49 Stem Cells for Musculoskeletal Repair
Stem cells are precursors that differentiate to become terminally fated functional cells of tissues and organs. They have properties of self-renewal and have the ability to reconstitute the cells of damaged tissue. This chapter will focus on mesenchymal stem cells (MSCs) in bone regeneration. There is a desire to use stem cells in bone repair both to accelerate healing and to enhance the regeneration of hard-to-heal factures. An increase in bone formation could be accomplished by enhancing the direct formation of bone by osteoblasts (intramembranous bone formation) or by increasing the amount of bone formed on a cartilaginous template produced by chondrocytes (endochondral bone formation). The amount of precursor cartilage formed by chondrocytes could be enhanced or the rate of bone formation on the cartilaginous template accelerated. Both osteoblasts and chondrocytes differentiate from MSCs. MSCs are derived from a wide variety of sources, most commonly marrow and adipose; these cells can also be derived from induced pluripotent stem cells or embryonic stem cells. Interestingly, MSCs not only can contribute to the formation of bone and cartilage but also support vascularization, modulate inflammatory responses, secrete cytokines and growth factors, and produce an extracellular matrix conducive to the progression of healing. While MSCs show promise in preclinical models of bone repair and in isolated clinical cases, the promise of MSCs for bone regeneration has not been fully realized. Methods for cultivation, activation, and delivery must be better developed before the utilization of stem cells for tissue engineering of bone achieves robust clinically application. This chapter will consider MSC origin during bone regeneration, sources for and isolation of MSCs, evaluation of MSC proliferation and differentiation, and ultimately the utilization of MSCs to heal bone.
49.1 Introduction to Mesenchymal Stem Cells
Bone healing requires a robust mesenchymal cell response in order to build new bone through either endochondral (cartilage-mediated) or intramembranous (direct appositional) bone formation. Undifferentiated mesenchymal cells give rise to both osteoblasts and chondrocytes, and additionally promote angiogenesis. Thus, stem cell approaches that enhance the mesenchymal phase of bone repair have great potential for promoting bone regeneration.
Stem cells are undifferentiated progenitor cells that have the ability to become differentiated tissue forming cells and that are also capable of self-renewal. Another trait assigned to stem cells is the ability to reconstitute an ablated tissue. Thus hematopoietic stem cells, the classical stem cell, exist in a quiescent state, self-renew, and can reconstitute ablated marrow developing into all of the progenitor cells that give rise to fully differentiated inflammatory cells and blood cells.
Similar to hematopoetic stem cells, bone marrow is also the tissue from which MSCs were first isolated. In the 1960s, Friedenstein described the ability to isolate cells from marrow that had a high proliferation potential and that could form bone. 1 In early years, these cells were referred to as marrow stromal cells (also abbreviated as MSCs), because of their ability to also promote hematopoiesis. The bone-forming ability of these cells was largely ignored until the early 1990s, when the cells were first referred to as “mesenchymal stem cells,” which also, coincidentally, used the same abbreviation. A variety of additional nomenclature has been used to refer to the cells over time, but both the “marrow stromal cell” and “mesenchymal stem cell” designations have been most prominently used. Over the past ten years the acronym MSC as mesenchymal stem cell has been used most frequently because of its broader application to cells that are derived from tissues other than marrow.
These MSCs exist in a limited number and have the ability to differentiate to become osteoblasts, adipocytes, and chondrocytes, as well as hematopoietic supportive stroma (Fig. 49.1). In addition, these cells are believed to be capable of self-renewal. 2 With their ability to become both chondrocytes and osteoblasts, investigators began to realize their great potential for regenerating bone. Since these early years, thousands of papers have been published that have focused on a variety of topics related to MSCs, including their isolation, cultivation, delivery approaches, therapeutic potential, and safety. Indeed, a variety of commercial entities have been developed that seek to capitalize on MSCs for healing tissues, most relevant to this chapter, bone.

Whether an absence of stem cells or dysfunction causes poor, delayed, and nonunion bone healing remains a debatable topic. Theoretically, it is conceivable that stem cell dysfunction could be a contributor to poor healing, but this is not fully understood. Stem cell number declines with age, and this is also true with MSCs, but whether a lack of MSCs or poor activation of MSCs during healing is directly associated with age-associated decline in healing has not been definitively established in either animal models or humans. Regardless of whether there is an inherent deficiency in MSCs, it is prudent to be able to capitalize on their unique properties to promote bone regeneration.
