49 Cervical Spine Trauma
Introduction
Injuries of the cervical spine, particularly when associated with spinal cord injury (SCI), rank among the costliest to society. The treatment of spine fractures should be tailored to each patient based on fracture pattern, comorbidities, and other patient factors. This chapter aims to provide an overview of the evaluation and preferred treatment of the more common injuries of the upper and subaxial cervical spine (▶Video 49.1).
I. Preoperative
History and physical examination
Preliminary evaluation:
The highest priority in patients with cervical spine injury is to establish and maintain the airway, restore ventilation, and maintain blood pressure.
Hypotension in trauma patients is typically due to insufficient blood volume, and responds to fluid resuscitation and transfusion. Patients with SCI may also have neurogenic shock from loss of sympathetic function, manifested as hypotension with bradycardia, which may be treated with vasopressors and atropine.
Maintaining adequate spinal cord perfusion by keeping the mean arterial pressure at 80 mm Hg or higher may be a factor in minimizing the extent of SCI, and in promoting greater functional recovery.
Clinical evaluation:
After completing the primary survey, sensorimotor function of the extremities and the integrity of the spinal column are assessed in detail as part of the secondary survey.
The neurological examination involves documentation of the American Spinal Injury Association (ASIA) Impairment Scale (AIS), the level of neurological injury, and the ASIA motor score, based on manual muscle testing of five key muscle groups in each of the four extremities (▶ Fig. 49.1 ).
Fig. 49.1 American Spinal Injury Association International Standards for Neurological Classification of Spinal Cord Injury.
Sensation to pinprick and light touch in all dermatomes and vibration or position sense are evaluated. Deep tendon reflexes in both arms and legs should be performed and pathologic responses recorded.
Perineal function is assessed by evaluation of perianal pinprick sensation, voluntary anal sphincter contraction, and the bulbocavernosus reflex. Intact perianal function may be the only indication of an incomplete lesion and, in addition to having significant prognostic value, may influence the timing of surgical intervention.
The use of high-dose methylprednisolone in adult patients within 8 hours of SCI due to nonpenetrating trauma is controversial. Particularly in patients with comorbidities, it may do more harm than good.
Imaging and cervical spine clearance
Asymptomatic patients:
The clinical evaluation is often key to assessing for potential spine injury.
Important elements of the clinical examination include the presence of a neurological deficit, neck or back pain, or a palpable abnormality in spinal alignment.
In alert, nonelderly patients with low-energy mechanisms and no distracting injuries, the absence of neck tenderness or pain through a physiologic range of motion is typically considered sufficient to clear the cervical spine without imaging.
Symptomatic and obtunded patients:
Patients who do not match the above description require radiographic screening, which consists of any variety of institutionally standardized methods designed to exclude fracture and confirm anatomic cervical alignment (▶ Fig. 49.2 ).
Fig. 49.2 Canadian C-spine rule for cervical spine clearance.
The question of how cervical spine clearance should be undertaken in the comatose patient remains a matter of controversy.
Radiographic evaluation:
i. In trauma patients, the cross-table lateral plain radiograph has been widely supplanted by advanced imaging due to limited visualization of the cervicothoracic junction by plain films and improved speed of screening CT:
Radiographs are widely available and have a relatively high specificity (94%) and sensitivity (96%) in most circumstances.
These radiographs must be evaluated for soft-tissue swelling, fractures, and abnormalities in alignment.
ii. CT scan:
High sensitivity (95–99%) and specificity (93%) for detecting cervical spine fractures.
Has become the primary screening method in level-one trauma centers.
iii. MRI:
Useful in assessing vertebral column and spinal cord anatomy in patients with neurological deficits, and otherwise undetectable soft-tissue injuries that may influence treatment, such as nondisplaced diskoligamentous injury, disk herniation, and epidural hematoma.
Especially helpful in patients with progressive neurological deficits or deficits that do not correspond to CT findings.
Spinal cord signal change on MRI may also shed light on the nature of a neurological injury in the absence of osseous injury.
MRI also allows for prognostic assessment of SCI.
Although MRI has excellent sensitivity, its poor specificity makes it suboptimal in screening for cervical spine injuries.
iv. CT myelography may be useful if neuroimaging is desirable, but MRI is unavailable or contraindicated.
v. Patients with high-energy mechanisms should also receive routine imaging of the thoracic and lumbar spine, whether by plain radiographs, helical CT, or reformatted thoracic and abdominopelvic CT.
II. Specific Injury Types by Anatomic Region: Classification, Treatment, Indications for Surgery, Outcomes, and Complications
Upper cervical spine (Occiput to C2)
The occipitocervical (O-C) junction is a functional unit that consists of osseoligamentous and neurovascular structures that extend from the skull base to C2. It includes the O-C and atlantoaxial articulations. Stability of the O-C junction is established primarily by its unusual ligamentous anatomy rather than by intrinsic bony stability. Patient outcome often depends more on associated intracranial injury than on the injury to the spine.
Occipital condyle fractures:
Classification—occipital condyle fractures may be unstable when they represent bony avulsion of major O-C stabilizers. Anderson described a classification system (▶ Fig. 49.3 ) consisting of three categories:
Fig. 49.3 Anderson and Montesano classification of occipital condyle fractures.
Type I: stable, comminuted axial loading injuries.
Type II: potentially unstable injuries caused by a shear mechanism that results in an oblique fracture extending from the condyle into the skull base.
Type III: alar ligament avulsion fractures that may be part of an unstable O-C dissociation.
