9 Surgical Intervention
Surgical intervention in the management of noncongenital early onset scoliosis and infantile idiopathic scoliosis is indicated if there is a failure of conservative management (either casting or bracing) or if the deformity is considered too severe at presentation for conservative treatment to have any real prospect of success. The aims of the surgical treatment of early onset scoliosis and infantile idiopathic scoliosis are to control the spinal deformity while maximizing both cardiac and respiratory development and allowing the spine to grow and gain the most “normal” length possible. Surgical intervention takes many different forms, but essentially these consist of distraction-based posterior instrumentation systems, growth guidance instrumentation, or anterior spine–based tethers. All of these methods of managing early onset scoliosis have their indications, contraindications, and specific complications. In reality, however, there can be no one “right” answer for the management of all forms of spinal deformity, which continues to be a difficult and taxing problem. The aim of this chapter is to introduce these concepts and indicate where the different types of operative intervention may be employed, to consider the specific pathologies that may be addressed, and to discuss the contraindications and complications of each particular type of operative intervention.
9.1 Classification of the Operative Treatments of Early Onset Scoliosis
Skaggs et al have described a classification of growth preservation techniques in early onset scoliosis: 1
Nonoperative
Observation
Bracing
Casting
Operative
Posterior distraction systems
Growing rods (single or dual, manual or remote lengthening)
Vertical expandable prosthetic titanium rib
Hybrid spine-to-rib construct
Growth guidance
Luque trolley
Shilla / Shilla-like
Anterior tether-based
Screw cable / ligament
Osteotomy
These are subdivided into nonoperative and operative groups. The nonoperative group includes serial observation, casting (with or without traction), and bracing. All of these techniques are indicated in certain circumstances and have been used worldwide with great success. They are covered elsewhere in this book and are not the subject of this chapter.
The operative group is subdivided into three groups:
Distraction-based techniques include “growing rods” (either single or dual), the vertical expanding prosthetic titanium rib (VEPTR), and “hybrid” spine-to-rib constructs. What is common to all these systems is the requirement for intermittent lengthening of the construct (through either serial reoperation or some form of external (usually magnetic drive) distraction.
Growth guidance instrumentation is aimed at allowing the spine to “grow” along a predetermined “path”; the instrumentation is used to guide growth in the appropriate direction. This does not depend on serial lengthening procedures. This was the original segmental instrumentation concept of Luque, developed initially in the Luque trolley (and modern pedicle screw–based derivations) and subsequently refined in the Shilla technique.
Anterior tether based systems function by placing nonfusion instrumentation across the growth plates of the vertebral bodies—a cable, a ligament, or multiple staples—in an attempt to retard growth on the convex side of the spine in the hope that the concave side will “catch up” with the induced asymmetric growth. 2
The effort to preserve growth is based on the understanding that we currently have about how early fusion inhibits spinal growth and growth of the thorax. This inhibition then interferes with the development of both the heart and lungs and leads to morbidity and early mortality through respiratory failure, as demonstrated by Pehrrson et al. 3 Subsequent work by Karol et al has shown that failure to attain a minimum vertebral height of the thoracic spine of 18 cm at maturity is strongly correlated with respiratory morbidity in early adult life. Consequently, all growth preservation systems are aimed at attaining at least 18 cm of thoracic spinal height, if not more, to maximize thoracic growth and development of the underlying organs. 4
Surgical growth preservation attempts to match as closely as possible the normal growth of the spine and thorax, as documented previously by Dimeglio et al, with the overall aim of reaching as closely as possible a spine of normal length and normal three-dimensional development of the thoracic cage and underlying organs. 5
9.2 Distraction-Based Instrumentation
9.2.1 Growing Rods
This posterior distraction-based instrumentation system was Harrington’s original concept in the 1960s for the management of scoliosis following polio. A strut was placed on the concave side of the apex of a deformity to prevent the deformity from increasing in size. The strut was then lengthened periodically in an attempt to maintain any correction obtained at the index surgery. Any growth obtained was of secondary concern and is considered only briefly in Harrington’s papers. However, no rotational control was associated with this instrumentation because the hooks did not lock to the rods. Consequently, significant crankshaft developed, and unchecked anterior growth in the presence of a posterior tether sometimes resulted in an even worse deformity than had been present initially. The other complication was a fracture rate of more than 66% during the lifetime of the construct. 6
