48 Use of Growth Factors in Musculoskeletal Research
Growth factors (GFs) are naturally occurring substances that bind to receptors on the cell surface and are capable of stimulating diverse cellular processes including proliferation and differentiation. GFs are usually a protein or hormone and most are pleiotropic, causing multiple biological effects with some stimulating changes in numerous cell types whereas others are specific to a particular cell type. Individual GFs tend to occur as members of larger families of structurally and evolutionarily related proteins. Although the term cytokine is sometimes used interchangeably with GF, cytokines are a unique family of GFs secreted primarily from leukocytes that stimulate both the humeral and immune responses, as well as activation of phagocytes.
GFs are known to play an important role in both normal and abnormal skeletal growth and development. Indeed, some of the most dramatic successes in pediatric orthopedics have resulted from the identification of deficiencies in cell signaling molecules that are crucial to skeletal development, such as vitamin D in rickets, thyroid hormone in cretinism, and growth hormone in hypopituitarism. The importance of GFs in skeletal development has been emphasized by the recent discoveries that the skeletal deformities of achondroplasia, Apert syndrome, Crouzon syndrome, Pfeiffer syndrome, and Jackson-Weiss syndrome are all caused by mutations for the fibroblast GF (FGF) family of signaling molecules. However, the systemic roles of GFs in development are beyond the scope of the current chapter, which will focus on applications involving the local delivery of GFs in isolation or in combinations with mesenchymal stem cells (MSCs) for tissue regeneration and healing.
48.1 Mechanism by Which Growth Factors Regulate Cell Behavior
Although GFs differ in their specific mechanisms of action on the basis of their structure and target cells, the mechanisms by which these proteins modify gene expression and ultimately promote gene translation into the proteins of interest can be generalized (Fig. 48.1). GFs bind with large, specific transmembrane receptor molecules present on the surface of target cells. These receptors convert information carried by GFs into a form usable by the cell. As such, the presence or absence of specific functional receptors defines a cell′s ability to respond to signals in the extracellular environment. Receptors may also add to the information carried by the signaling molecule, thereby integrating intracellular information to the delivered message. GF receptors are linked to various genes in the nucleus by a cascade of reactions in the cytoplasm, resulting in cell division or transcription of deoxyribonucleic acid sequences to messenger ribonucleic acid and protein production. Stimulation of this cascade often activates several genes and may therefore generate multiple effects. Each family of GF has a corresponding family of receptors. Although there are marked structural differences among receptor families, many of the links in the gene-activating cascade are shared between families. Therefore, binding of different GFs to their respective receptors may result in the same cellular effect. GFs may act through endocrine, paracrine, and autocrine regulation. In the endocrine pathway, cell-signaling molecules are released by the secreting cell into the circulation to act on distant target cells. In the paracrine pathway, the cell-signaling molecules are secreted locally to act on neighboring cells. The autocrine pathway is characterized by cellular self-activation.

48.2 Actions of Growth Factors
In contributing to tissue healing and regeneration, GFs may exert effects in five broad categories. The majority of GFs contribute to regeneration through several of these actions.
Cellular proliferation: At the site of injury, GFs may stimulate proliferation of progenitors to ensure that the pool of progenitors lost through differentiation is replenished.
Cell migration: Tissue formation during healing requires the orchestrated movement of cells in particular directions to specific locations. Cells often migrate in response to and toward specific signals including GFs in a process known as chemotaxis.
Cell survival at the site of injury: Injured tissue represents a harsh microenvironment where inflammation, low oxygen concentration, and loss of trophic factors may contribute to poor survival of host and transplanted MSCs. GFs contribute to the suppression of harmful inflammation and revival of endogenous tissues. 1
Differentiation of progenitors: GFs are key regulators of lineage fate and its progression. 2
Angiogenesis: Tissue regeneration is dependent on angiogenesis. GFs promote new blood vessel formation essential for the delivery of oxygen, nutrients, and progenitors to the site of injury.
48.3 Growth Factors in Mesenchymal Stem Cell Transplantation
Individual or synergistic combinations of GFs can be delivered directly to sites of injury where they act directly on host cells to bring about their therapeutic effect. However, GFs are increasingly being used in combination with MSCs, whose ability to differentiate into bone, fat, muscle, and cartilage while beneficially modifying local immune environments and creating a regenerative microenvironment has made them a promising substrate for musculoskeletal regeneration.1,2 Concomitant delivery of GFs may augment both the regenerative potential of transplanted MSCs while optimizing a regenerative microenvironment through actions on cells within target tissues.