Indications for surgery—operative intervention, in the form of O-C fusion, is generally reserved for type III injuries with O-C instability. Nonoperative management with a cervical collar is recommended for the majority of (stable) type I and II injuries.
Outcomes and complications—symptomatic post-traumatic arthritis resulting in neck pain, occipital headaches, restricted O-C motion, and torticollis.
Palsy of closely associated cranial nerves (IX, X, XI, XII) has been described.
If part of an O-C dissociation, prognosis is worse (see later).
OC dissociation:
Classification—the Harborview classification system, based on the extent of instability, may require traction testing of minimally displaced injuries (≤2 mm) to appropriately guide treatment and prognosis.
Indications for surgery: If the basion dens interval (BDI) or basion axis interval (BAI) is greater than 12 mm, O-C dissociation is likely, and should be investigated with MRI.
Displacement of more than 2 mm at the atlantooccipital joint on static imaging or with provocative traction testing (▶ Fig. 49.4 ), or the presence of neurological injury, is an indication for O-C stabilization.
Fig. 49.4 Positive provocative traction test, manifested as greater than 2 mm of atlantooccipital joint distraction, can be used to confirm the diagnosis of occipitocervical dissociation in a patient with instability that was out of proportion to the amount of initial occipitocervical displacement.
Outcomes and complications—most O-C dissociations are fatal.
The outcome of survivors is dependent on the following:
i. Type and severity of associated injuries, particularly closed head injuries.
ii. Severity of neurological injury at the O-C junction.
iii. Timing of diagnosis and stabilization of O-C dissociation is important. Delayed diagnosis is associated with secondary neurological deterioration and possibly death in up to 75% of patients.
Vertebral artery injury should be considered in any distractive upper cervical injury.
Fractures of the atlas:
Classification—classified as either stable or unstable based on the integrity of the transverse alar ligament (TAL).
TAL insufficiency can be diagnosed directly by identifying bony avulsion on CT scan or ligament rupture on MRI, or indirectly by identifying widening of the C1 lateral masses with ≥7 mm lateral overhang relative to the lateral masses of C2 on either open mouth odontoid or coronal CT images (▶ Fig. 49.5 ).
Fig. 49.5 Upright open-mouth anter-oposterior (odontoid view) view demonstrates a cumulative 11-mm overhang bilaterally of the C1 lateral masses beyond the C2 lateral mass, suggesting rupture of the transverse atlantal ligament. Although the C1–C2 overhang can also be measured on coronal CT images, upright open mouth views provide a better measure of stability due to loading of the spine in the upright position.
C1 fractures are also classified as (i) axial loading type C1 ring fractures, (ii) lateral mass fractures, and (iii) posterior arch fractures.
Indications for surgery—most C1 fractures are treated nonoperatively.
If upright radiographs with external immobilization show unacceptable lateral mass displacement (≥7 mm) or an anterior atlantodens interval (ADI) of greater than 3 mm, patients are typically treated with posterior C1–C2 or occiput C2 fixation.
Surgical stabilization typically consists of posterior C1–C2 instrumented fusion.
Outcomes and complications:
i. Severe complications are rare.
ii. Eighty percent incidence of residual neck pain, possibly due to post-traumatic arthritis.
iii. Seventeen percent nonunion rate.
iv. Severe malunion of unstable atlas fractures may result in painful torticollis, requiring realignment and O-C fusion.
Atlantoaxial instability:
Classification—three atlantoaxial instability patterns can occur and may coexist.
i. Type A injuries are rotationally displaced in the transverse plane. These deformities are usually nontraumatic in nature.
ii. Type B injuries are translationally unstable in the sagittal plane due to TAL insufficiency. Distinguishing a ligamentous TAL tear (type I) from a bony avulsion fracture (type II) may impact treatment (▶ Fig. 49.6 ).
Fig. 49.6 (a) Lateral radiograph demonstrates anterior translation of C1 on C2 with increased atlanto dens interval associated with traumatic transverse atlantal ligament (TAL) disruption. (b) Axial CT shows avulsion fracture of the TAL insertion site (arrow), which makes this injury potentially amenable to nonoperative treatment. (c) Due to the high amount of displacement and instability and the patient’s multiple injuries, a C1–C2 posterior instrumented arthrodesis was performed as demonstrated on lateral x-ray done 6 months postoperatively.
iii. Type C injuries are distractive injuries that represent a variant of O-C dissociation.
Diagnosis:
i. Type B: suspect if plain radiographs or CT shows ADI greater than 3 mm.
ii. Type C: suspect if distraction is noted on imaging studies or if Harris’ lines are greater than 12 mm.
Indications for surgery:
i. Type B: translational instability—posterior atlantoaxial arthrodesis.
ii. Type C: distraction injuries—posterior atlantoaxial versus O-C stabilization.
Outcomes and complications:
i. Acute TAL insufficiency is usually fatal.
ii. In survivors, profound neurological deficits or head injury may be present.
iii. Syncope and vertigo may result from injury to vertebrobasilar arterial system.
iv. Atlantoaxial distraction has a similar prognosis to O-C dissociation.
Odontoid fractures:
Classification—three-part classification of Anderson and D’Alonzo (▶ Fig. 49.7 ).
Fig. 49.7 Anderson and D’Alonzo classification of odontoid fractures. (a) Type I. (b) Type II. (c) Type III. (d) Type IIa (segmentally comminuted).
i. Type I injuries are bony avulsions of the alar ligament, which may result in O-C dissociation.
ii. Type II injuries at the odontoid waist, which have the highest propensity for pseudar-throsis, due to vascular watershed phenomenon and small cancellous bone surface area. The IIa subtype consists of a highly unstable, segmentally comminuted fracture.
iii. Type III fractures extend into the cancellous vertebral body and have wider, well-vascularized cancellous fracture surfaces.
