4 Healing of soft tissues and bone
4.1 Skin and subcutaneous tissue
Jian Farhadi
4.1.1 General aspects of initial tissue response to injury
The normal response to tissue injury is a timely and orderly healing process resulting in restoration of anatomical and functional integrity [1]. Wound healing, however, is not a simple, linear process but rather a complex interaction of dynamic processes involving cell-to-cell and cell-to-matrix activities mediated by humoral messengers ( Fig 4.1-1a ) [2, 3]. Knowledge of the normal physiological process of tissue healing and repair helps to appreciate pathophysiological responses. Wound healing is characterized by three classic phases that, however, do not follow sequentially, but rather partially overlap in time ( Fig 4.1-1b ). The three phases are:
inflammatory or substrate phase (hemostasis and inflammation)
proliferative or fibroblastic phase (cellular migration and proliferation, protein synthesis)
remodeling and maturation phase (wound contraction).
The goal of these well-orchestrated biological processes is the repair of the injured skin and subcutaneous tissue with fibroblast-mediated scar tissue. Various categories of wound healing have been described. However, a distinction must be made between primary and secondary healing. Primary wound healing or healing by first intention ( Fig 4.1-2a–c ) occurs within hours after closing of a surgical incision where wound edges are directly approximated without significant tissue loss (this generally signifies full-thickness) (chapter 10.1). Wound closure is performed with sutures, staples, or adhesive. A surgical incision only destroys a limited number of cellular constituents and scarring is minimal. In contrast, secondary wound healing or healing by secondary intention ( Fig 4.1-3a–c ) is characterized by the formation of granulation tissue, which contracts and finally reepithelializes (chapter 10.1). In such cases the wound may be superficial, intermediate, or full thickness. The different phases of wound healing are more distinct than in primary wound healing. Furthermore, granulation tissue only develops because there is a need for final wound closure. This may result in pronounced contraction of wounds.




4.1.2 Phases of wound healing
Inflammatory or substrate phase
Trauma to living tissue creates a vascular injury and bleeding, which in turn triggers the cellular and molecular responses to initiate hemostasis. The healing process cannot start before hemostasis has been accomplished. Any disturbance at this stage will lead to an impairment of wound healing. Key players in hemostasis are vasoconstriction, platelet aggregation, and fibrin deposition resulting in clot formation, which is primarily composed of embedded blood cells, aggregated platelets and a fibrin mesh [4]. Vasoactive amines are responsible for vasoconstriction, which starts as soon as the epidermis and dermis are penetrated. This process also triggers the secretion of prostaglandins, such as thromboxane. Platelet aggregation is stimulated by tissue factors released by damaged cells. Platelets adhere to the vascular endothelium and to each other in a process involving fibrinogen and von Willebrand factor [5]. Fibrin deposition in the clot seals the wound, preventing further fluid and electrolyte loss, and limiting the risk of contamination from outside. Fibrin is also the endogenous mesh material within the provisional wound matrix into which fibroblasts and other cells migrate as the healing process continues.
Inflammation was first described by John Hunter in 1794 as erythema, edema, heat, and pain (rubor, tumor, calor, and dolor). One of its main functions is to attract inflammatory cells to the injured area in order to repair the damaged tissue. Increased vascular permeability allows leucocytes and macrophages to migrate into the extravascular space starting phagocytosis, destroying bacteria, and eliminating debris in order to allow the repair processes to take over [6].
More explicitly, vasoconstriction is followed within 10–15 minutes after injury by vasodilation. Simultaneously, the endothelial cells lining the capillaries adjacent to the wound form intercellular gaps allowing leakage of plasma and cells into the extravascular space. The inflammatory phase is initiated and regulated by the release of numerous cytokines: α-granules liberate platelet-derived growth factor (PDGF), platelet factor IV, and transforming growth factor β (TGF-β), while vasoactive amines such as histamine and serotonin are released from dense bodies found in platelets. Platelet-derived growth factor acts as a chemotactic agent for fibroblasts and, along with transforming growth factor β, is a potent modulator of fibroblastic mitosis, leading to prolific collagen fibril construction in later phases. Fibrinogen is converted to fibrin and the framework for completion of the coagulation process is formed. Fibrin provides the structural support for the cellular constituents of inflammation. This process starts immediately after the insult and may continue for a few days.
Within the first 6–8 hours, the next phase of proliferation sets in, with polymorphonuclear leukocytes (PMN) engorging the wound. Transforming growth factor β facilitates migration of polymorphonuclear leukocytes from surrounding blood vessels into the extracellular space. The highest concentration of polymorphonuclear leukocytes is reached 24–48 hours after tissue injury. The start of their activity correlates with the termination of the inflammatory phase [7]. An imbalance of bacteria against polymorphonuclear leukocytes may lead to an acute infection, which becomes clinically visible after ~ 72 hours.