Second-generation posterior distraction based mechanisms were brought to the fore with implant systems such as the pediatric ISOLA Spine System (DePuy Synthes Spine, Raynham, Massachusetts), which has been the basis of the growing rod program in Birmingham. The construct employs either single or dual rods placed posteriorly to the spine with combination hook or screw foundations at both the proximal and distal ends of the curve. The Birmingham approach is always to use a dual rod construct, with the foundations typically placed in the upper thoracic spine and midlumbar spine. The approach is through a long posterior skin incision followed by subperiosteal exposure only of the vertebra required for the foundations. The procedure, analogous to a Wiltse-type approach, can be performed through four small incisions lateral to the midline over the foundations, with the rods then tunneled between the skin bridges. The rods are placed in a submuscular plane (or subcutaneous plane) to minimize skin problems and maximize soft tissue cover. Tandem growing connectors are placed over the relatively straight sagittal thoracolumbar junction outside the lumbar lordosis or thoracic kyphosis (Fig. 9.1). This approach allows the greatest part of the curve, including the apex, to remain undisturbed during implantation because no periosteal stripping of the posterior musculature from the back of the spine is required.

At regular intervals (dictated by pathology, but usually every 6 months), the construct is lengthened. This is a day case procedure in which a small incision is made over the tandem connectors without disturbing the midline. The upper rod is then distracted away from the lower construct to lengthen the spine. This process is repeated until maturity is reached or the spine autofuses and becomes stiff, such that further lengthening procedures are not possible. Studies have shown that approximately seven lengthening procedures are possible before the spine stiffens and lengthening achieves little and becomes increasingly difficult. 7 The timing of starting the growing rod program must take this limitation into account in conjunction with matching the growth velocity of the child. Thus, the average age for the implantation of growing rods is approximately 6 years. However, each case must be reviewed on an individual basis, and failure to control the curve may force operative intervention at an earlier age.
Once maturity has been reached, if there is still evidence of an articulation with patent facet joints or well-preserved disk spaces across the apex of the vertebral body, then a final fusion procedure is often recommended. The posterior implants are exposed and the rods exchanged. Further correction can be obtained through osteotomies of the posterior spinal elements. The posterior elements of the spine are then decorticated. Fusion is stimulated by adding either allograft or artificial bone to the autograft harvested from the posterior elements of the spine. This can be supplemented with iliac crest autograft from the posterior superior iliac spine as required.
Studies of the original cohort of pediatric ISOLA growing rod instrumentation patients through to graduation and beyond as studied and documented by the Growing Spine Study Group (which included the Birmingham cohort) has shown that a dual growing rod technique resulted in 5.7 ± 2.9 cm of spinal growth during a treatment period of 4.37 ± 2.4 years, with a greater growth rate for those lengthened at intervals of 6 months or less than for those lengthened at intervals longer than 6 months. 8
Growing rods are suitable for a curve requiring instrumentation when fixation is possible at both the proximal and distal aspects of the curve, and in recent times these have evolved to become a screw-based construct distally and a screw or screw-and-hook construct proximally. Growing rods are contraindicated if it is not possible to obtain sound fixation to the spine or if the child is not suitable or will become unsuitable for multiple reoperations, even if these are of a lesser magnitude than the initial insertion of instrumentation. Newer materials have meant that growing rods, pedicle screws, and hooks can now be manufactured in titanium, so that the imaging difficulties that arose with steel implants in magnetic resonance (MR) imaging scanners have now been minimized. The infection rates also seem to have been reduced. 9
Although posterior growing rods are initially inserted with the assistance of spinal cord monitoring, monitoring is not always employed for lengthening procedures. Neurologic complications following a lengthening of growing rods have been reported anecdotally. These have ranged through the entire spectrum, from a temporary loss of motor and sensory function that returned fully on removal of the distraction to permanent incomplete neurologic loss. Good practice dictates that a wake-up test always be performed in the operating theater following any lengthening procedure, with the instruments kept sterile until the child has passed the wake-up test satisfactorily and is seen to move both arms and legs purposefully with normal power to command. This is done even if multimodal monitoring has been used throughout the lengthening procedure.