Indications for surgery:
i. Type I:
The treatment of type I odontoid fractures relates to their impact on O-C stability. The indications for surgical management of these injuries are therefore the same as those discussed for the treatment of O-C instability.
ii. Type II:
Surgical indications remain controversial, but the weight of recent evidence suggests decreased mortality and complications with surgery. We advocate surgical stabilization for displaced fractures in patients with functional needs, distractive patterns of displacement, or fractures with associated SCI (▶ Fig. 49.8 ).
Fig. 49.8 (a) Sagittal CT image demonstrates displaced type II geriatric odontoid fracture, which was treated with posterior reduction and C1–C2 instrumented arthrodesis. (b) Postoperative upright lateral X-ray shows restoration of alignment and stability.
Relative indications include multiply injured patients, associated closed head injury, initial displacement of greater than 4 mm, angulation greater than 10 degrees, delayed presentation (>2 weeks), multiple risk factors for nonunion, the inability to externally immobilize, associated intracranial or thoracoabdominal injury, other medical comorbidities, and the presence of associated upper cervical fractures.
Displaced, noncomminuted fractures with favorable bone quality and fracture obliquity and appropriate body habitus are ideal for anterior odontoid screw fixation.
In patients with extensive fracture comminution, compromised bone quality, or with technical constraints to anterior odontoid screw trajectory, we favor posterior atlantoaxial fusion using either transarticular screw fixation or segmental C1–C2 fixation.
iii. Type III:
Operative stabilization is not commonly required, but is warranted in patients with significant/progressive deformity, SCI, or distractive instability patterns.
Delayed unions or pseudoarthroses occur in up to half of nonoperatively treated patients, and are also amenable to posterior C1–C2 or C1–C3 fixation.
Posterior C1–C2 versus C3 posterior spinal instrumentation and fusion (PSIF) is the surgical treatment method of choice, since anterior odontoid screw fixation has a high failure rate with type III odontoid fractures.
Outcomes and complications:
i. Associated with significant morbidity and mortality.
ii. Neurological injury occurs in up to 25% of type II odontoid fractures, and ranges in severity from isolated cranial nerve injury to complete quadriplegia.
iii. One-year mortality rates for elderly patients with type II odontoid fracture have been reported to be as high as 40%.
iv. Fracture nonunion and missed injuries are the most common complications.
v. Risk factors for nonunion include initial nonoperative treatment, displacement of greater than 4 mm, fracture angulation greater than 10 degrees, male gender, older age, and delay in treatment.
vi. Perioperative complication rate of approximately 30% and a nonunion rate of approximately 10% have been described with odontoid screw fixation.
vii. C1–C2 fusions have reported nonunion rates of 4% or less using rigid fixation.
viii. Though considered more benign injuries, nonoperative treatment of type III odontoid fractures is associated with pseudoarthrosis rates of 9 to 13%.
Traumatic spondylolisthesis of the axis (hangman’s fractures):
Classification—three primary injury types and two “atypical” subtypes (▶ Fig. 49.9 ).
Fig. 49.9 Classification of hangman fractures. (a) Type I. (b) Type IA (atypical hangman fracture). (c) Type II. (d) Type IIA. (e) Type III.
i. Type I: minimally displaced, stable fracture of the pars interarticularis.
ii. Type IA: atypical unstable, obliquely displaced fracture typically extending through one pars and more anteriorly into the body on the contralateral side.
iii. Type II: displaced injuries of pars interarticularis with greater than 3 mm C2–C3 translation.
iv. Type IIA: unstable flexion–distraction injury with associated C2–C3 disk and interspinous ligament disruption. Kyphosis is the prevailing deformity rather than translation (▶ Fig. 49.10 ).
Fig. 49.10 Sagittal CT images at midline (a) and through the pars interarticularis (b) show kyphosis disproportionate to the degree of anterior translation and a horizontal tension type failure of the pars interarticularis (arrow), both of which are hallmarks of the type IIA hangman fracture. This injury typically results from a flexion–distraction mechanism, causing posterior-to-anterior disruption of the C2–C3 disk space, which is illustrated on sagittal T2-weighted MRI (c). (d) Lateral X-ray 3 months after anterior diskectomy and fusion shows acceptable alignment.
v. Type III: Unstable injuries in which C2–C3 facet dislocation accompanies pars interarticularis fracture.
Indications for surgery:
i. Operative stabilization is rarely indicated for traumatic spondylolisthesis of the axis, the most common of which are type II injuries.
ii. Most type I and II injuries are treated with 12 weeks of external immobilization using a rigid collar or halo vest.
iii. Type IA injuries behave unpredictably and often fail nonoperative treatment.
iv. Type IIA injuries typically require surgical stabilization:
Traction is contraindicated, as it accentuates their kyphotic deformity.
A C2–C3 anterior diskectomy and fusion (ACDF) with plating allows for fusion across the least number of levels (▶ Fig. 49.10 ).
Posterior stabilization is more stable, but unless adequate purchase is achieved across the fractured C2 pars interarticularis, loss of atlantoaxial motion results from the need to extend fixation to C1.
Type III injuries are generally irreducible by traction and require open posterior reduction and stabilization. Stabilization options include the following:
i. Posterior C1–C3 fusion.
ii. Posterior C2–C3 fusion using lag screws across the fracture at C2.
iii. Anterior C2–C3 ACDF if reduction has occurred spontaneously or in the unusual event that reduction occurs by closed methods.
