47 Scaffolds for Tissue Engineering and Materials for Repair
The use of synthetic materials for orthopedic applications is well established and has significantly improved the quality of life in patients suffering from tissue loss and degeneration. To date, the majority of these implants have been metals and ceramics. However, as the global population demographics change and people live longer, patients are now outliving their implants and require successive and often more complex operations to replace them; therefore, alternative materials are required to meet these challenges.
Initial biomaterial and implant research focused on finding inert substrates that could replace lost or damaged tissue, but evoke minimal effects on the native tissue. Coupled with advances in cell-based therapies and the emergence of regenerative medicine, there has been a significant shift in focus from looking at the application of inert materials that replace lost and damaged tissue, to trying to identify biocompatible and bioactive materials that can stimulate and coordinate the replacement and regeneration of tissue.
This chapter focuses on the theory behind the identification of materials that can be used to stimulate growth and differentiation of cells for the purposes of repair and regeneration of orthopedic tissues.
47.1 Identifying the Problem
The first stage is to identify the specific problem you are trying to address and therefore the best possible combination of cells, scaffolds, and growth factors to address this. The potential application for scaffolds and biomaterials in orthopedic tissue engineering is huge, and so are the number of different biomaterials and fabrication methods available (Fig. 47.1).

Therefore, identifying the most appropriate material to the specific need is fundamental because no single biomaterial can satisfy all problems. For example, in addressing massive bone loss, a scaffold may be required to act as a structural replacement to the bone while the cellular component replaces the lost tissue. Here, the scaffold is required to have mechanical strength as well as the bioactive properties necessary to stimulate regeneration. If the scaffold is designed to be biodegradable, it must be designed with a resorption rate that allows the mechanical strength of the scaffold to remain until the cellular component has remodeled and can resume its natural role. In addition, the potential implications of this type of construct are the need for significant periods of in vitro cell culture in a suitable bioreactor to attain the required number of cells prior to differentiation (Fig. 47.2a). However, there are other scenarios where the aim of the scaffold is to allow the ingress of native stem cells, progenitors cells, and blood vessels to repopulate the graft. Hence, the role of the scaffold is to promote ingress and facilitate differentiation of the native cells (e.g., small bone defects, coating of orthopedic implants; Fig. 47.2b). Thirdly, in some situations, the aim might simply be to deliver factors or cells directly to the site of injury to either stimulate, supplement, or replace existing stem cell populations (e.g., nonunions, avascular necrosis; Fig. 47.2c).

47.2 Biomaterials and the Stem Cell Niche
The stem cell niche refers to the immediate environment that surrounds a stem cell and comprises a complex number of factors that provide signals and stimuli to the cell. The sum effects of these signals can maintain the cell in a quiescent state, or at appropriate times cause the cell to proliferate and differentiate depending on the need. Interactions between the cell, its neighbors, the basement membrane, soluble factors, and the extracellular matrix (ECM) are all fundamental in this process. Therefore, it is crucial that we understand these processes and aim to mimic them when considering designing scaffolds and biomaterials. Factors as simple as the shape that the cell is maintained in can have a significant impact on its capacity to differentiate. Maintaining a spherical morphology has been shown to be important in chondrogenesis of mesenchymal stem cells (MSCs), and therefore substrates that maintain cells in a more spherical shape have shown good potential compared to substrates that cause the spreading out of cells into a flattened fibroblastic type morphology. 1 Further studies have demonstrated that the hardness of the substrate on which a stem cell attaches is able to predict the behavior of the cells, with cells preferentially differentiating toward a tissue that mimics the hardness of the substrate on which they are grown.
47.2.1 Example from the Literature: Nanoscale Effects on Cellular Differentiation
An elegant example of the importance of cells and the surface with which they directly interact was shown by the work of Dalby et al. 2 In their study, they used electron beam lithography, a technology used in the manufacture of Blu-Ray disks, to fabricate ultraprecise nanotopographies. The polymethylmethacrylate culture surface was embossed with nanopits of 120 nm diameter and 100 nm depth, and the arrangement of these pits was varied to form five distinct patterns of varying symmetry and disorder (Fig. 47.3).

