6.6.2 Femur, shaft (including subtrochanteric fractures)
To access the videos, please follow the URL link
1 Introduction
Femoral shaft fractures are markers of high-energy transfer to the body in skeletally mature individuals with healthy bone. Associated injuries are common as femoral fractures are frequently part of polytrauma and patients may develop the systemic inflammatory response syndrome. This can occur even in isolated femoral fractures. While the standard treatment for most femoral shaft fractures is undisputedly reamed, locked, antegrade intramedullary (IM) nails, there are many open questions related to treatment of femoral fractures. Some of these questions involve intraoperative positioning, direct and indirect reduction techniques, treatment of concomitant fractures of the femur and timing of surgery to avoid systemic complications.
1.1 Epidemiology
A recent population-based, prospective epidemiological study described that femoral shaft fracture incidence is 10–37 fractures per 100,000 population per year in a mixed urban/rural region [1]. The distribution of prehospital deaths, high-energy mechanism and low-energy mechanism was 17%, 48%, and 35%, respectively. The age distribution is typically trimodal with relatively low peak among children followed by a high peak of young adults and an increasing peak among patients older than 65 years. This latter group is due to osteoporosis, complications of osteoporosis treatment (bisphosphonates), periprosthetic fractures, and increased activity of senior citizens [2].
1.2 Special characteristics
The femur is the largest long bone in the body and generally heals at a high rate, probably because of a good blood supply from circumferential muscles. There are various associated injuries, such as ipsilateral femoral neck fracture, ipsilateral intraarticular distal femoral fractures, knee ligament and meniscal injuries, ipsilateral tibial shaft fractures (floating knee), and polytrauma. Femoral fractures are associated with systemic complications and malunion is frequent. Bisphosphonate-associated fractures of the femur are a new entity and have a unique pathophysiology [3]. Pathological fracture of the femur from metastatic disease is also increasing as the population ages.
2 Evaluation and diagnosis
2.1 Case history and physical examination
The trauma patient with a potential femoral fracture must be evaluated in a systematic manner with a primary and secondary survey following advanced trauma life support (ATLS) principles. There may be associated intracranial, spinal, chest, abdominal, and pelvic injuries with sources of bleeding beyond the femoral fracture. Isolated, closed femoral fractures are associated with up to 1.5 L of blood loss during the first 48 hours.
As an isolated injury, the femoral shaft fracture is not generally the cause of hemorrhagic shock in an otherwise healthy patient with a short prehospital time. Thus, other sources of bleeding (chest, abdomen, pelvis, open wounds, and other fractures) should be considered in patients with hemodynamic instability.
Open femoral fractures and bilateral femoral fractures can cause shock and exanguination, especially in coagulopathic patients. Early traction and local compression is essential to reduce bleeding. Patients with bilateral femoral shaft fractures have a higher mortality rate and are more likely to have other systemic injuries (5.6% versus 1.5%) [4].
There are few crucial laboratory tests that indicate the (patho-)physiological response to injury and resuscitation and the potential for unfavorable outcome (extended intensive care unit [ICU] stay, multiple organ failure, sepsis, or death). Metabolic acidosis on the arterial blood gas (base deficit and lactate are the best surrogates) is a good marker of hemorrhagic shock and tissue hypoperfusion; sequential measurement helps monitor the response to resuscitation. These parameters are more reliable and specific than blood pressure and hemoglobin concentration. Trauma patients who do not clear their metabolic acidosis by 24 hours, those who have low initial platelet count and elevated creatinine are more likely to develop severe systemic inflammatory response syndrome and organ failure.
Recent data shows that the most frequent cause for blood transfusion during the first 24 hours after injury in level I trauma centers primarily dealing with blunt trauma are fracture and musculoskeletal injury.
Associated femoral neck and shaft fractures and knee ligament injuries must be carefully investigated. When focusing on the femoral fracture itself, the soft-tissue injury (eg, closed degloving, open fracture wound, lacerations) and the neurovascular status of the limb must be carefully assessed.