Outcomes and complications:
i. Associated injuries such as upper cervical (15%), subaxial (23%), or head injuries usually have a greater influence on prognosis than the C2 fracture itself.
ii. Neurological injury has been identified in only up to 10% of patients, but occurs in 60% of type III and 33% of type IA fractures (▶ Fig. 49.11 ).
Fig. 49.11 Atypical (type IA) hangman fracture, as seen on (a) lateral X-ray and (b) axial CT image. Along with type III injuries, type IA injuries have a higher propensity for causing spinal cord injury than other hangman fractures because the fracture pattern may form a spike that impinges on the spinal cord with fracture displacement (arrow).
iii. Type IA injuries also have a greater potential for vertebral artery injury because of common foramen transversarium involvement.
iv. Traumatic spondylolistheses of the axis have a 5% pseudoarthrosis rate.
v. Type IA, IIA and III fractures are more challenging to treat due to either atypical fracture orientation or associated ligamentous injury.
Subaxial cervical spine (below C2)
Classification of subaxial cervical spine injuries:
There is no universally accepted classification system for fractures and dislocations of the subaxial cervical spine.
A recently developed (2016) AO subaxial C-spine injury classification combines morphological features of the injury, type of facet injury, severity of neurological injury, and patient specific modifiers (▶ Fig. 49.12 ).
Fig. 49.12 (a) AO morphological classification of subaxial cervical spine fractures. (b) Algorithm for determining AO subaxial cervical spine injury type. (Acknowledgment of copyright – AOSpine International© AOSpine International, Switzerland Acknowledgment of the AOSpine Knowledge Forum work “AOSpine is a clinical division of the AO Foundation—an independent medically guided nonprofit organization. The AOSpine Knowledge Forums are pathology-focused working groups acting on behalf of AOSpine in their domain of scientific expertise. Each forum consists of a steering committee of up to 10 international spine experts who meet on a regular basis to discuss research, assess the best evidence for current practices, and formulate clinical trials to advance spine care worldwide. Study support is provided directly through AOSpine’s Research department and AO’s Clinical Investigation and Documentation unit. This figure can be found at www.aospine.org/classification.)
Brief summary of AO classification:
i. Morphology.
ii. Type A: compression injuries with intact tension band (AO through A4).
iii. Type B: tension band injuries without spinal discontinuity or translation (B1 vs. B2).
iv. Type C: displacement or translation of one vertebral body relative to another in any direction; anterior, posterior, lateral translation, or vertical distraction (no subtypes):
Injuries are classified by level and either C, B, or A in this order.
Type A (vertebral body) injuries associated with type B or C injuries are then listed, as they may affect treatment or prognosis.
v. Facet injury descriptors (F1 through F4):
Describe the specific features of the facet injury.
F1: nondisplaced facet fracture.
F2: facet fracture with potential for instability.
F3: floating lateral mass.
F4: pathologic subluxation or perched/dislocated facet.
vi. Neurological examination (Nx and N0 through N4):
Describes the severity of neurological injury.
Complimentary to the AIS and ASIA motor scores.
vii. Case-specific modifiers—patient or injury features that may affect treatment or prognosis:
M1: evidence of posterior capsuloligamentous complex injury without complete disruption.
M2: critical disk herniation.
M3: ankylosing spine condition.
M4: vertebral artery injury.
Mechanistic classification systems based on that proposed by Allen et al in 1982 can be valuable in comprehending instability patterns. It is useful to consider the injury mechanism as occurring along a continuum involving four “cardinal” force vectors to which the spine may be subjected: distraction, compression, extension, and flexion (▶ Fig. 49.13 ).
Fig. 49.13 The Allen–Ferguson cervical spine fracture classification is based on the presumed forces applied to the neck at the time of injury, and results in a continuum of fracture patterns based on varying and often combined influence of four primary force vectors: distraction, extension, compression, and flexion. A lateral compression injury pattern was also included, but is not illustrated.
Injury categories:
AO type A injuries: vertical compression injuries—burst fractures:
i. General considerations:
Vertical compression injuries result from an axial load applied to the top of the head with the cervical spine in a nonflexed position. The fracture pattern, commonly known as a cervical burst fracture if the posterior cortex is involved, is characterized by relatively symmetric loss of anterior and posterior vertebral body height.
Uncommon injury with C7 most commonly affected.
Distinguished from flexion–compression (flexion teardrop) injuries by relative absence of kyphosis and translational malalignment.
Injury to the posterior ligament complex is uncommon.
ii. Treatment:
Treatment is largely determined by the presence or absence of SCI, the degree of canal compromise, spinal alignment, and the integrity of posterior soft tissues (▶ Fig. 49.14 ).
Fig. 49.14 (a) Sagittal CT image demonstrates a C7 burst fracture. These typically occur in the lower cervical spine. Because of the extent of comminution, and the presence of neurological deficits, the patient underwent an anteroposterior reconstruction consisting of C7 corpectomy, C6–C7 laminectomy and C5–T1 posterior arthrodesis, as demonstrated on (b) postoperative sagittal CT image and (c) lateral X-ray 6 months postoperatively.
If overall architecture of the vertebral body is reasonably well maintained, with minimal retropulsion and no neurological injury, treatment consists of 12 weeks of immobilization with a rigid cervical orthosis, SOMI-type brace or halo vest.
With more severe loss of vertebral body height, wider centrifugal fracture displacement resulting in greater bony retropulsion into the spinal canal, kyphotic malalignment, and neurological deficit occurs more frequently. Anterior decompression and stabilization with corpectomy, interbody reconstruction, and plating are warranted. Addition of PSIF is appropriate if there is concern about stability of stand-alone anterior reconstruction, particularly in the presence of posterior ligamentous injury (type B injury).
