8 The Blood Vessels and Microcirculation
8.1 Vascular Supply to the Hand from the Base to the Fingertips
With the advancement of hand surgery and techniques of vascular imaging, knowledge of vascular anatomy of the hand is mandatory. During the early embryonic period, the axial artery arises initially and then develops into the brachial artery in the upper arm and the interosseous artery in the forearm, providing the main supply of blood to the upper extremity. Next, the median (MA), ulnar, and radial (RA) arteries appear as sprouts of the arterial trunk. In the beginning, the MA, together with the interosseous artery, is involved in the majority of the blood supply to the forearm and hand; however, it dwindles after the second embryonic month and is replaced by the ulnar and radial arteries. Finally, the RA and UA provide most of the blood supply to the hand and are supported by the MA and interosseous arterial system.
The blood vessels in the hand communicate via four major arches: the anterior and posterior carpal arch at the level of carpal bones and the superficial and deep palmar arch (SPA and DPA) at the midpalmar level. These arches form three circuits on the palmar side and one circuit on the dorsal side of the hand. The dorsal arch is the source vessel for the anatomically diverse dorsal metacarpal artery system. The SPA and DPA are the most important, because they provide the blood supply to all of the fingers. The SPA represents the last segment of the ulnar artery and runs in the volar aspect of the midpalm, dorsal to the palmar aponeurosis and volar to the flexor tendons and the lumbrical muscles. Despite statements indicating that the variations in the SPA are so numerous that it is difficult to establish a specific type, a wide variety of classification systems can be found in classical anatomy textbooks and the literature. The SPA is classified between complete and incomplete types. Either the existence of an anastomosis with a contributing artery or the situation when the UA alone reaches the index finger is defined as complete arch (85%) (▶Fig. 8.1). When no presence of such anastomosis can be seen, the SPA is defined as incomplete (15%) (▶Fig. 8.2). Bilateralism of the complete SPA can be detected in 80% of patients, and the same type of arch can be found in 60% of cases. Bilateralism of incomplete SPA can be detected less frequently (4%). 1


At the adult stage, the MA sometime remains (0.9–20% of cases) as a thin artery that runs along the median nerve, frequently arising from the common or anterior interosseous artery. 2 It may terminate at the wrist, anastomose with the SPA (▶Fig. 8.3), or give off branches to the index finger and thumb at the palmar side without anastomosing with the RA or UA.

The DPA is formed mainly by the RA that passes to the dorsal side of the hand under the abductor pollicis longus and extensor pollicis brevis tendons. It then pierces the first (or, rarely, second) interosseous space and forms the DPA with the deep palmar branch of the UA (▶Fig. 8.1). The DPA does not have as many variations as the SPA but may be incomplete as well (▶Fig. 8.4) and is a source of three to four palmar metacarpal arteries.

Arterial vascularization of the palmar subcutaneous tissue is supported by palmar branches (4–12, average 7) arising from the main digital arteries. The branches anastomose with their counterparts on the opposite side within the subcutaneous tissue. There are three anatomical patterns: I-shaped (64%), with a single vessel, narrow V-shaped (23%) with a bifurcation starting at the origin of the vessel, and Y-shaped (13%), branching off beyond the origin of the vessel. 3 The small vein or veins accompanying the palmar branches drain either into the superficial longitudinal venous network or into the deep venous network accompanying the main digital artery.
Recent studies on vascular anatomy of the upper extremity demonstrate a great deal of variability in the first web space as the source of vessels for blood supply to the thumb and index finger, as well as in their communications with the ulnar-sided vessels.
At the level of fingertips, the microcirculatory network of the nail folds is accessible for in vivo examination (capillaroscopy). Capillaroscopy gives remarkable information from both clinical and diagnostic points of view. In healthy adults, the capillaroscopic pattern consists of parallel capillary loops disposed in rows and formed by an arteriolar afferent branch, a reflexion apex, and a venular efferent branch. The capillary arteriolar branch usually has a diameter inferior to the venular one. The arteriovenous ratio is about 1:5 to 1:2.
8.2 Differences between Large, Medium-Sized, and Small Arteries and Veins and the Vascular Network of the Microcirculation
The wall composition of vessels has been described as consisting of three structurally distinct layers: the intima (endothelium), media, and adventitia (▶Fig. 8.5). The structure and the thickness of each separate layer depend on the size and function of the vessel in the vascular system (▶Table 8.1). Moreover, evidence suggests that the cellular and extracellular components of these three layers are interconnected in multiple ways; thus, their traditionally distinct boundaries are blurred.