2.2 General condition of the patient
There are many theoretical and arbitrary classifications available in the literature, although few have been validated. Most frequently patients are categorized as “stable”, “borderline”, “unstable”, and “in-extremis”. These categories are based on dozens of variables, which are not independent from each other. From a practical point of view, the key decision is whether to perform early total care with definitive fracture fixation within 24 hours or damage limitation with temporary (external) fixation as soon as possible. Thus, in practical terms there are only two categories. The situation is dynamic and decision making is based upon the timeline of events, associated injuries, and the physiological condition of the patient, comorbidities, logistics, and resources.
2.3 Imaging
Good quality preoperative imaging is essential for planning femoral fracture stabilization and for the exclusion of proximal or distal femoral fractures that require operative intervention at the time of the stabilization of the femoral shaft.
The x-ray examination should consist of x-rays in two orthogonal planes. The x-rays must include the joint above and below the fracture. The most commonly missed associated fractures of the femur, which require operative management, are the femoral neck fracture (31B) and distal femoral partial articular fracture (Hoffa, AO/OTA 33B3.2). These fractures can often be undisplaced or overlooked due to more obvious and potentially life-threatening injuries. Preoperative imaging can be improved by an x-ray view with 15° of internal rotation of the femoral neck, a perfect lateral view of the knee joint, careful evaluation of the trauma computer tomographic scan and by looking for occult fractures on the image intensifier at the time of surgery.
3 Anatomy
3.1 Key anatomical features
The femoral shaft is not straight and has a radius of curvature of approximately 1.5 m. There are lateral cortical flares at the proximal and distal metaphysis and these features must be considered when long plates are used: contouring will be required.
3.2 Blood supply to the femoral shaft
The blood supply of the femoral shaft is derived from two main sources. The inner two-thirds of the cortex and medulla derives its blood supply from the nutrient artery which arises from the second perforating femoral artery and enters the bone proximally and posteriorly along the linea aspera. The outer one-third of the cortex is supplied by the periosteal arteries that are derived from the surrounding muscles supplied by the perforating arteries ( Fig 6.6.2-1 ). Following displaced fractures, the circulatory pattern of the femoral shaft is drastically altered due to disruption of the medullary blood supply. However, the periosteal blood vessels are seldom extensively stripped because of their perpendicular orientation to the cortical surface. Until the recovery of the endosteal circulation, the periosteal vessels are the main source of blood supply around the fracture zone. This highlights the importance of preserving the periosteal vessels and the perforating arteries.

4 Classification
4.1 AO/OTA Fracture and Dislocation Classification
The AO/OTA Fracture and Dislocation Classification classifies diaphyseal femoral fractures and fractures of the subtrochanteric segment into the 32 region (chapter 1.4). This classification is not different to any other shaft fracture classification. Within the boundaries of the diaphysis, the fracture pattern does not really change the method of fixation (eg, reamed, locked IM nail). The classification can help preoperative planning for direct and indirect reduction techniques as well as predict difficulties in achieving anatomical rotation and intraoperative complications. It can indicate associated soft-tissue injury and blood loss and may predict risk for delayed union ( Fig 6.6.2-2 ).

5 Surgical indications
Nonoperative treatment of femoral shaft fracture by traction or cast brace has unacceptable outcomes and should only be used when surgery is not an option. Traction is used for temporary stabilization until definitive fixation can be performed. Most femoral shaft fractures are treated using IM nails. Plates may be used when there is an associated metaphyseal or articular fracture and for periprosthetic fractures. Temporary external fixation is used for damage limitation surgery and sometimes in the management of infected nonunion.
