5 Three-Dimensional Carpal Imaging of the Scaphoid
The shape of the scaphoid creates challenges in injury diagnosis, deformity correction, and fracture fixation. The crucial relationships of the scaphoid with the adjacent carpal bones to achieve a stable wrist further complicates the process of detecting a possible osteoligamentous disruption. An adequate understanding of the scaphoid osteology and the relationships of the scaphoid with the surrounding carpal bones is vitally important to achieve a satisfactory therapeutic outcome following injury.
Surgeons naturally think three-dimensionally; however, many medical technologies like radiographs and ultrasonography impose two-dimensional restrictions that limit the ability to diagnose and treat patients effectively. A three-dimensional (3-D) appreciation of the anatomy and the injury is crucial to effectively make the correct diagnosis and deliver the appropriate treatment.
This appreciation in 3-D can be conveyed in basically two ways:
Stereoscopically—in which different images of the object are delivered to each eye, as in viewing an actual object
3-D simulation—in which the same image is seen by both eyes, but an impression of 3-D shape is conveyed by using 2-D “tricks,” such as shading, shadows, and motion
Computer screens, hard copy paper, or film can only display a 2-D image; therefore, the impression of depth and contour must be simulated by shading, colors, and shadow, as well as motion.
Stereoscopic 3-D vision from a 2-D image, as distinct from a 3-D object in space, is possible by using 2-D visual tricks, such as “parallel viewing” and “cross-eye viewing” ( Fig. 5.1 ) (e.g., in the Magic Eye images), or by using polarizing or shutter glasses and special computer screens and viewing systems.
In addition to an appreciation of the 3-D anatomy of the object in space, it is also important to consider the ability to manipulate a 3-D volume data set and so create a variety of diagnostically useful reformatted images. The initial step in the imaging process is to capture a volume data set of the anatomical region. The basic unit of data is the voxel (volume pixel). This is the 3-D version of the 2-D pixel, and contains position data of the point clouds representing the object in space as well as a radiodensity value (usually as a Hounsfield unit). The utility of 3-D scaphoid imaging is derived from the varied ways one can manipulate and display this information.
The loss of bone integrity is usually the critical factor in scaphoid injuries. Because the boundaries between the scaphoid and other tissues are best identified with computed tomography (CT), most of the discussion will relate to the manipulation of the CT-captured volume data. Although magnetic resonance imaging (MRI) can provide valuable information regarding bone quality and vascularity, the ability to manipulate the 3-D data is less applicable to MRI than to CT at this time, although many of the image data manipulation techniques are the same.

▪ Three-Dimensional Image Data Display
Major advances have been made in the image data acquisition technology for CT with the introduction of slip ring technology and faster processing capacity. It is now possible to acquire a volume data set with very fine spatial representation,1 which can then be converted to a variety of 3-D images.
Primary slice display—Because of changes in CT data capture with helical scanning, a volume of data is captured, whereas in previous CT technology a series of slices was captured. To transfer the data set to a workstation, however, the block of volumetric data must be converted into axial or transverse slices of variable thickness ( Fig. 5.2 ).
Multiplanar reformation (MPR; Fig. 5.2 )—This is a technique wherein the axial slices are stacked to allow the display of a variety of planes in any direction or orientation. With higher-resolution CT data capture, the voxels have equal sides (i.e., they are close to an actual cubic shape or isotropic), and thus the image clarity and resolution for any given plane will be as good as those of the original axial slices. This contrasts with older-generation CT2 scanners or MRI, in which the voxels are nonisotropic.
Curved plane reformation—This technique creates a longitudinal viewing plane that follows the length of any particular anatomical structure. It is most applicable in vascular scanning because it can straighten the vessel to give a better appreciation of any compression or narrowing.1
Maximum-intensity projection (MIP)—In this display technique, only a certain value of voxels in a defined density range is displayed on the 2-D screen. This is useful for creating the appearance of a plain radiograph, or showing metal or angiographic studies ( Figs. 5.3 and 5.4 ). A minimum-intensity projection (mIP) can also be created. This imaging technique is more useful for identifying low radiodense areas, such as air, and does not have much value in assessing the wrist.