It is also notable that MSCs not only differentiate to become bone-forming cells, they also possess unique properties as “bystander” cells (Fig. 49.2). They produce anti-inflammatory cytokines, are “immunoprivileged,” and do not produce a substantial immunological reaction. In addition, they produce additional growth factors that drive the healing cascade, in particular, promoting vascularization. Finally, MSCs also contribute to the production of a provisional matrix that is essential for supporting subsequent phases of the healing cascade. Thus, research has shown the ability of MSCs to modulate healing, repair, and remodeling in tissues such as heart and muscle, even when not directly contributing to tissue formation. Thus, while MSCs have been a focus for bone tissue engineering because of their ability to form bone and cartilage tissue, the cells could also play additional roles in healing bone.

While stem cells hold great promise for tissue engineering applications to increase bone regeneration, there are a variety of shortcomings that have limited their usefulness. In this chapter, these limitations and methods to address these limitations will be considered. Approaches for isolation, cultivation, differentiation, and delivery will be discussed. Where appropriate, the gaps in our knowledge base and potential strategies for addressing these gaps will be indicated.
49.2 The Physiological Origin of Mesenchymal Stem Cells during Bone Regeneration
Before considering MSCs as a therapeutic to repair bone, it is useful to consider the endogenous origin and function of MSCs during bone regeneration. Bone regeneration is complex, and mesenchymal cells are activated to populate a bone injury site secondary to a poorly described inflammatory response. Interfering with the inflammatory response can decrease mesenchymal influx, but the cytokines that are directly required for inducing mesenchymal influx are not well described. Furthermore, while some literature suggests that both local and distant progenitor cells contribute to repair, must recent data, using genetic models of cell tracking in mouse models, support the concept that local cells are the primary contributor to healing.
Bone marrow ablation delays fracture repair supporting an essential role for the marrow in bone regeneration; however, whether this is secondary to a loss of MSCs has not been fully established. The strongest evidence of the contribution of MSCs in the marrow to healing bone is developed from studies of mice with genetically engineered, lineage-specific markers that can identify the origin of cells and whether those marked cells develop into osteoblasts or chondrocytes. 3
Periosteum appears to be the major contributor of MSCs to bone repair (Fig. 49.3). Physical disruption of the periosteum by periosteal stripping delays healing. After injury, periosteal cells proliferate extensively during the mesenchymal phase of healing and then directly develop into both cartilage-forming chondrocytes and bone-forming osteoblasts in the fracture callus. The periosteum is also the primary source of chondrocytes in a gap defect model in a mouse, and this remains true when donor bone is grafted prior to injury so that the periosteum has an endosteal location.3

This work was recently extended by another research group working in a mouse model that temporally labels mesenchymal progenitors with a fluorescing protein at the time of fracture. They show definitively that periosteal MSCs have pronounced proliferation and that those cells can become both chondrocytes and osteoblasts. As well, MSCs from the marrow and endosteum also contribute to the healing response, but to a somewhat lesser degree. In this study, the fluorescing protein was expressed based on the expression profile of the alpha smooth muscle actin gene. While this protein is not specific for MSCs, it is a useful marker for cells that show mesenchymal multipotency. In uninjured bone, alpha smooth muscle actin–expressing cells are associated with the vasculature as well localized to the periosteum and are found sporadically in the marrow and endosteum. 4
Circulating stem cells may also have a minor contribution to endogenous repair; however, there is relatively little evidence of this in experimental models of bone healing. When bone morphogenetic protein (BMP)-containing collagen pellets are implanted in muscle or subcutaneously, this can induce the migration of cells from the marrow to the pellet that can then give rise to both cartilage-forming chondrocytes (which then undergo endochondral ossification) and bone-forming osteoblasts. More definitive proof that endogenous MSCs could migrate from the bone marrow to an injury site was provided with studies of systemic injection of a CXCR4 antagonist, which blocked healing. 5 CXCR4 is an essential receptor involved in cell homing and migration. Similarly, CXCR4 agonists can promote healing. However, presence in low numbers in regenerate tissue is not necessarily indicative of direct contribution to healing, thus whether circulating cells directly contribute to the formation of bone tissue under normal healing situations remains controversial. In most studies of injecting high numbers of MSCs in systemic circulation during the process of bone healing, relatively few cells home to the injury site and those that do likely do not differentiate into osteoblasts or chondrocytes and therefore do not contribute to the callus. However, despite not contributing directly to bone tissue formation, it remains possible that the injection of cells systemically could enhance healing through bystander effects, as previously described (production of proinflammatory cytokines, recruitment of other cell types, etc.).
These studies using lineage-marked mice are extremely valuable in discriminating the origin and fate of cells; however, it is worth considering that at this time the mouse remains the most amenable model for these studies. Studies must be done in larger animal models that more accurately mimic bone healing in humans to best understand the sources of cells into healing bone. This is necessary because if we identify which cell populations give rise to osteoblasts and chondrocytes, and which serve modulatory roles in normal healing bone, we can be guided in a more rational manner as we consider stem cells for therapies.