There are no absolute indications for retrograde nails but relative indications are:
Obesity (it is difficult to find the correct antegrade entry point)
Ipsilateral femoral neck and shaft fractures
Ipsilateral femoral and tibial shaft fractures (one incision for both IM nails) ( Fig 6.6.2-3 )
Multiple injuries (the surgeon can prepare and drape other extremities, abdomen, and chest with the patient supine on the radiolucent table)
Bilateral femoral fractures (one set up in supine position on radiolucent table)
Unstable spine injuries
Pregnancy (minimization of the fetus’ exposure to radiation)
An uncontaminated traumatic knee arthrotomy
Ipsilateral pelvic and/or acetabular fractures (antegrade nail incision might interfere with subsequent incisions)
Severe soft-tissue injuries or burns at the antegrade nail starting site
Preexisting proximal femoral hardware ( Fig 6.6.2-4 )


Relative indications for femoral plating include:
Type 32B and type 32C fractures, with proximal extension into the trochanteric area or distal extension into the condylar area
Children older than 8 years with open epiphyses, or when there is no possibility of using elastic nails to provide stability
Fractures with a narrow or deformed IM canal
Fractures with associated spinal or pelvic fractures (further damage may be caused by using a fracture table for femoral nailing)
Fracture in association with abdominal injury that requires a laparotomy (can proceed for plating on a normal operating table at the same time)
Fracture with associated vascular injury requiring repair
Periprosthetic or periimplant fractures
Ipsilateral neck and shaft fractures when the neck fracture is treated using a separate implant
Fractures associated with severe lung contusion where immediate IM nailing may be high risk
6 Preoperative planning
6.1 Timing of surgery
Before the 1970s, stabilization of long-bone fractures, including the femur, was often performed after a delay of a few days to allow time for “stabilization” of the patient. It has become clear that early fixation of long-bone fractures reduces patient morbidity and mortality, especially in the severely injured patient [5] (see chapter 4.1). Timely fixation of femoral fractures reduces chances of remote organ injury, improves outcomes of severe brain injury and makes less likely all immobilization-related complications [6]. This stabilization can be with temporary external fixation or with definitive nails or plates.
There is no absolute rule for the time frame of fixation but it should be performed at the earliest safe time point after prompt resuscitation (optimized patient condition, optimal resources, and personnel available) and usually within 24 hours from injury. The patient is considered resuscitated if there is no clinically significant metabolic acidosis (base excess less than 4 mMol/L [trending down] and lactate is less than 2 mMol/L). Severe head and chest injury can be a reason for staged care of femoral fractures (damage control with primary external fixation followed by secondary IM nail) but also serves a good argument for early definitive care to optimize patient positioning, and better ventilator and intracranial pressure management [1]. In general, the decision on early definitive fixation or damage control depends upon the physiology of the patient rather than the anatomical injury.
Temporary external fixation has been shown to lead to less blood loss and reduced operating time compared with immediate IM nailing or plating. This intervention can be safely converted to IM nail fixation within 2 weeks after injury [7, 8]. Staged care, with initial external fixation and later IM nail, is shown to reduce interleukin-6 levels at both stages compared with primary IM nail [9]. Its clinical relevance in the modern era of judicious hemostatic resuscitation is unknown. It is proven that unnecessary overuse of the damage-control approach (application to uncompromised or borderline patients that can be resuscitated) leads to increased ICU stay, ventilator days, and higher infection and complication rate compared with early total care [10].
6.2 Implant selection
6.2.1 Intramedullary nailing
The closed reamed interlocking IM nail is the gold standard for femoral shaft fractures. It provides relative stability for fracture healing by callus formation. With minimally invasive techniques, this can result in less soft-tissue injury, less disturbance of blood supply, less blood loss, and a lower infection rate. Intramedullary nails are load-sharing implants and early weight bearing is recommended; implant failure is uncommon. The surgeon must know the design characteristics of each nail and special surgical techniques, eg, trochanteric entry, piriformis entry.
