5 The Hand and the Brain
5.1 Introduction
The everyday use of hands is highly dependent on the interaction between the sensory and motor systems in the central nervous system (CNS). The interaction of sensory systems, such as those for vision, hearing, and somatosensory function, can help in identifying external objects and the body’s relationship to them and thereby create an internal representation of the external world in the brain. This internal representation is the framework used by the motor system in the CNS to plan, coordinate, and execute motor programs used to guide purposeful movement of different body parts, such as the hand. 1 – 3 Furthermore, it is through the moving hand that a large diversity of tactile experience is unveiled; hence, the moving hand is a facilitating agent for more complex tactile experiences. Because many motor and sensory acts of daily life are unconscious, we are often unaware of their complexity. However, this complexity becomes apparent when a person sustains an injury to the nervous system, such as a median or ulnar nerve injury in the forearm, often resulting in a permanently impaired somatosensory function as well as decreased motor function in the hand. 4
The CNS in general and the brain in particular have a tremendous motor- and sensory-related capacity, controlling some 640 different muscles in the body and somatosensory information from these muscles and the skin. This is made possible by the large amount of interacting nerve cells and nerve tracts in the brain (▶Fig. 5.1).

Anatomically, the brain is composed of macroscopically symmetric regions: prosencephalon (diencephalon and telencephalon), mesencephalon, and rhombencephalon (medulla oblongata, pons, and cerebellum). The most caudal and in many respects the simplest part of the CNS, the spinal cord extends from the base of the skull to the first lumbar vertebra (▶Fig. 5.2). The spinal cord is divided into a central core of gray matter, containing the nerve cell bodies, and an outer layer of white matter, containing ascending and descending tracts of myelinated axons.

Nerve tracts in the CNS representing motor and sensory control are bilaterally symmetrical and, if connecting the brain to the spinal cord, cross to the contralateral side of the spinal cord or brain. Thus, motor and sensory control of one hand is mediated mainly by the contralateral brain hemisphere. Although the CNS is often described in anatomical terms, its functional organization is much more complex and different anatomical brain areas contribute to various functional systems. The primary motor cortex, located in the precentral gyrus of the frontal lobe, and the primary somatosensory cortex, located in the postcentral gyrus of the parietal lobe (▶Fig. 5.3), contain large numbers of different highly specialized neurons processing information before sending it on to other areas inside the brain or to the spinal cord and on to the muscles. Already in the end of the 19th century and in the first half of the 20th century, cerebral somatosensory and motor maps were characterized in great detail by several scientists, including Charles Sherrington and Wilder Penfield. 5 They showed that the hand and face, including the tongue, are represented in sensory and motor cortex by very large areas, reflecting the large number of nerve cells needed for fine motor control and somatosensory processing in these body parts compared to, for example, the legs or torso (▶Fig. 5.3).

Furthermore, tremendous advances in neurobiology and in functional neuroimaging have shown that both the motor and the somatosensory systems are much more complex than first assumed. A seemingly simple task such as grasping a glass of water for drinking involves a complex network of several different cerebral areas working together in a highly orchestrated manner to achieve the goal. A key issue in understanding the working hand is knowledge of how the motor and somatosensory systems interact and how information from sensory receptors in the skin, joints, and muscles is used for appropriate motor control. Understanding this is not only fundamental in its own but also of importance in understanding disorders in these systems and their treatment.
5.2 Motor System
The anatomy of the muscles controlling the human hand places special needs on the system responsible for control of voluntary movement. Many muscles controlling hand and finger movement are located in the forearm or proximal hand, and they have long, sometimes multiple, tendons crossing several joints and sometimes acting on multiple fingers. Thus, control of hand and individual finger movements requires a complex pattern of neural activation and inhibition of several muscles.
Movement in the upper extremity is generated by stimulation of skeletal muscle fibers by lower motor neurons, with their cell bodies located in the anterior horn of the spinal cord. Activity in the lower motor neurons is coordinated by interneurons forming local circuits in the spinal cord. The activity in these local circuits is in turn governed by input from descending projections from upper motor neurons located in the cerebral cortex and brainstem 1 , 2 and also by feedback from somatosensory nerves.
The primary motor cortex has a distinct order, somatotopy, where neurons controlling the different body parts and patterns of movement are located together. However, the neurons are not equally divided between the different body parts; instead, a disproportionally large area, which is to say a large amount of nerve cells of the primary motor cortex, is devoted to muscular control of the hand compared to the lower extremity (▶Fig. 5.3). In fact, monosynaptic projections from the primary motor cortex onto spinal motor neurons are most dense for muscles controlling hand and finger movement. This results in a greatly enhanced capacity to perform fine hand and finger movement (▶Fig. 5.4).