The complications of growing rods are threefold. First, there is a risk for infection in the construct. This is because the spine and implant are exposed on multiple occasions through the same incision over a period of many years. The soft tissue in this area will become stiff and scarred, with a poor vascular supply, which adds to the overall risk. The infection rate has decreased with the use of titanium implants rather than steel because of the surface oxidation properties of titanium. Infection is managed similarly to any orthopedic infection, with débridement and often VAC (vacuum-assisted closure; Kinetic Concepts, Inc., San Antonio, Texas) therapy. A persistent deep infection can make it necessary to remove all instrumentation, including hooks, screws, and rods, to eradicate the infection. On removal of the implants, control of the scoliosis is lost, and the deformity can recur rapidly. An alternative method of curve control must then be employed, such as a brace or plaster, while the infection is cleared. Once this occurs, then re-instrumenting the spine to continue the growth program or converting to another form of construct can be considered.
The quoted infection rate for a growing spine construct over the life span of the construct, including the multiple reoperations for lengthening, rod revision, etc., is 10.4%. 9 Infection rates are increased in a growing spine construct by poor care in the surgical approach to lengthening, but also through a subcutaneous placement of the rods and poor skin over the prominent implant (especially in smaller children). Certain conditions, namely Prader–Willi syndrome, mucopolysaccharidosis, and graft-versus-host disease, predispose to infection.
The second complication of growing rods is rod fracture. This is thought to be due to cyclical loading of the construct over the unfused apex of the spine through many years of spinal movement during normal day-to-day life. This issue is well-known in orthopedic implant surgery. Ultimately, if a fusion does not consolidate, the implant will loosen or break. Breakage was originally observed in the original series of Harrington growing rods and has subsequently been observed in all growing rod systems. The Growing Spine Study Group has investigated this problem and has published its findings: a rod fracture rate of 15% with a re-fracture rate of 33%. The rod fracture rate is less if a dual rod over a single rod construct (11% vs. 36%) is used, presumably because the force is shared between the two rods. 10
In the case of a rod fracture, there is currently controversy as to whether fracture of one rod mandates that all of the other rods in the construct be replaced. The reasoning here is that all of the rods have undergone the cyclical loading that has led to the rod fracture and are therefore at increased risk for fracture. A recent review of the Birmingham growing rod series has revealed that 80% of second rod fractures occur in the rod that originally failed, regardless of which rods were revised and replaced after the first rod fracture. It does seem that this finding indicates unequal loading of the construct leading to repeated fractures of just one rod. Our practice now is to revise only the fractured rod when a first rod fracture occurs and to replace all rods after a second rod fracture to minimize the operative insult to the patient. 11
The final major complication related to growing rods is sagittal malalignment. It is a misunderstanding that “growing rods grow” the spine. What actually occurs is the inherent relative overgrowth of the anterior spine, which seems to be the final common pathway of early onset scoliosis / infantile idiopathic scoliosis. This overgrowth causes a crankshaft phenomenon to occur in the presence of the fixed posterior tether of the rods. Extending the growing rods then “takes up the slack” of the anterior crankshafting, increasing the vertical height of the spine and reducing the size of the curve. Children will often appear to “know” when they are due for a lengthening because they will describe the onset of pain around the spine and chest, usually in the month before surgery. This pain may be related to the relative anterior spinal overgrowth, and it is often observed that the pain is relieved by surgery. If premature spontaneous posterior fusion has occurred and anterior growth has been observed to continue, then these lead to anterior spine rotation and worsening of the deformity through a true crankshaft phenomenon. In this situation, thoracoplasty may be indicated for cosmesis in the presence of an ugly chest wall deformity.