Retrograde or antegrade nailing
Intramedullary nails can be introduced in an antegrade or retrograde manner. Antegrade nailing is the gold standard (see chapter 3.3.3) but retrograde nailing has clear advantages in certain scenarios (see section 5 above). Despite potential drawbacks from the intraarticular entry point in the knee with retrograde nailing, antegrade nailing has no better clinical outcomes than the retrograde technique [11, 12]. With antegrade nailing, patients must be warned of the risk of hip pain at the entry site and with retrograde nailing, patients must be warned that there is a risk of knee pain at the entry point.
Reamed versus unreamed procedure
Femoral nails can be inserted either after reaming of the medullary canal or without reaming (“reamed” or “unreamed” IM nails) (see chapter 3.3.1). The union rate of the unreamed technique is lower and these patients require more secondary procedures than with management using reamed techniques [13]. Originally, reaming was considered responsible for lethal pulmonary embolism after IM nailing in severely injured patients. Recent studies [14] have shown that the severity of the pulmonary injury and the quality of resuscitation are probably more decisive for the development of pulmonary dysfunction than the fixation device or insertion technique (see chapter 4.1). The potential complications of reaming are further reduced with the utilization of sharp, deep fluted reamer heads and 0.5 mm increments in reaming. The reamer-irrigation-aspiration (RIA) system was developed to address the elimination of marrow particle and inflammatory mediator loading of the systemic circulation but it is almost exclusively used in elective and semi-elective situations for bone graft harvesting and reaming/irrigating of infected medullary canals.
6.2.2 Plating
Plating of the femoral shaft as a primary treatment has limited indications (see section 5 above). In general, a 4.5 broad plate is recommended and precurved plates to match the bow of the femur are helpful. For diaphyseal fractures extending into the distal metaphysis and joint, the locking options provided by the distal femoral locking plate (LCP-DF 4.5) may be helpful. For complex diaphyseal fractures that extend proximally to the intertrochanteric region, the 95° angled blade plate remains a useful option.
Open femoral stabilization
Open compression plating of the femoral shaft is rarely indicated in the acute setting. In terms of implant selection and technique, long broad LCPs 4.5 are recommended with at least eight cortices of fixation at either side of the fracture. Whenever it is possible, lag-screw insertion perpendicular to the main fracture line is advisable, preferably through the plate. In transverse fractures, a prebent plate, the articulated tensioning device and dynamic compression through the plate are all essential parts of the correct technique.
Minimally invasive plate osteosynthesis
Recently, there has been enthusiasm for plate fixation of multifragmentary fractures extending into the metaphysis using an indirect reduction and percutaneous, submuscular plate insertion through small incisions proximal and distal to the fracture site. Experimentally, this leads to less damage to perforator vessels and the periosteal blood supply [15]. The advantage of the percutaneous bridge plating technique is better preservation of blood supply to the fracture fragments with early callus formation due to relative stability. The technique also leaves the endosteal circulation undisturbed, which is responsible for two-thirds of the diaphyseal blood supply. The disadvantage of this technique is a higher risk of malunion with inexperienced surgeons [16]. The latest plate generation featuring the LCP is well suited for minimally invasive osteosynthesis (see chapter 3.1.3). Preoperative planning should include selection of appropriate length and type of plate, assessment of precontouring, the type, location, and number of screws to be used and their order of insertion ( Fig 6.6.2-5 ).