It is important to understand that the primary motor cortex does not contain a map of nerve cells controlling individual muscles; instead, patterns of movement are represented in the motor map of the primary motor cortex. 6 Neurons controlling hand and finger movement are located mainly within the hand area of the primary motor cortex; however, they also overlap extensively with neurons controlling more proximal muscles of the arm. This highly dynamic organization of the finger, hand, and arm motor maps in the primary motor cortex makes complex and well-coordinated movement possible in the upper extremity. This dynamic organization stands in striking contrast to the primary somatosensory cortex, where sensory information from the hand and fingers is sent to and processed in specific areas.
Although the primary motor cortex has the most direct access to the spinal interneurons and motor neurons, it does not solely control voluntary movement. Instead, it has been shown that the motor system is organized in a functional hierarchy including several different cerebral areas forming a motor network, where each level is concerned with a different decision. Cerebral control of movement is generally divided into three sequential stages—goal setting, planning, and coordination of muscle contracture—during which intentions are transformed into actions. 2 , 7 Most voluntary motor acts have a specific goal, so the first stage of the network is concerned with deciding the purpose or goal of the movement, which requires the prefrontal cortex (the anterior part of the frontal lobes lying in front of the motor and premotor areas) (▶Fig. 5.5).

At the next level, a motor plan is formed, involving interactions between the premotor areas (located anteriorly to the precentral gyrus or primary motor cortex) and somatosensory areas of the cerebral cortex. Normal behavior does not include a single movement of the hand and fingers; instead, it normally constitutes a sequence of movements that together form a behavior satisfying a specific aim. Movement design is mainly attributed to premotor areas. Neurons in premotor areas that control hand movements are strongly influenced by tactile stimuli on the glabrous surface of the palm and digits. 2 Furthermore, posterior parietal regions in the brain (Brodmann’s areas [BA] 5, 7, 39, and 40) play an important role in sensory guidance of movement. Neurons in area 5 receive central and peripheral nerve signals, allowing these neurons to integrate central motor commands with peripheral sensory information during reaching and grasping and thus making it possible to moderate and correct the motor plan and commands. 3 Furthermore, the parietal cortex is critical for the integration of visual data necessary for coordination of eye and hand movements. 8 Premotor areas also have a cognitive function. Specific neurons, known as mirror neurons, are activated during motor acts but also when a person simply watches another person perform a motor act. 9 These neurons are thought to be important for our understanding of the intentions and acts of other people as well as for learning new motor skills.
Somatosensory and visual cortex and cerebellum are also important in the formation of a motor plan, as they mediate information on the current state of the body to the premotor areas, such as the position, posture, and movement of the arms and hands and their interaction with the environment. 10 , 11
Information from the somatosensory and visual cortex to the motor system can play at least three functional roles: (1) feedback control of ongoing movements, such as to help adjust the output signal from the neurons controlling muscles acting on the hand to ensure that a person applies adequate force to an object to grasp it and to be able to manipulate it, but not to crush it or let it slip; (2) feed-forward control of intended movement, such as by positioning the hands appropriately while awaiting an approaching object, such as a ball; and (3) motor learning. The importance of somatosensory and visual information for movement of the hand becomes obvious after an injury to these systems. Such injuries not only cause impairment of object identification but also result in a severe impairment of the ability to move the hand and arm, especially when the movement includes interactions with objects.
After the complex process of motor planning comes the last stage in the control of voluntary movement, the coordination of muscle contractions needed to perform the planned movement. This is done by the primary motor cortex, brainstem, and spinal cord (▶Fig. 5.6).

The motor network is not completely hierarchical, sending information in a specific sequence between different brain areas where the primary motor cortex is the final common path. Although the primary motor cortex has the most direct access to the lower motor neurons, the premotor and parietal cortical areas can also influence spinal motor function through their own corticospinal projections. Furthermore, all areas in the motor network are heavily interconnected (▶Fig. 5.7).