The intima , a physiological barrier between blood components and extravascular tissues, consists of endothelial cells and a basement membrane. The endothelial cells are arranged longitudinally in the direction of flow with an overall thickness of 0.2 to 0.5 µm, except the location of cell nucleus. In feeding arterioles, the endothelial cells are approximately 100 µm in length by 10 µm in width, and this 10:1 ratio is reduced as downstream arterioles diminish in diameter. The endothelial cells functionally participate in controlling vessel tone via production and release of vasoactive cytokines that influence the neighboring smooth muscle cells. Recently, the intracellular cytoskeletal components were intensively studied and it was found that they participate in the signaling pathways, causing the synthesis and release of the vasoactive factors that ultimately fine-tune the contractile state of vessels’ smooth muscle cells in response to blood flow or pressure stimuli. The shear forces caused by blood flow induce the stress fiber formation and the stabilization of microtubules, allowing the polarization of the endothelial cells, with the microtubule-organizing center redistributed to the downstream side of the nucleus. The endothelial cells within the intima may vary phenotypically. Between the typical endothelial cells, the endothelial progenitor cells can be found; they are able to transdifferentiate into mesenchymal cells with smooth muscle phenotype.
The basement membrane under endothelial cells is approximately 0.1 µm in thickness and consists primarily of collagen type IV, laminin, and heparan sulfate proteoglycans. Additional components include collagens type I, III, and V, and fibronectin. Besides its primary function, which is to provide anchoring support for the endothelium, basement membrane exposed to blood components after injury provides signals for the recruitment and migration of cells across the vessel wall. 4
The media consists of cells and the extracellular matrix (ECM). The ECM consists of lamellae of elastic material with intervening layers of vascular smooth muscle (VSM) cells, collagen fibers, and ground substance. The ECM is responsible for the passive mechanical properties of the arteries. In the proximal aorta, elastin is the dominant component of the ECM, whereas in the distal aorta and its branches the collagen-to-elastin ratio is reversed, with a predominance of collagen in peripheral muscular arteries. The proportion of elastin and collagen type I and type III differs markedly between species and has a substantially differential mechanical effect on the stiffness and distensibility of the vessel wall. Additionally, since the structure of ECM is determined at a very young developmental stage and thereafter remains quite stable, several neurohormonal factors as well as chemical modifications (i.e., glycation, cross-linking) may modulate the properties of the ECM during the subject’s life. The morphology supports the main role of the very proximal part of vascular system (i.e., aorta and its major branches), which is to serve as a temporal buffer for the blood during the ejection phase of the heart.