AO type B injuries—extension or flexion “bending” injuries without translational displacement.
i. Caused primarily by a flexion or extension moment, with potential application of compressive or distractive forces that give characteristic morphological features (▶ Fig. 49.15 , ▶ Fig. 49.16 ).
Fig. 49.15 Sagittal CT image demonstrates a C7–T1 flexion–distraction injury, consisting of tension failure of the C7 spinous process (black arrow) and compression fracture of the superior end plate of T1 (white arrow). Fig. 49.16 C6–C7 extension fracture in a patient with diffuse idiopathic spinal hyperostosis (DISH). Injuries that have angulation without translation are designated as type B injuries.
ii. More severe stages of these injuries may involve sagittal plane translation, which typically affects treatment and prognosis, thus categorizing them as type C injuries (▶ Fig. 49.17 ).
Fig. 49.17 Sagittal CT image of C6–C7 extension fracture with translational malalignment (AO type C) that occurred below a previous C6–C7 fusion in a patient with ankylosing spondylitis.
iii. Facet injuries are quantified separately (▶ Fig. 49.12 ) based on the severity of the facet injury.
AO type C injuries—unilateral facet dislocations and fracture-dislocations with translational displacement.
i. The defining characteristic is a rotational deformity in the axial plane.
ii. The rotational deformity in unilateral facet injuries is manifested on sagittal plane images as 25% or less anterolisthesis of the affected vertebral body (▶ Fig. 49.18 ).
Fig. 49.18 Sagittal CT images along the midline (a) and the left facet joints (b) show a C4–C5 left unilateral facet dislocation with perched facets. Anterior vertebral body translation of less than 25% at the injury level indicates the presence of unilateral facet dislocation.
iii. The mechanism of injury involves a flexion injury with resulting distraction of the posterior elements, coupled with a rotational force.
AO type C injuries—bilateral facet dislocations and fracture-dislocations with translational displacement.
i. Typically results in at least 50% anterior vertebral body translation. Facet fractures occur frequently with unilateral and bilateral facet dislocations.
ii. Reversal of the position of the superior relative to the inferior facets provides the typical appearance of facet dislocation on axial and sagittal CT images.
iii. MRI illustrates the soft-tissue injury and degree of spinal cord compression, and is likely to show disruption of the interspinous ligament and facet capsules, with at least partial intervertebral disk disruption in over 60% of cases.
iv. Disruption of the annulus may result in disk extrusion into the spinal canal, which may have important treatment implications, as discussed in detail later.
v. MRI also plays a prognostic role in predicting the potential for functional recovery from SCI based on the appearance of the injured spinal cord.
The role of prereduction MRI:
Whether an MRI scan to identify disk herniation is required prior to closed reduction of facet dislocations remains a matter of considerable controversy.
Spinal realignment in the presence of posteriorly extruded disk material has been postulated to result in SCI from cord compression, if reduction causes displacement of the disk material into the canal.
However, regardless of the presence of intervertebral disk extrusion, if a patient can participate for neurological examination, closed reduction with clinical monitoring of neurological status has been shown to be safe, with the understanding that the procedure be aborted at the first sign of neurological abnormality.
Conversely, in a patient who is obtunded, anesthetized, or in whom a reliable neurological examination cannot be obtained during the course of reduction, circumstances may dictate that an MRI scan be obtained prior to any attempt at reduction. If the MRI were to demonstrate a concerning disk extrusion, anterior diskectomy could be undertaken prior to spinal realignment and fixation.
Available evidence does not strongly support any treatment standard for facet dislocations.
The risk of neurological worsening during spinal realignment must be weighed against the detrimental effect of delay in spinal realignment on neurological outcome.
Treatment:
Closed reduction technique:
i. Patients who present with a unilateral or bilateral facet dislocation, particularly when associated with neurological injury, should undergo an attempt at closed reduction at the earliest possibility, according to the above guidelines.
ii. The reduction is ideally performed in a fluoroscopy suite, under mild sedation.
iii. Traction pulleys are positioned to allow for flexion of the neck during the initial phase of reduction to facilitate disengagement of the dislocated facet.
iv. After application of the initial 5 to 10 lb of traction, the O-C junction should be scrutinized for undetected unstable ligamentous injuries.
v. With the addition of subsequent weight, the injured level and all other intervertebral levels should be evaluated for unacceptable distraction.
vi. A thorough sensorimotor evaluation must be performed after each increase in weight, and the patient should be questioned regarding the presence of new or worsening neurological symptoms. Any such complaints should cause the reduction procedure to be aborted in lieu of an open reduction (see later).
vii. Weight is added in 5- to 10-lb increments until the dislocated facet appears to have “cleared” its more caudal counterpart. Increasing the extension vector of the traction by lowering the height of the traction pulley or placing an interscapular bump beneath the patient may then facilitate the final phase of reduction.
viii. Once reduction has been achieved, traction weight is incrementally reduced under fluoroscopic evaluation to between 15 and 25 lb, depending on which level is involved. Higher weights may be required if recurrence of subluxation occurs.
ix. An MRI of the cervical spine is obtained at the earliest possibility, regardless of whether one was obtained prior to reduction, to evaluate the spinal cord and the presence of any compressive lesions.
x. If an objective neurological deficit occurs, the inciting event should be reversed. Although controversial, methylprednisolone might be administered according to institutional protocol. Imaging studies should be obtained to assess for potential causes.