Volume rendering (VR)—In this technique, each voxel in the 3-D volumetric data set is given a particular color and transparency depending on its value; this is then projected onto a 2-D screen ( Fig. 5.5 ). There is no actual 3-D model created, only a projection of the cumulative values through a series of virtual rays of light (ray casting) to create a type of “colored shadow” on the viewing screen. The aspect of viewing and the position of the virtual cameras can be altered, but the images can be saved only as a series of screen captures, or movies. There are limitations in terms of measurement, image manipulation, and segmentation (separation of various parts of the image). The only way to allow the clinician to review the 3-D images in an interactive way with a VR technique is to reimport the entire data set, then reapply the initial settings to re-create the previous 3-D image. This is usually possible only on a dedicated workstation because of the large memory demands and specialized software required.
Surface shading—This is a type of VR in which only specific voxels on one surface of an image object are given an opacity and color ( Figs. 5.6 and 5.7 ). In this instance only the voxels of certain values closest to the viewing position are displayed. The voxels corresponding to the threshold value of the surface to be visualized, be it bone or contrast-containing vessel, are displayed as an opaque surface facing the selected viewing direction. This creates a virtual surface, which conceptually can be seen as an angled ray of light that illuminates the closest surface. The viewing position can be varied, while the impression of contour is accomplished through shading.
Surface rendering—This is distinct from VR or surface shading in that by using threshold values of radio density, boundaries are identified between certain anatomical structures (e.g., bone and soft tissue) with edge detection software. From this, an actual 3-D model can be created that is composed of a series of polygonal meshes ( Fig. 5.8 ). The object can be saved and the primary voxel data discarded. This has major advantages for the surgeon because the object can be manipulated and viewed by the clinician without needing to reimport the entire data set (as would be needed with VR), and it can be measured, manipulated, and utilized for virtual surgery.






Only mesh rendering creates an object on which the clinician can perform secondary segmentation and virtual manipulation. It is a misnomer to refer to 3-D segmentation if using a VR image creation technique from the volumetric data set because this implies that the 3-D objects are actually segmented. In reality, no 3-D object is actually created, and the separation occurs in two dimensions on images projected onto the screen. VR selects voxels for protection but does not segment a 3-D object. Surface (mesh) rendering, on the other hand, does create a specific 3-D model, which can therefore be secondarily segmented and manipulated.
This is a very confusing area for most clinicians because most current radiology “3-D” imaging uses a VR technique that simply projects the data within a 3-D working space onto a 2-D screen. The visualization of those data points in space can be provided with variable transparency and color to create a very complex visualization of the entire 3-D data set. The critical feature is that with VR no actual 3-D object is created—it is simply a projection of the sum total of those data points in the line of sight onto the 2-D screen. It is akin to viewing the shadow cast by a tree onto an opaque window, but then being able to provide variable colors to the actual shadow. It is not possible to interact with the data set itself, and all that is provided is simply a screen capture of the shadow. In essence, the image provided has as much substance as a hologram, and although excellent visualization can be achieved, it is not possible to specifically interact with the image itself.

There are therefore many ways to manipulate and visualize the 3-D data set, and each has a particular utility in the assessment of carpal and more specifically scaphoid injuries.