In the distal arteries and at the level of microcirculation, VSM cells largely predominate within the media, forming one or more cell layers (i.e., one or two layers in feed arterioles). The VSM cells control vascular diameter via cell contraction and relaxation processes. The VSC cells are spindle-shaped, with an average length of approximately 100 µm, arranged perpendicular to the longitudinal axis of the vessel in a circumferential fashion. The cells wrap around the vascular circumference and maintain their position but sometimes overlap each other transversally to the longitudinal axis of the vessel wall. The population of VSM cells is not homogenous. They may have different mixtures of phenotypes, not only with contractile and synthetic but also with proliferative and apoptotic behavior. The occurrence of each of the phenotypes depends not only on age but also on location in the vascular tree and prevailing conditions. Moreover, the embryonic origin of the different VSM cells is different in various parts of the vascular tree. In the avian abdominal aorta and small muscular arteries, the VSM cells are of mesodermal origin, whereas those of the aortic arch and thoracic aorta are mainly derived from the ectodermal cardiac neural crest—essential in the formation and organization of elastic laminae and tensoreceptors in the great vessels. 5 In the microcirculation network, the VSM cells are created during embryogenesis, as a result of complex angiogenesis in hypoxic conditions.
In arterioles, besides the VSM cells, an internal elastic lamina can be found. The internal elastic lamina is a sheet of approximately 0.3 µm in thickness consisting primarily of degradation-resistant elastin molecules. The lamina is not present in all arterioles but is supposed to provide the recoil properties of the vessel wall that are important in dealing with pulsatile blood pressure. Interestingly, the internal elastic lamina appears wavy with evenly distributed ridges, which suggests that under physiological conditions it does not contribute significantly to the viscoelastic characteristics of the vessel wall. Additionally, on scanning micrographs, small fenestrae can be observed within the solid structure of the internal elastic lamina. The fenestrae grow in size and number during development, enable the transport of molecules from the blood to the media and extravascular tissues, and allow for direct contact between endothelial and VSM cells. 6 Importantly, the size and number of these holes have been shown to change in response to different stimuli, which potentially makes the structure able to remodel rapidly.
The adventitia consist of some fibroblasts, not surrounded by the basement membrane, embedded in an ECM made of thick bundles of collagen fibers oriented along the longitudinal axis of the vessel. Some cells in the adventitia considered as the fibroblasts are probably stem mesenchymal progenitor cells. Within adventitia of the arterioles, the nonmyelinated nerve endings are also present at a distance of approximately 5 µm from the outermost VSM. 7 Additionally, the elastic fibers arranged with a longitudinal pitch are also present, especially in the expandable tissues such as skeletal muscles. Historically, the adventitia was considered as a structural support for the vessel and a scaffold for the anchoring of nerve endings. Currently, it is suggested that the fibroblasts contribute to the plasticity of the arteriolar wall (modulation of VSM activity), participate in production and remodeling of the ECM compounds (TGF-β, endothelin-1, α-actin), generate inflammatory signals, and potentially contribute to the generation of tensile force. In response to injury, the fibroblasts can transform to myofibroblasts and perhaps into smooth muscle cells, which play crucial role in the vascular repair.
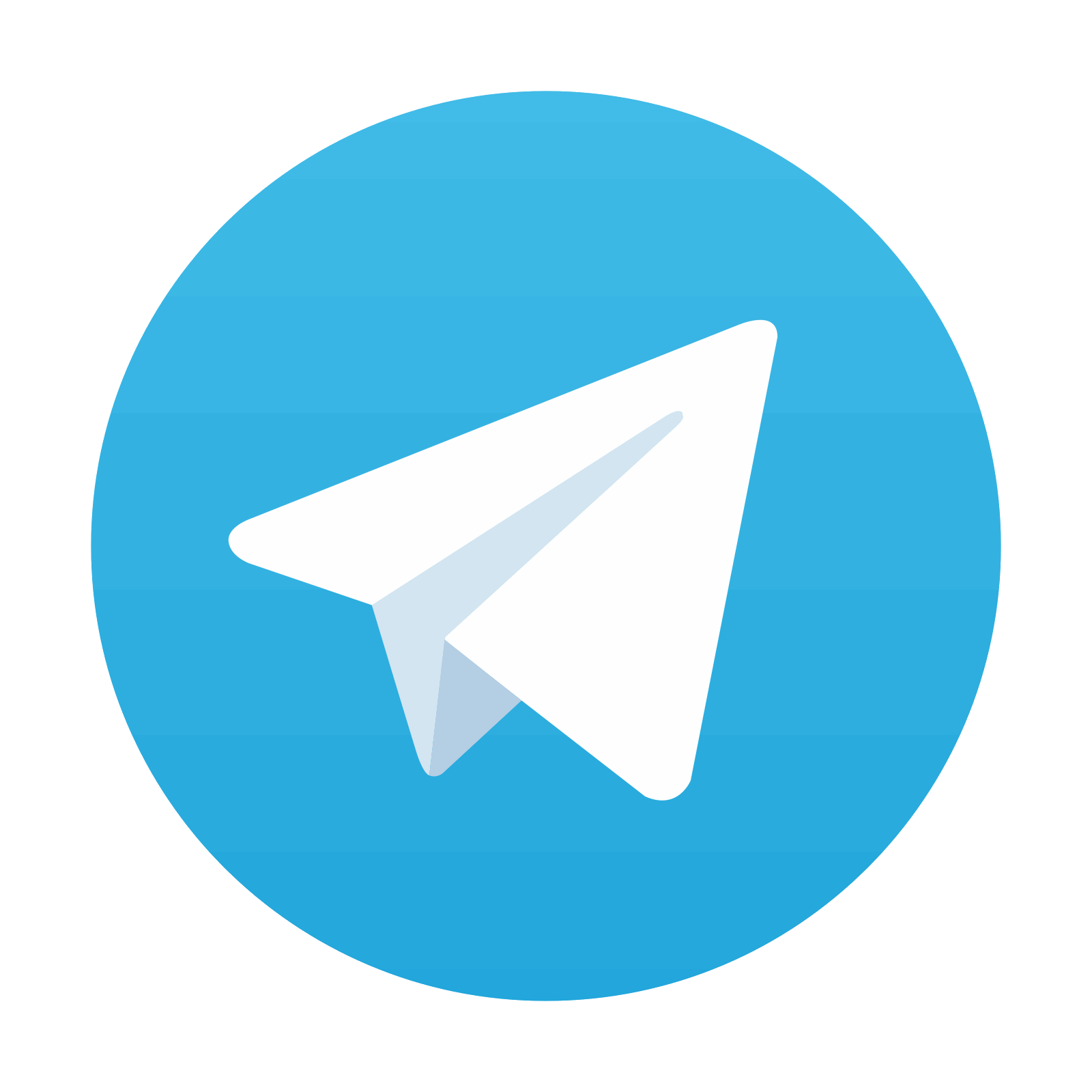
Stay updated, free articles. Join our Telegram channel

Full access? Get Clinical Tree
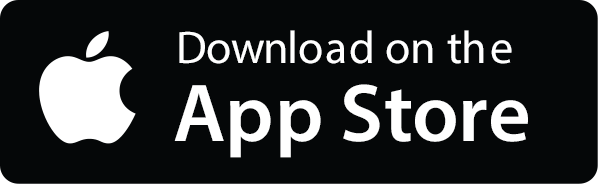
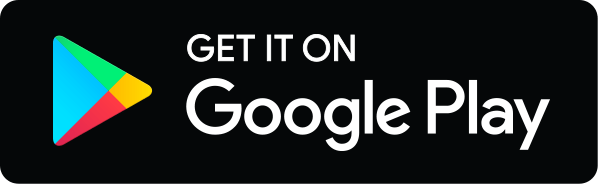