They demonstrated that variations in the nanotopography had significant effects on MSC attachment, morphology, gene regulation, and protein and mineral production. They observed that using disordered patterns, they were able to stimulate osteogenesis as observed by the increased production of osteopontin, osteocalcin, upregulation of genes specific to osteogenesis, and production of mineralized bone matrix when compared to the ordered and symmetrical patterns. The effects seen by the change in nanopatterning were in the absence of any other inducing agents such as growth factors; however, the distinct differentiation profile that was observed had a similar efficiency to those cultured in osteogenic media. In a similar series of experiments, the same group also identified nanopatterned surfaces that could maintain MSC pheno-type and growth over prolonged periods of culture. 3
47.3 Metals as Biomaterials
Metals form the majority of orthopedic implantable materials that are in current clinical use and are the material that is most routinely used in load-bearing implants and internal fixation devices. However, their design and function has undergone significant change and advancements. When manufactured appropriately, they display high-tensile, high-fatigue, and high-yield strengths with low reactivity of the native tissues. However, these same features that are seen as advantageous are responsible for the ultimate failure of many of these devices.
The high strength of the implant can shield the surrounding tissue from its normal stress and strains. This results in an associated atrophy and weakening of the bone resulting in bone degeneration. Furthermore, due to the inert nature of many metals, there is no osseointegration at the interface between the metal and the host tissue. This results in a layer of connective tissue developing between the implant and the prosthesis, causing a natural weakness. Therefore, much attention has been placed on developing osteoconductive and osteoinductive surfaces that will cause seamless integration of the implant and the host tissue through their influence on native or implanted tissue stem cells (Fig. 47.2b).
Porosity in orthopedic implants is advantageous because it can facilitate tissue adhesion, growth, and if appropriate vascularization, thus turning an inert structure into a bioactive scaffold that integrates with the host. In addition, varying the degree of porosity of an implant will also have effects on the elastic modulus and can be tailored to that of the tissue to prevent issues such as stress shielding.
While most metals are relatively inert and are not biologically active, many of their oxides and alloys show excellent osteoinductive and -conductive properties. There have been many techniques described to increase the bioactivity and osteoconductivity of metallic implants and biomaterials including mechanical methods, plasma sprays, chemical treatments, and surface treatments, all of which aim to create a more bioactive interface.
This would have many significant advantages including rapid tissue integration, enhanced differentiation of stem cell populations, and increased bonding at the tissue-implant interface.
47.3.1 Example from the Literature: Coating of Titanium Implants with Collagen, RGD Peptide, and Chondroitin Sulfate
In an attempt to enhance osteogenesis and improve integration of the implant, the researchers hypothesized that using components of the bone ECM may enhance bone healing and osteogenesis around implants. Using a tibial titanium nail model in rats, they coated the surfaces of the titanium nails with different components of the ECM including type I collagen, chondroitin sulphate, and the RGD peptide sequence (Arg-Gly-Asp). Each of these components is known to be important in osteogenesis and has been previously shown to enhance bone growth and repair when used as a scaffold. Here, the authors chose to coat the surface of the titanium to create an osteoinductice and osteoconductive interface to enhance bone repair and integration.
They demonstrated that the addition of ECM proteins significantly enhanced bone remodeling in the early stages (days 7 and 14), which led to increased new bone formation in the later stages (day 28).