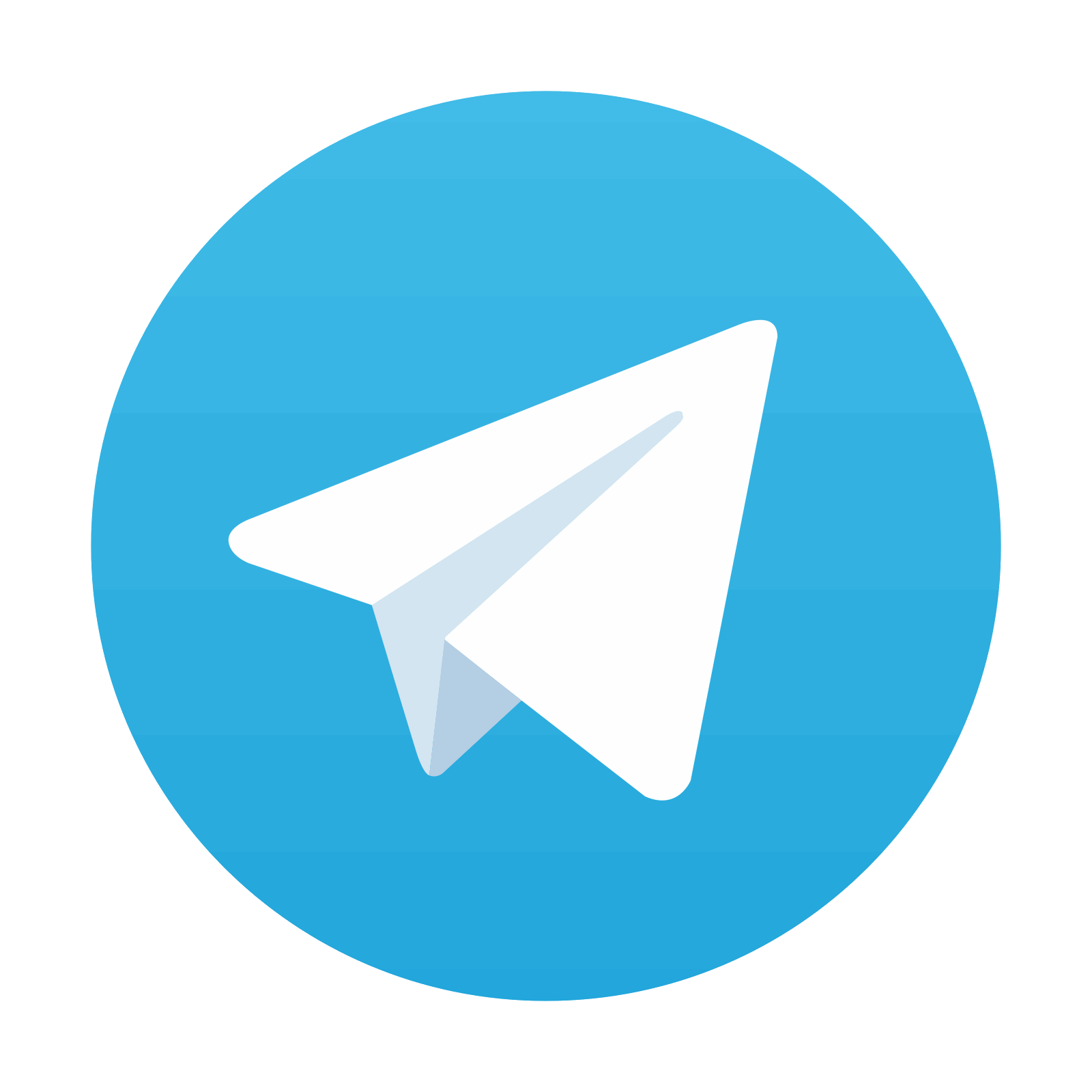
Stay updated, free articles. Join our Telegram channel

Full access? Get Clinical Tree
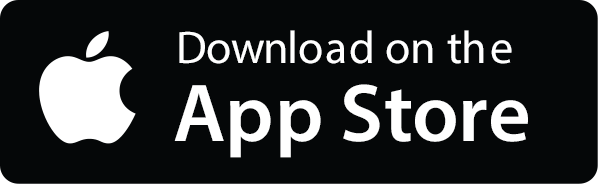
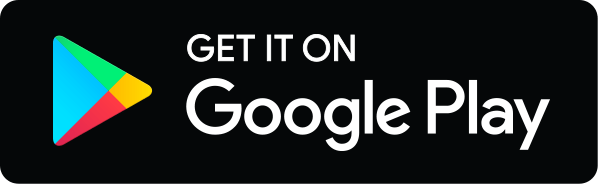