In addition, GFs are playing an increasing role in the preparation and preconditioning of MSCs prior to delivery. Although MSCs are easy to isolate, relatively safe, and do not require immunosuppression, there currently exists a gap between the number of MSCs that can be obtained from the donor site and the number required for implantation to regenerate tissue. Standard methods of MSC expansion are not fully suitable due to time- and age-related constraints for autologous therapies. Furthermore, experiments in small animals have shown that MSCs do not persist well in the graft environment. Either the cells do not incorporate into the host tissue, or if there is incorporation, the cells are rapidly lost. This is likely to reflect the various threats that MSCs face at the site of delivery leading to loss of cells (Fig. 48.2). Through their effects on proliferation, survival, and differentiation of MSCs, pretreatment with GFs may prove helpful in addressing these issues prior to delivery.

48.4 Selected Growth Factors and Their Actions
Growth factors with potential clinical applications in the augmentation of healing of musculoskeletal and connective tissues can be categorized on the basis of their biological activities and clinical use. They include the transforming growth factor beta (TGFβ) superfamily (includes the TGFβ1–3 and the bone morphogenetic proteins [BMPs] among others), Wnt, FGFs, and epidermal GFs, vascular endothelial GFs, insulinlike GF, and platelet-derived growth factor beta (PDGFβ) (Table 48.1).
48.4.1 Transforming Growth Factor Beta
The TGFβs form part of the wider TGFβ superfamily, which consists of many GFs and morphogens that have roles in skeletogenesis and skeletal homeostasis. This family also includes the BMPs (considered separately subsequently), growth and differentiation factors, anti-Mullerian hormone, and activin.
Although also capable of stimulating osteogenesis, TGFβs (of which there are three isoforms, TGFβ1–3) are best known for their effects on the chondrogenic differentiation of MSCs. 3 TGFβ stimulates cell replication and extracellular matrix formation while inhibiting the alloreactive immune response. In vitro, MSCs exposed to TGFβ show increased proliferation and a bias toward the chondrogenic lineage. While all three isoforms induce proliferation of MSCs and chondrocyte formation, TGFβ3 has the most pronounced effect on chondrogenesis and consistently increases proliferation of MSCs. 4 Cartilage-specific gene expression occurs through intracellular signaling cascades involving SMAD proteins, the mitogen activated protein kinases, p38, extracellular-signal regulated kinase-1, and c-Jun N-terminal kinase. 5
Animal studies have evaluated the influence of TGFβ on the healing of cartilage defects and fractures. Several studies have demonstrated that the effects of TGFβ are highly dose dependent, with high levels favoring chondrogenesis, while low doses favor osteogenic differentiation. Guo et al 6 investigated whether TGFβ1-modified MSCs to enhance the repair of full thickness articular cartilage damage in a rabbit model. The MSCs transfected with TGFβ1 were seeded onto a biomimetic scaffold in vitro and allografted into cartilage defects. The proliferation and generation of cartilaginous matrix by TGFβ1-transfected MSCs was substantially greater than in MSCs without TGFβ1 with evidence of surface hyaline cartilage-specific extracellular matrix synthesis and reconstruction of the subchondral bone at 24 weeks. Park et al 7 described an enhanced neocartilage formation by rabbit MSCs using a hydrogel plus TGFβ1.
Studies have also demonstrated stimulation of bone regeneration by two- to four-fold a following local application of exogenous TGFβ in animal models of implant fixation. 8 These studies demonstrate that the effect of TGFβ1 is highly dose dependent such that intermediate doses cause more bone to form than low or high doses. Using a knockout mouse model, absence of TGFβ1 has also been shown to be detrimental to bone mineralization. 9
TGFβs have yet to be evaluated in clinical settings in orthopedics.