Junctional kyphosis remains a difficult problem with the use of any posterior distraction system. Time will tell whether the systems of the new generation are any less likely than the older ones to cause junctional kyphosis. Posterior distraction can lead to significant junctional issues at both the proximal and distal ends of the construct. By the very nature of the mechanism of action of growing rods, posterior distraction is kyphogenic, especially at the proximal end of the construct. Proximal junctional kyphosis can be a significant problem above the growing spine construct, leading to hardware failure and pullout of the proximal anchors. There have been anecdotal reports of unsupported screw-only constructs pulling out backward and causing catastrophic neurologic injury through screw transection of the spinal cord. 12 Thus, there is a role for proximal hooks to protect the construct from screw pullout at the proximal foundation, either as a claw or in combination with screws.
At the end of the growing process, following the final fusion procedure and discharge of the child, there is a return to theater rates for other spinal surgery, related either to the growing rods or to other areas of the spine. If fusion does not occur soundly following definitive fusion, pseudarthrosis will be seen. This will lead to rod fracture, with the subsequent sudden onset of pain and/or the development of a palpable mass in the skin. Progression of the residual deformity may also occur, requiring revision fusion and rod exchange.
After a definitive fusion, pain distal to the instrumented and fused segments resulting from degenerative changes in the disk and facet joints can also be a reason for further operative intervention at a later date—decompressive and/or fusion surgery in adulthood. The problem of distal junctional kyphosis in posterior distraction systems is currently poorly understood, but as more children with growing rods age, it will undoubtedly come to more surgeons’ attention.
In an effort to avoid multiple surgeries to lengthen posterior growing rods, with their attendant complications, new technologies have developed to automate the distraction process with magnetic actuators. Automation has been achieved with both the Phenix (Soubeiran, France) and MAGEC (Ellipse Technologies, Irvine, California) devices. These are magnetic field–activated devices that allow interval lengthening to be accomplished as a nonoperative outpatient procedure, eliminating the need for small, repeated interval surgeries. Early experience with the MAGEC implant, as reported by Akbarnia et al, 13 has been positive and represents a significant breakthrough in the treatment of these children. However, long-term follow-up (in excess of 10 years) will be required for an accurate assessment of this and other innovative techniques in early onset scoliosis surgery and their place in the management of early onset scoliosis. Unfortunately, it currently is not possible for a child with a MAGEC implant in situ to undergo MR imaging because the device becomes heated while in the magnetic field of the scanning machine. This issue is being addressed by the manufacturers (Fig. 9.2).