Definitive surgical treatment:
i. Cervical facet dislocations and fracture-dislocations require operative stabilization.
ii. In the absence of anterior compressive lesions on postreduction MRI, posterior stabilization is appropriate, and may be superior biomechanically to anterior fixation.
iii. Even in the absence of disk herniation, ACDF is acceptable and sometimes preferable, particularly in patients whose care could be compromised by prone positioning, or in whom ACDF might spare a fusion level.
iv. Although biomechanically inferior to PSIF, treatment with ACDF has been largely equivalent for (fracture-)dislocations, with fusion rates exceeding 90%.
v. In the presence of significant disk herniation on postreduction MRI, particularly with associated neurological deficits, ACDF is the most appropriate treatment.
vi. Although not always necessary after ACDF, adding PSIF may be appropriate for highly unstable injuries or in patients otherwise prone to failure of fixation due to osteoporosis or other medical comorbidities.
vii. The inability to successfully reduce a facet dislocation with closed techniques may complicate the choice of anterior versus posterior approach.
viii. Traditionally, in the absence of disk herniation, unreduced flexion–distraction injuries have been realigned and stabilized through a posterior approach.
ix. Conventional treatment for unreduced injuries with MRI evidence of disk herniation has been anterior diskectomy followed by prone positioning and posterior reduction and stabilization, possibly followed by anterior interbody fusion.
x. If an anterior approach is warranted for unreduced dislocations, the authors prefer to complete the reduction and stabilization entirely from the anterior approach, when possible. Once the diskectomy has been performed, reduction can safely be performed by various means, such as Gardner–Wells tong traction, or direct manipulation of the vertebral bodies with a Cobb elevator, lamina spreader, or Caspar pins.
xi. Surgical procedures requiring reduction are performed with spinal cord monitoring.
Flexion–Compression (flexion teardrop) injuries
General considerations:
Axial loading injuries with an associated flexion force vector, typically caused by diving injuries, football spearing injuries, and motor vehicle collisions.
Consistent injury pattern with varying degrees of severity:
i. In more severe stages, the primary fracture line separates the anteroinferior corner of the vertebral body (the so-called teardrop), which remains aligned with the caudal intervertebral disk and vertebra, from the remaining, posteriorly displaced vertebral body.
ii. The severity of retrolisthesis and canal compromise, the presence of which qualifies the injury as AO type C, correlates with risk of SCI. Injuries with greater than 3 mm of retrolisthesis have greater than 90% likelihood of SCI, over half of which are complete.
iii. The axial compression results in an associated sagittal split through the vertebral body in up to two-thirds of cases, as well as bilaminar fracture. The combination of sagittal vertebral body split with neural arch fracture is usually associated with a severe SCI.
The flexion component threatens the integrity of the posterior ligamentous structures.
Classification:
The severity of flexion–compression injuries is contingent on the degree of vertebral comminution, kyphosis, and retrolisthesis.
Radiographs and CT scan should be scrutinized for evidence of interspinous or facet widening, and MRI should be obtained to assess the status of the interspinous ligament, facet capsules, and ligamentum flavum.
Lower-grade injuries without gross angular or translational malalignment or compromise of the posterior ligamentous complex (PLC) are best classified as AO type A injuries.
Moderate-grade injuries with disruption of the PLC and kyphosis, without retrolisthesis, are best classified as AO type B injuries ( Fig. 49.19 ).
Fig. 49.19 Sagittal CT image of C5 flexion teardrop fracture in a neurologically normal patient. Because there is minimal retrolisthesis and kyphosis, and no evidence of severe posterior element injury, an anterior-only reconstruction was performed.
The highest grade injuries, in which PLC injury and kyphosis are associated with retrolisthesis, are best classified as AO type C injuries ( Fig. 49.20 ).
Fig. 49.20 Sagittal CT image of a C5 flexion teardrop fracture with severe retrolisthesis and posterior ligamentous complex disruption with kyphosis in patient with quadriplegia.
Treatment:
Patients with SCI or injuries qualifying as AO type B or C require surgery.
Low-grade (type A) injuries—in the absence of neurological deficits, nonoperative treatment with 12 weeks of rigid external immobilization:
i. The potential for progressive kyphosis and instability after nonoperative treatment is high, and requires careful radiographic follow-up.
ii. Flexion–extension radiographs should be obtained after 12 weeks, when external immobilization is discontinued.
iii. Anterior corpectomy and instrumentation are required in the presence of SCI.
Moderate- to high-grade (types B and C) injuries: operative intervention is required in the presence of SCI or PLC injury:
i. Corpectomy with anterior instrumentation is the surgical treatment of choice, for flexion teardrop injuries, and is superior to rigid immobilization in maintaining sagittal alignment and minimizing treatment failures.
ii. Supplemental posterior fixation may be required in more severe injuries, in the presence of metabolic bone disease, if stability remains a concern due to extensive posterior injury, or if a posterior approach is required for the purpose of spinal cord decompression.
Outcomes—high-fusion and functional neurological recovery rates in patients with incomplete SCI have been reported with anterior treatment of flexion–compression injuries, with few approach-related complications.
Extension injuries
General consideration:
Extension injuries usually result from a blow to the face or forehead.
The degree to which extension is combined with a distraction or compression force vectors influences both the injury morphology and subsequent treatment.
In extension–distraction injuries, the sequence of injury to the spinal column progresses from the anterior through the middle column to the posterior column. Simultaneous compressive forces across the posterior elements may result in fractures involving the neural arch, lateral masses, or pedicles.