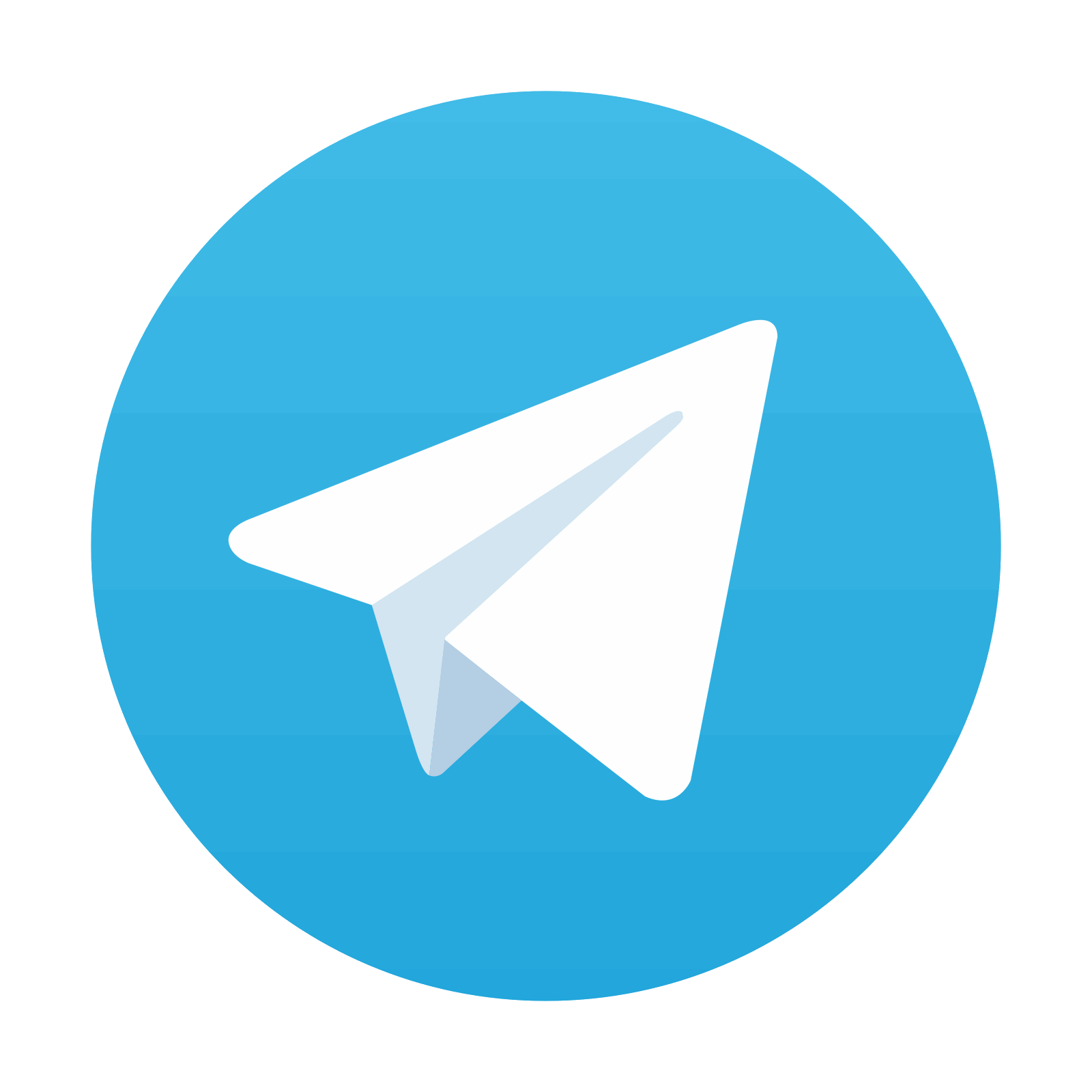
Stay updated, free articles. Join our Telegram channel

Full access? Get Clinical Tree
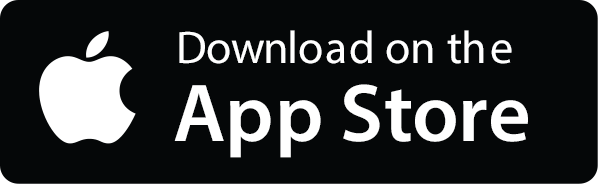
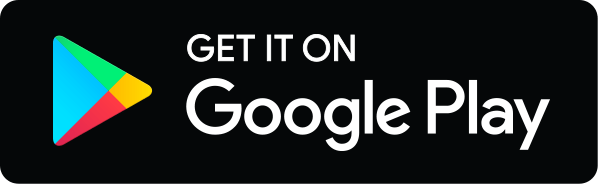