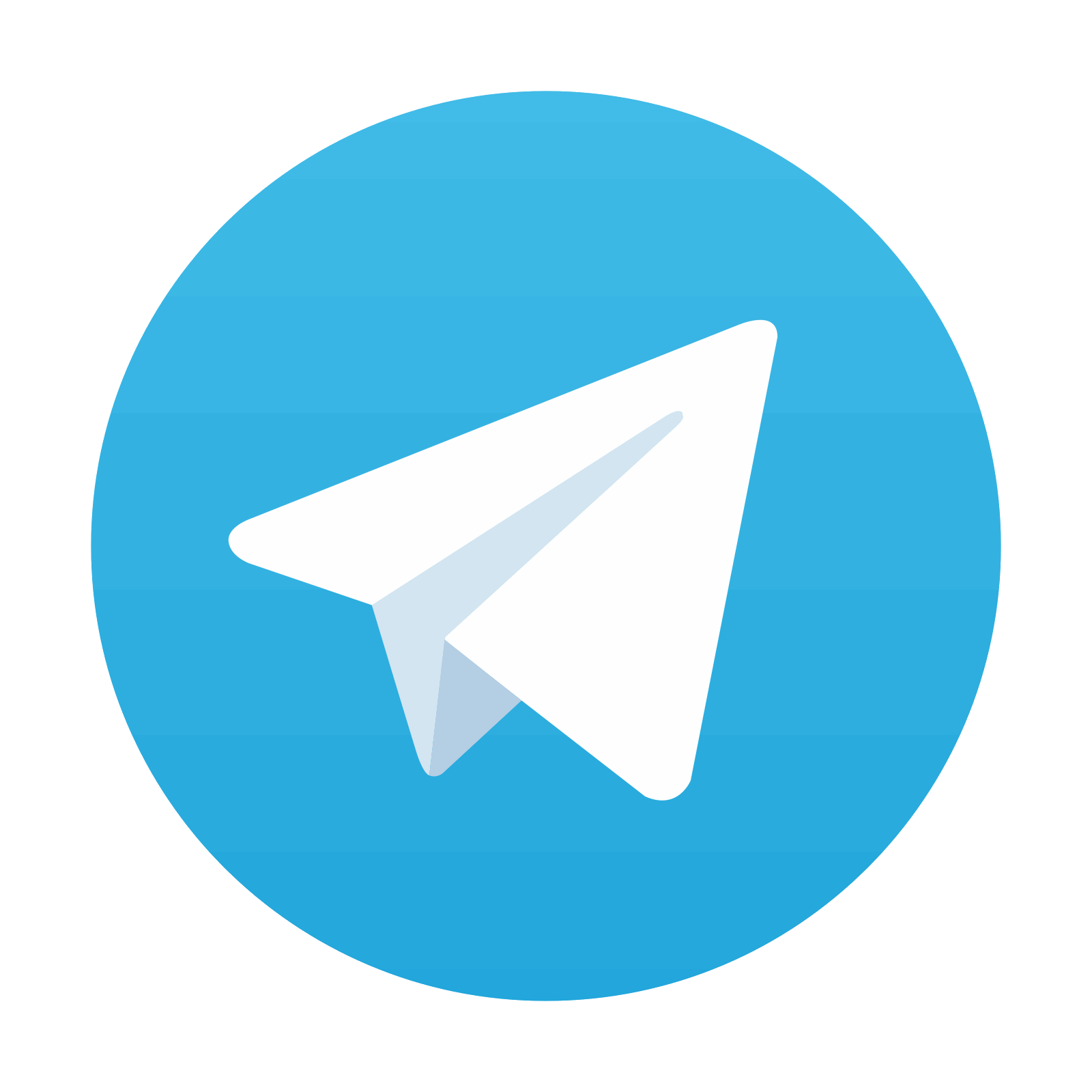
Stay updated, free articles. Join our Telegram channel

Full access? Get Clinical Tree
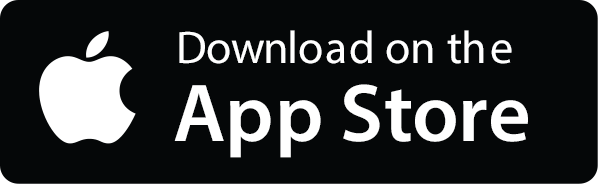
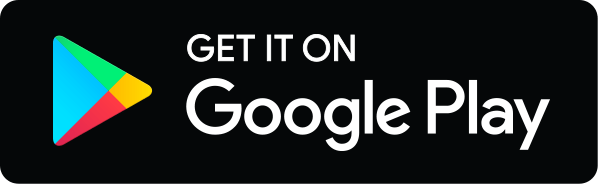