As mentioned earlier, the motor output signal is monitored by neurons in the premotor areas and also by visual and tactile information. Furthermore, the motor network is monitored and modulated by the basal ganglia and the cerebellum. The cerebellum is well connected to the motor network (▶Fig. 5.7) and acts, for example, to correct errors in ongoing movements. Specialized neurons in the cerebellum assess the difference between the ongoing motor commands as issued by the motor network and the actual movement being performed. Furthermore, the cerebellum is important for learning new motor skills. The basal ganglia gate motor commands from the motor network, a function of great importance for the initiation of movement and the design of movement sequences. Diseases in the basal ganglia such as Parkinson’s disease, in which dopaminergic neurons in the basal ganglia are destroyed, cause difficulties both in initiating movement and in terminating movement once initiated.
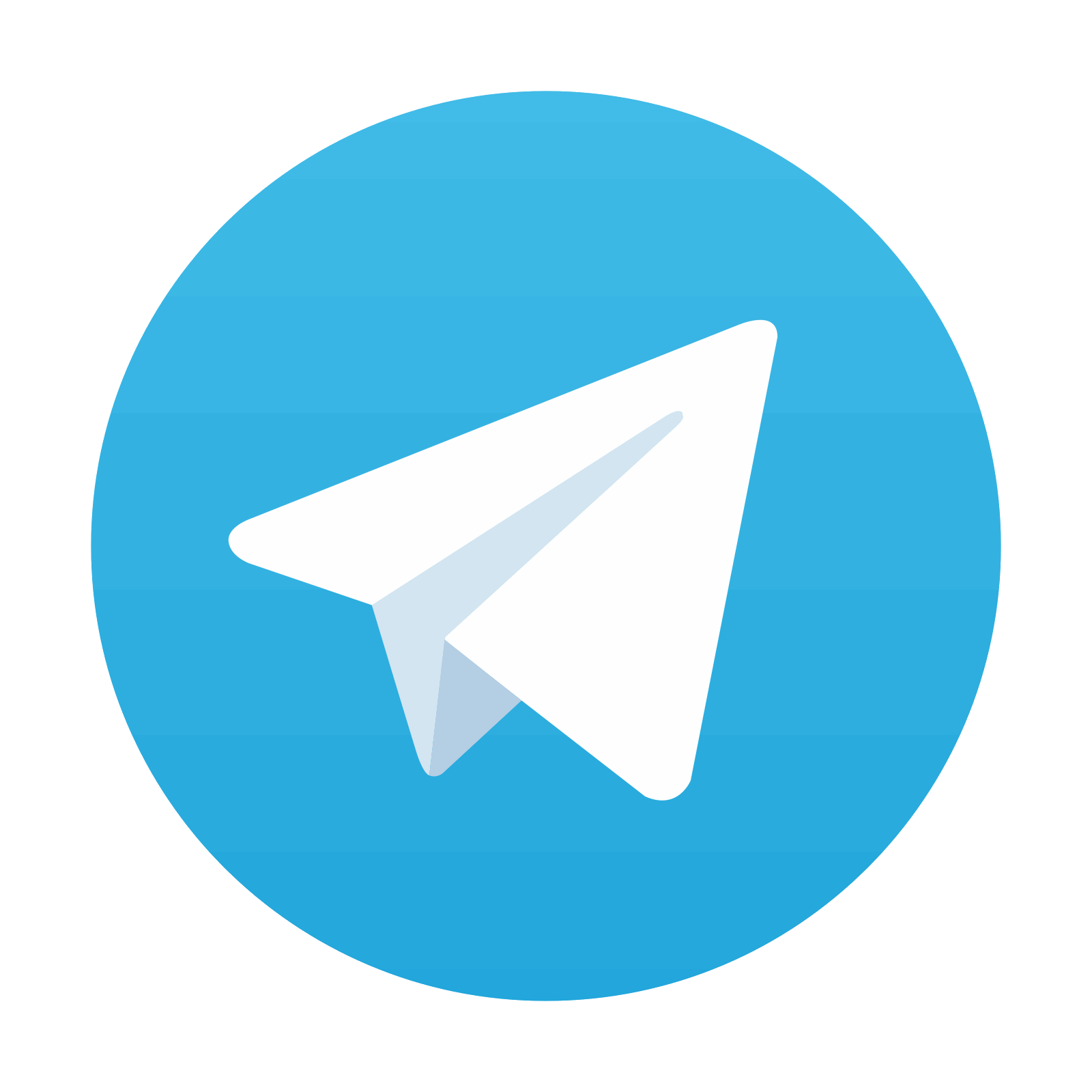
Stay updated, free articles. Join our Telegram channel

Full access? Get Clinical Tree
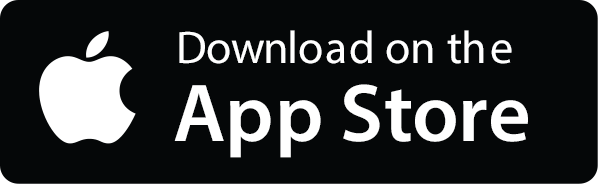
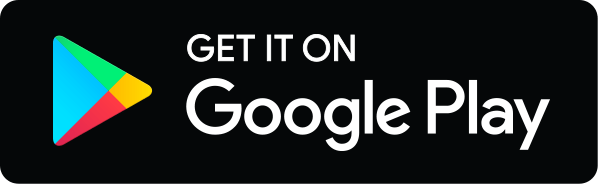