Extension injuries typically result from high-energy mechanisms in younger patients with nonspondylotic cervical spines, or from seemingly trivial injuries in older patients with spondylotic or ankylosed spines. Treatment for these two groups can differ significantly.
Injuries are broadly divided into those that result in obvious compromise of the osseoligamentous elements of the cervical spine, and those with SCI but no obvious radiographic evidence of musculoskeletal injury or spinal instability.
Injuries with radiographic signs of a vertebral column lesion are classified and treated according to the severity of hyperextension deformity and sagittal plane translation, which also correlate with the likelihood of SCI.
Extension injuries are classified as AO type B3 in the absence of translation (usually retrolisthesis) and as AO type C injuries if associated with translation/retrolisthesis. SCI occurs with higher frequency in patients with retrolisthesis due to compression between the posteroinferior end plate of the posteriorly displaced vertebral body and the anterosuperior lamina of the more caudal vertebra.
Cervical SCI not accompanied by radiographic signs of osseoligamentous injury are typical of central cord syndrome and SCWIORA (SCI without radiographic abnormality).
Examples of extension injuries:
Extension teardrop fractures:
i. Malrotated avulsion fracture of anteroinferior end plate, most often of C2.
ii. Should be differentiated from the more severe flexion teardrop fracture.
iii. External immobilization for 6 to 12 weeks is successful in most patients.
Disruptions of the anterior longitudinal ligament (ALL) and intervertebral disk:
i. Purely diskoligamentous injuries involving the ALL, intervertebral disk, and possibly the posterior ligaments.
ii. Lack of a visible fracture may present a diagnostic dilemma, particularly in injuries that reduce spontaneously on supine imaging.
iii. Retrolisthesis indicates a more unstable injury with compromise of the posterior longitudinal ligament.
iv. Anterior cervical diskectomy and fusion with plating is effective as either a primary form of treatment or in patients with dynamic instability after nonoperative treatment.
Special circumstances—extension injuries in ankylosed spines:
i. Patients with ankylosed spines secondary to DISH (diffuse idiopathic skeletal hyperostosis) or seronegative inflammatory spondyloarthropathies (e.g., ankylosing spondylitis) have poor tolerance for even nominal extension forces and typically sustain either AO type B3 (hyperextension without translation [▶ Fig. 49.16 ] or AO type C [with translation]; ▶ Fig. 49.17 ) injuries.
ii. These fractures usually occur in the lower cervical spine and can be highly unstable despite being minimally displaced and difficult to identify. The resulting delay in diagnosis can result in potentially catastrophic neurologic consequences.
iii. Patients with ankylosis of the cervical spine who present with neck pain and no obvious acute injury on initial radiographic assessment should be evaluated with a thorough imaging workup including CT and possibly MRI.
iv. If a spine fracture is identified in this patient population, noncontiguous injuries should be sought with multiplanar CT and possibly MRI of the entire spine.
Treatment of hyperextension injuries in patients with ankylosing spine conditions:
Patients with ankylosing conditions who sustain extension injuries should be treated with urgent decompression of the spinal canal, if warranted, which can usually be achieved through closed spinal realignment techniques.
Standard longitudinal tong traction should be avoided because of the potential to further extend and potentially distract these three column injuries.
Provisional immobilization in a halo vest after realignment of the spine to its native, usually kyphotic position or support on a stack of towels can be useful.
Due to their elevated risk of developing epidural hematoma, patients with ankylosing spondylitis or DISH and neurological deficits should have an MRI of the cervical spine at the earliest opportunity.
Mortality rates of greater than 30%, neurological morbidity rates of greater than 50%, and a high likelihood of pulmonary complications have been reported in patients with ankylosing spondylitis who sustain cervical spine fractures, all of which increase in elderly patients.
Because of the high complication rates in nonoperatively treated patients, however, an aggressive surgical approach is recommended.
Canal decompression and stabilization is best achieved by posterior multisegmental instrumentation using plate or rod–screw systems.
Anterior fixation alone is generally not recommended due to the difficulty with achieving adequate screw fixation in the usually osteoporotic vertebral bodies.
Extension injuries without radiographic abnormality—central cord syndrome:
Forced neck hyperextension may cause SCI from compression of the spinal cord between the infolded ligamentum flavum posteriorly and diskosteophytes anteriorly.
SCI can be complete or incomplete.
Although central cord syndrome is the most common SCI under these circumstances, other types of incomplete SCI may result.
The diagnosis of central cord syndrome is contingent on substantially worse upper than lower extremity function.
The prognosis for recovery after central cord syndrome is thought to be good, although patients frequently have significant residual hand dysfunction and spasticity.
Treatment is contingent on the severity of SCI and canal compression.
Initial nonoperative treatment is appropriate in patients with minor, improving neurological deficits, although eventual surgical intervention is typically required to address the causative underlying stenosis.
Early surgical intervention is appropriate in patients with ongoing compression who have severe, progressive, or nonimproving neurological deficits.
i. The surgical approach depends on the location of compression (e.g., anterior vs. posterior), the number of levels involved, cervical alignment, associated instability patterns, and preexisting conditions (e.g., metabolic bone disease, comorbidities).
Additional subaxial spine injuries: Distraction injuries
Distraction injuries of the subaxial cervical spine are extremely unstable high-energy injuries that result in tension failure of all three columns (▶ Fig. 49.21 ).
Fig. 49.21 (a) Sagittal CT angiogram image of distraction injury at C4–C5 with associated quadriplegia. (b) Radiographs 1 year postoperatively demonstrate a healed fusion with acceptable cervical alignment.
Diskoligamentous injury with or without avulsion fracture is most common.