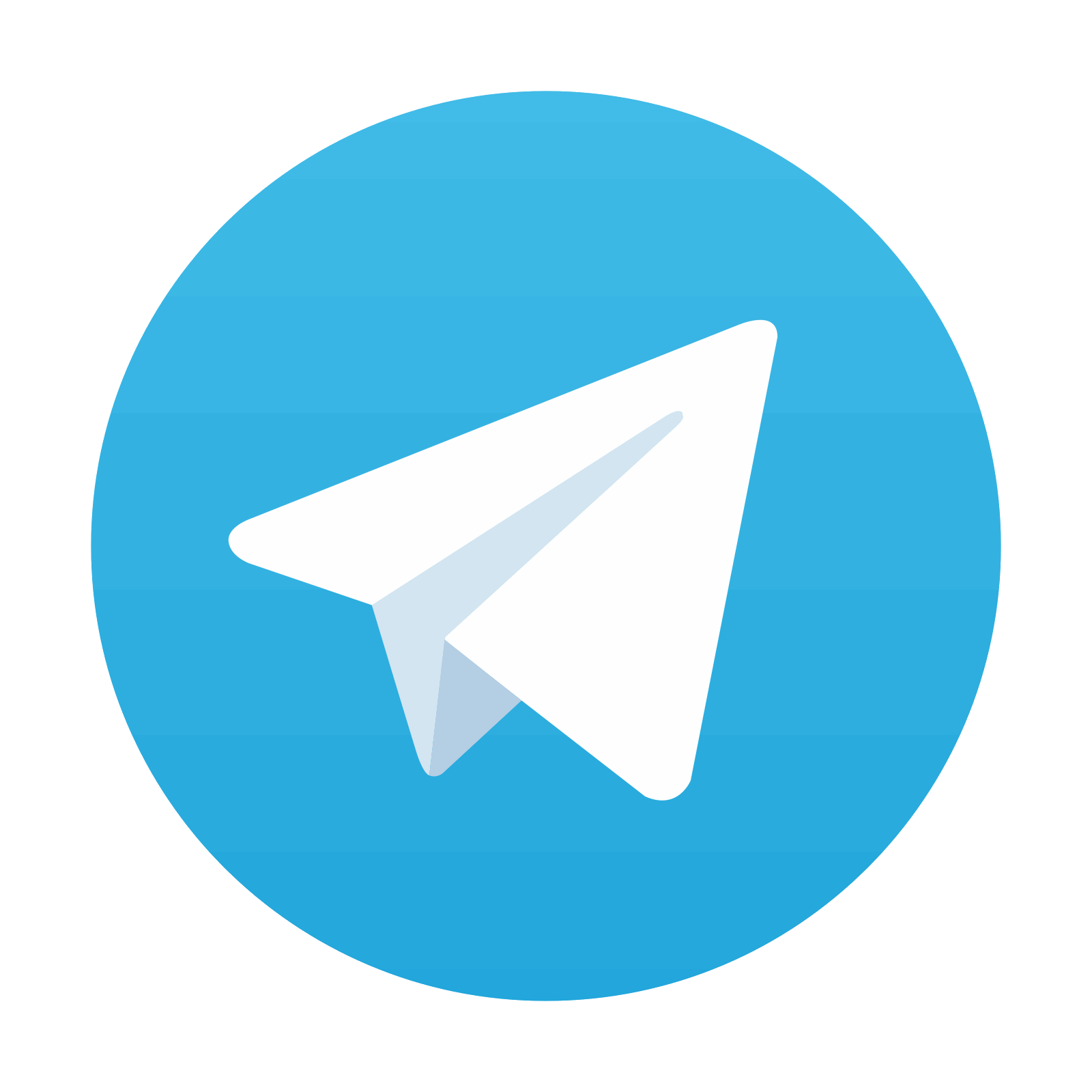
Stay updated, free articles. Join our Telegram channel

Full access? Get Clinical Tree
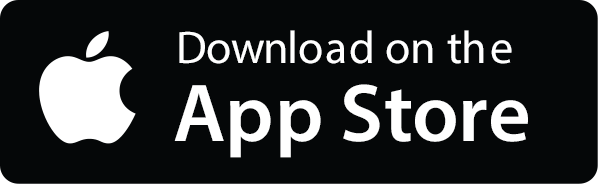
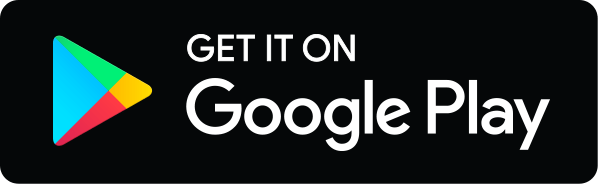