49.3 Sources of Mesenchymal Stem Cells
While the most relevant sources of MSCs for normal physiological bone repair are the bone and the marrow, MSCs can also be isolated from other tissues. MSCs are present in adipose, muscle, and can systemically circulate. In addition, MSCs can be derived from induced pluripotent cells and from embryonic stem cells. The ideal source of MSCs for bone repair regenerative therapies would be autologous, easily accessible, pain-free, and would result in large amounts of cells that behave in the expected and desired manner.
As previously mentioned, MSCs were first isolated from bone marrow, and bone marrow remains a common source of cells; however, bone marrow–derived MSCs are rare. They occur in every 10,000 to 100,000 nucleated cells, depending on species examined and methodologies utilized for isolation. Therefore, to get a purified population of MSCs requires that they be separated from hematopoietic cells in marrow samples. The first methodology to isolate MSCs involved plating cells on tissue culture plastic and allowing single cells to adhere and to form fibroblastoid colonies. In this case, contaminating hematopoetic cells are removed because they are nonadherent cells, and then the rapidly expanding progenitor populations are able to “out-compete” remaining potential contaminating cells. However, this approach can still result in significant cell heterogeneity (Fig. 49.4).

Bone marrow is readily accessible, and given that surgeons frequently use cancellous autograft for regeneration—which contains marrow in many cases—there is significant orthopedic surgeon comfort in the utilization of marrow as a source of MSCs. 6 Indeed, it is hypothesized that one important positive benefit to healing with autograft material is the potential contribution of transplanted cells; again, it is not well established that the cells from autograft material contribute in any significant manner to healed bone in humans.
An important question to consider regardless of the source of the MSCs is the degree of processing that is required for a viable therapeutic. Specifically, is it necessary to process and isolate the MSCs or is it sufficient to deliver MSCs in admixtures of cells in minimally processed autologous or allogeneic material? These approaches of using minimally processed tissue to deliver “stem cells” have been used clinically, but there is no definitive data in humans to support that these minimally processed samples contribute cells that are involved in bone regeneration.