Their severity and associated translational displacement classifies them as AO type C injuries.
In addition to resuscitation measures, early treatment should focus on the evaluation and treatment of spinal cord and vertebral artery injury.
Surgical stabilization is invariably required, with posterior reduction and stabilization being the preferred method.
Secondary anterior cervical diskectomy and interbody fusion may be considered, but are not routinely necessary.
Additional subaxial spine injuries: Lateral compression injuries
Lateral compression injuries are unusual and occur mainly as a result of motor vehicle collisions and sports-related mechanisms.
They typically involve unilateral arch fracture with ipsilateral vertebral body compression, with possible contralateral posterior facet widening..
SCI is unusual, though the causative mechanism of lateral flexion of the neck results in frequent traction injuries of the contralateral nerve roots and brachial plexus.
Higher-grade injuries with more severe coronal plane deformity and associated facet subluxation may require operative intervention.
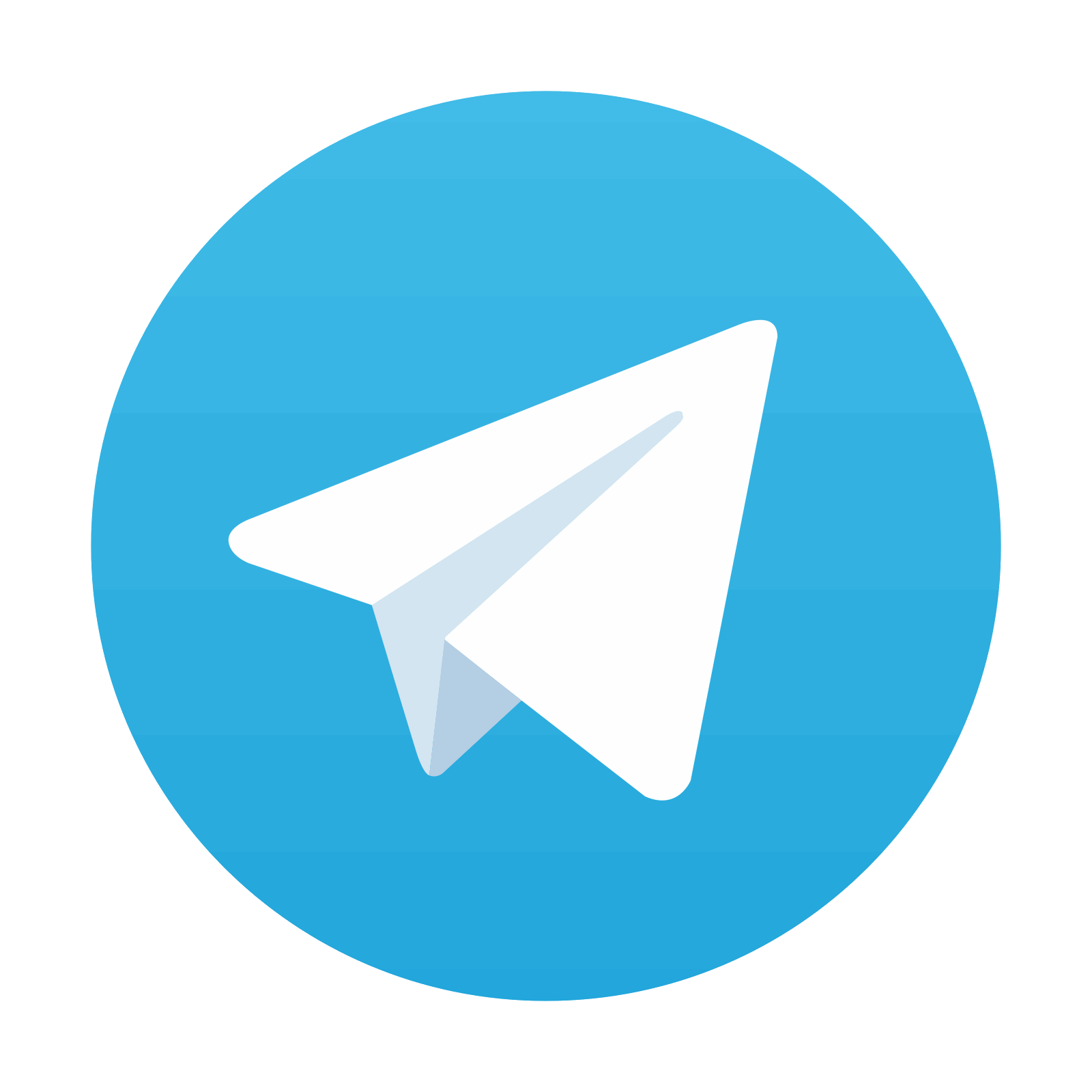
Stay updated, free articles. Join our Telegram channel

Full access? Get Clinical Tree
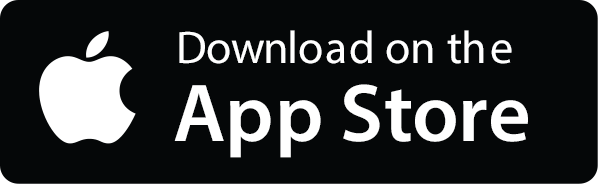
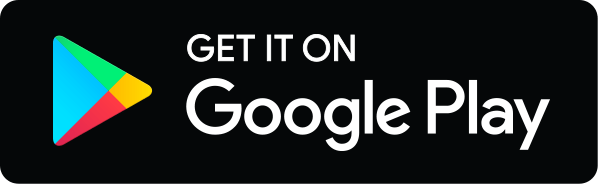