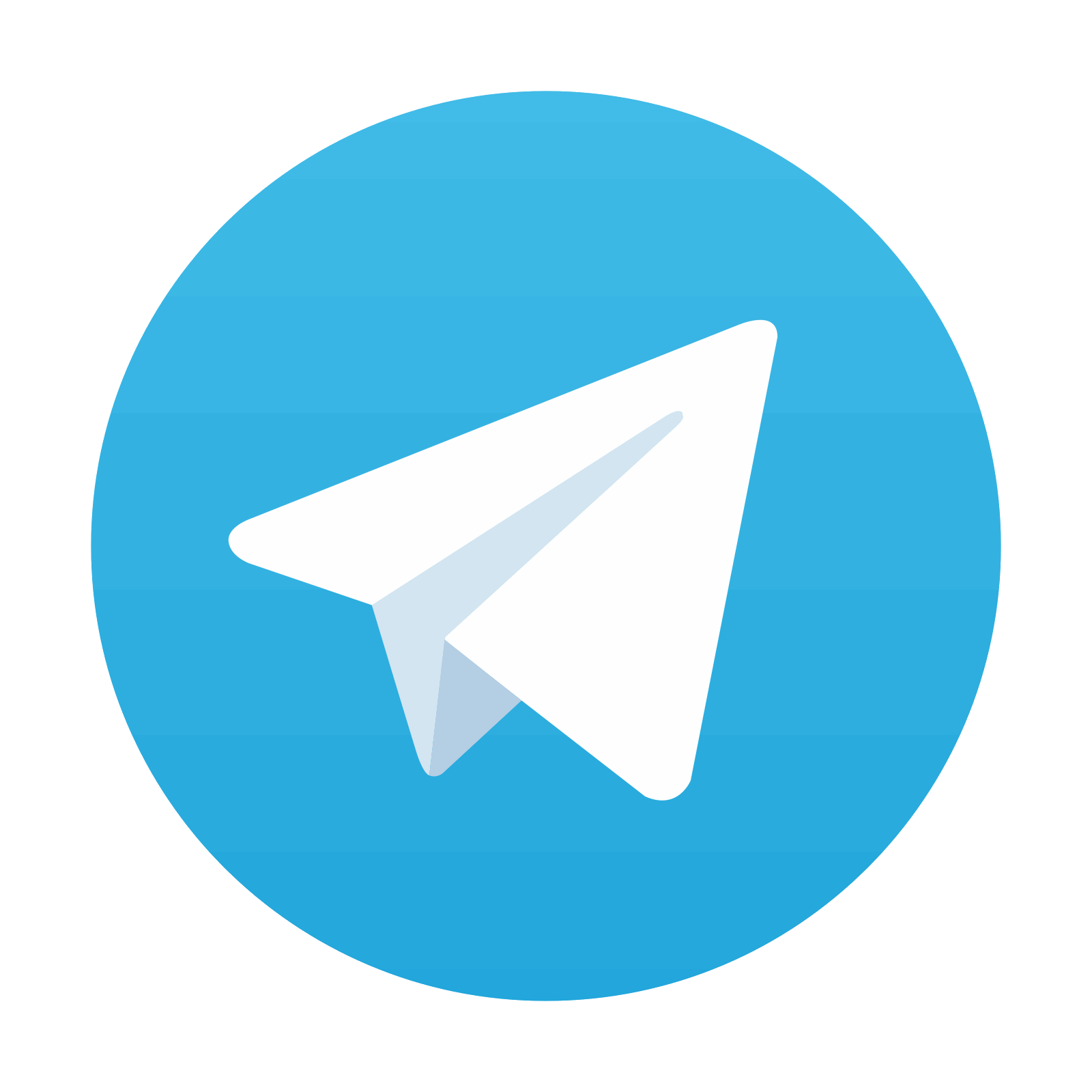
Stay updated, free articles. Join our Telegram channel

Full access? Get Clinical Tree
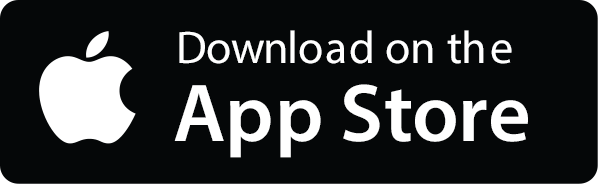
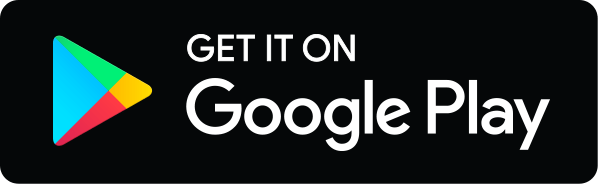