Interestingly, MSCs also exist in solid bone, though there is some controversy about the source of an expanded cell population in these studies. The first studies used bone harvested using curettage or marrow reaming. Marrow is washed from isolated bone and then the bone is treated with collagenase. Bone chips are filtered away, and the cells that remain are plated and proliferate. Cells derived from bone have tridifferentiation potential and are similar to bone marrow–derived MSCs. 7 Despite having fewer total nucleated cells, there are apparently many more MSCs as evaluated using colony forming unit-fibroblast (CFU-F) analysis, suggesting the presence of more MSCs per total nucleated cell. Intriguingly, it is not entirely clear whether the cells that are present in these studies of whole bone are derived from within the bone or whether they exist on bone surfaces, for instance, representing the periosteum or the endosteum. Recent published work using fluorescent labeling shows that cells that can become MSCs may have transdifferentiated from osteocytes that were embedded in bony lacunae. 8
As previously discussed, periosteum is well recognized both experimentally and clinically as being a primary contributor of cells that form both cartilage and bone at the time of bone injury. It is a well-established standard of care that orthopedic surgeons not disturb or remove periosteum and that indeed when periosteum is removed the ability to heal is decreased. Experimentally, in rodent models, work has clearly shown the essential role of the periosteum in healing, as bone that is stripped of periosteum is incapable of repair. Furthermore, periosteum appears to contribute the bulk of cells to fracture healing in a mouse model.4 However, although periosteum is plentiful and represents the source of progenitor cells for naturally occurring fracture, it is generally considered a more difficult tissue to harvest compared to bone marrow.6 In addition, another complicating factor with respect to isolating periosteal cells is that the cellular layer of the periosteum (the cambrium layer) is strongly attached to the bone surface. Thus, developing methodologies that permit the harvest of periosteal donor tissue and cultivation of enough cells could be useful. Alternatively, developing tissue engineering strategies that seek to activate the endogenous periosteal progenitors may hold even greater promise.3
Although there is no evidence that adipose tissue is an endogenous source of MSCs for in vivo bone repair, harvesting MSCs from fat presents another possible source for therapeutic use. Adipose-derived MSCs are able to undergo osteogenic, chondrogenic, and adipogenic differentiation similar to MSCs from other tissue types. Adipose is a readily available source of progenitor cells for both autologous and allogeneic applications. Adipose-derived MSCs are present in the stromal vascular fraction of fat and are easily isolated by separating adipose-containing cells from other nucleated cells in fat using density centrifugation. Adipose is easily harvested through lipoaspiration and can then be used to isolate adipose-derived MSCs. 9
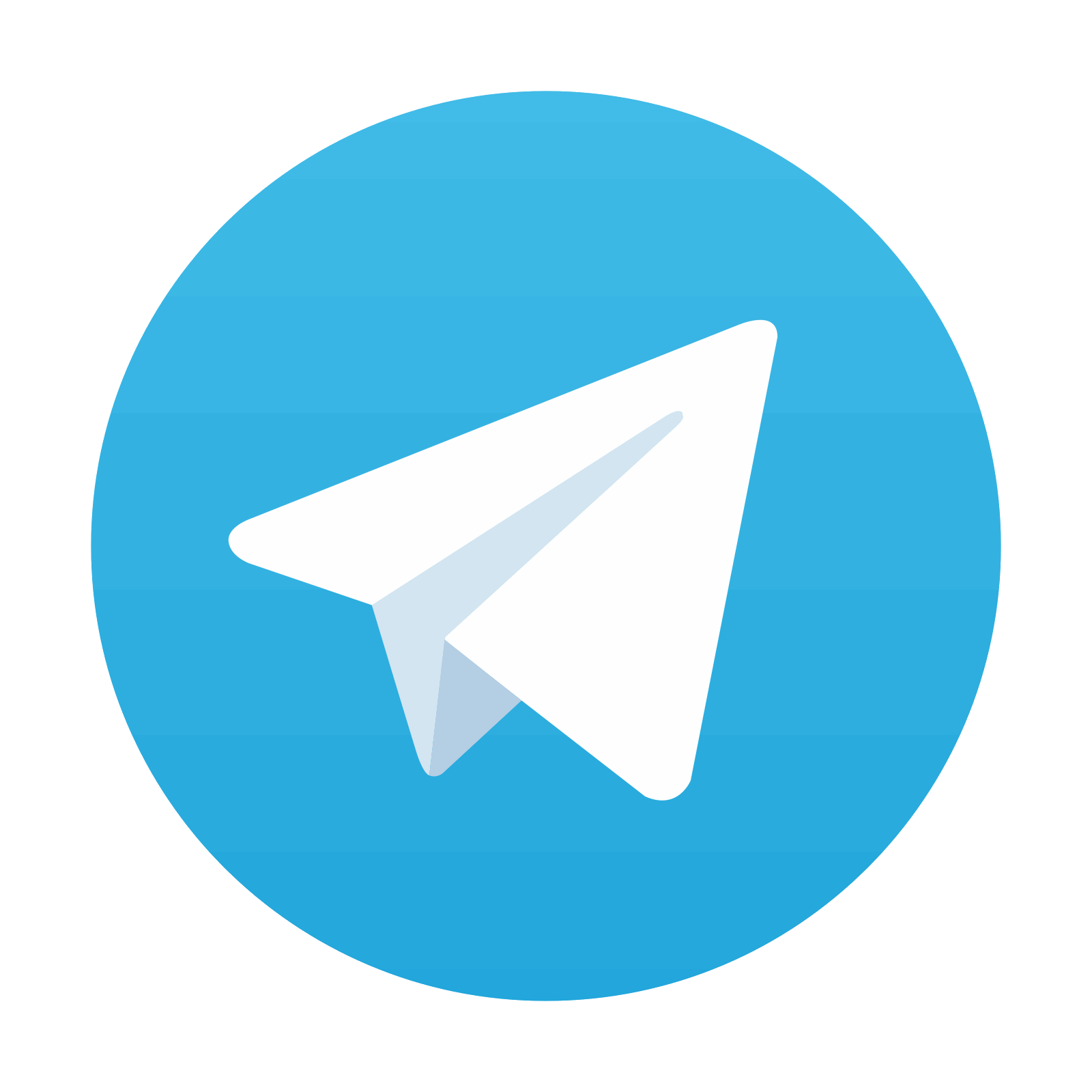
Stay updated, free articles. Join our Telegram channel

Full access? Get Clinical Tree
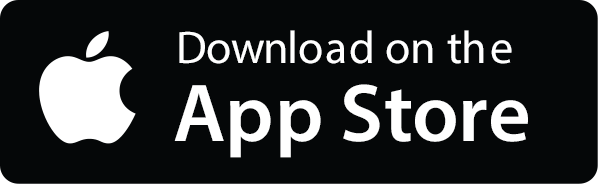
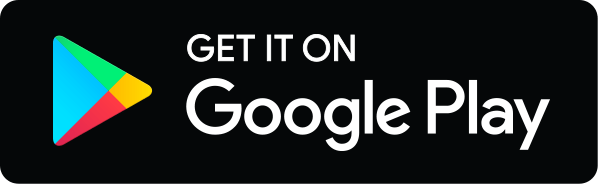