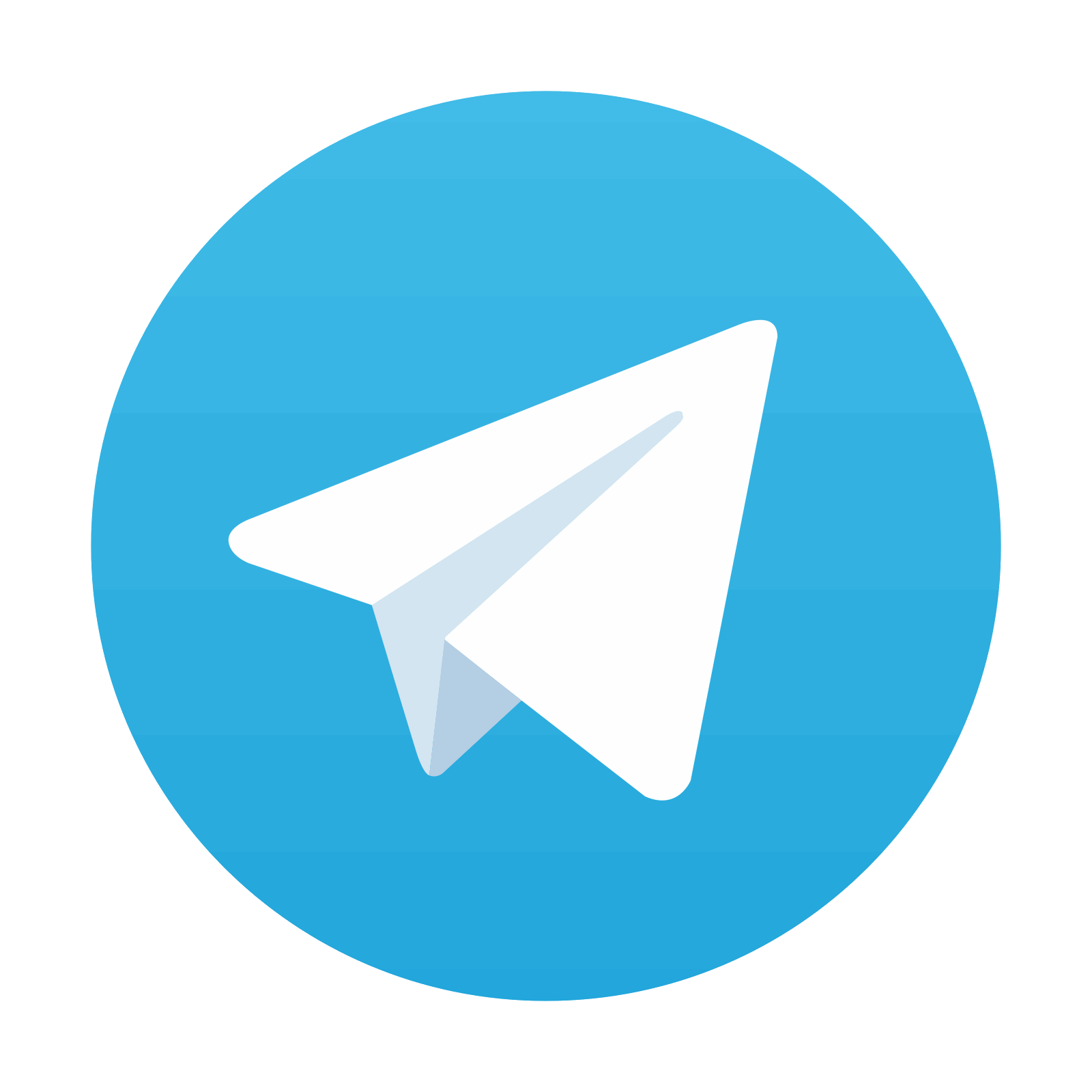
Stay updated, free articles. Join our Telegram channel

Full access? Get Clinical Tree
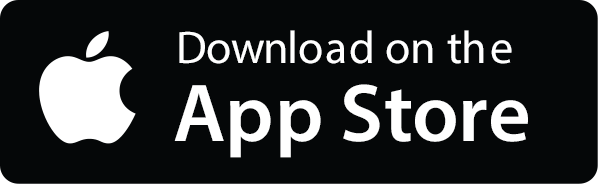
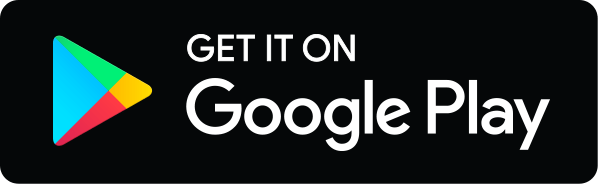