Proliferative or fibroblastic phase
Even before the inflammatory phase has come to an end, ie, 2–3 days after injury, fibroblasts begin to enter the wound, marking the onset of the proliferative phase that will last up to 14 days [2]. The process of angiogenesis starts concurrently with fibroblast proliferation when endothelial cells migrate to the area of the wound in order to supply the tissue with oxygen and other nutrients. The tissue in which angiogenesis has set in typically looks red due to the presence of numerous newly developed microvessels. Key factors in the angiogenic process include high lactate levels, acidic pH, and, in particular, decreased oxygen tension [8]. Angiogenesis is initiated by endothelial buds or sprouts deriving from preexisting, intact capillaries at the wound periphery and possibly from the wound ground [9]. These buds or sprouts grow by cellular migration and proliferation and may eventually come into contact with a bud or a sprout stemming from another nearby capillary. They interconnect and generate a new functional capillary network, ie, offering passage for cellular components such as erythrocytes and leukocytes. The two most important cytokines that contribute to angiogenesis are fibroblast growth factor 2 (FGF-2) and vascular endothelial growth factor (VEGF).
The resulting granulation tissue, which is typical for secondary wound healing, functions as rudimentary tissue. It first appears in the wound as early as the inflammatory phase, 2–5 days postinjury, and continues to grow until the wound bed is covered. Beside fibroblasts, inflammatory cells, endothelial cells, and myofibroblasts, granulation tissue consists of a new, provisional extracellular matrix as well as newly formed, patent blood vessels. Granulation tissue approximately contains 30% type III collagen.
Finally, reepithelialization of the wound is crucial for the reestablishment of the barrier function of the skin. Incisional skin injuries, with a minimal epithelial gap are typically reepithelialized within 24–48 hours. During the first 24 hours after injury, basal cells appear at the wound edge and begin to migrate across the denuded wound surface. If the initial injury does not destroy epithelial appendages such as hair follicles, sebaceous and sweat glands, these structures also contribute migratory epithelial cells to the healing process. The migration of epithelial cells continues until there is an overlap with other epithelial cells migrating from different directions. When two epithelial cells meet, “contact inhibition” stops their movement [10].
Remodeling and maturation phase
The quality and quantity of matrix deposited during this phase of healing significantly influences the strength of a scar [11]. Collagen constitutes more than 50% of the protein in scar tissue, and its production is essential to the healing process. Fibroblasts are responsible for the synthesis of collagen and other regenerative proteins during the repair process. Collagen synthesis is stimulated by transforming growth factor β, platelet-derived growth factors, and epidermal growth factors [12, 13]. Collagen synthesis also depends on the wound and the characteristic constitution of the patient′s body including age, tension and pressure (chapter 4.4). Collagen synthesis continues at a maximum rate for 2–4 weeks and subsequently begins to slow down. Disturbed healing resulting in chronic wounds often is the result of aberrations in collagen deposition, for example, as observed in diabetic patients or smokers (chapter 4.4). Conversely, hypertrophic scar or keloid formations result from excessive collagen synthesis.
Initially the wound matrix is primarily composed of fibrin and fibronectin, which are gradually replaced by collagen and other proteins such as proteoglycans, the key components of a mature matrix. Additional proteins such as thrombospondin I and secreted protein acidic rich in cysteine (SPARC), which support cellular recruitment and stimulate wound remodeling, are also produced and found in the mature wound matrix [14]. The concentration of collagen subtypes varies among tissues. Type I collagen predominates and makes up 80–90% of the collagen seen in intact dermis. The remaining 10–20% consist of type III collagen.
Remodeling of the scar begins to dominate the wound-healing activities ~ 3 weeks after injury and can continue for up to 2 years. The rate of collagen synthesis reaches a peak by the third week, then starts to decrease and levels off at a balance with the rate of collagen breakdown. The downregulation of collagen synthesis is mediated by γ-interferon [15], tumor necrosis factor α (TNF-α) [16] and the collagen matrix itself [17]. Contraction of the wound is an ongoing process resulting in part from the proliferation of the specialized fibroblasts termed myofibroblasts, which resemble contractile, smooth muscle cells. Wound contraction occurs to a greater extent with secondary healing than with primary healing and greatly depends on the rate of fibroblasts differentiating into myofibroblasts ( Fig 4.1-3b ). Maximal tensile strength of the wound is achieved by the twelfth week. The ultimate resultant scar only has 80% of the tensile strength of the original skin, which has been replaced [18, 19].
4.2 Muscle and tendon
Douglas W Lundy
4.2.1 Healing of muscle
The forces involved in producing a fracture almost always cause some degree of injury to the surrounding soft-tissue envelope. This injury can range from very minimal to a severe lesion with cavitation and loss of entire motor units (chapter 3).
The repair process is characterized by three phases, which run concurrently, respectively overlap in time (chapter 4.1). Immediately after injury, the damaged muscle enters the inflammatory phase. Proteases begin to degrade the necrotic portions of the muscle. As the surrounding vascular system has also been damaged, inflammatory cells invade the zone of injury from the lacerated vessels. These cells include macrophages, polymorphonuclear leukocytes and lymphocytes that release chemotactic factors and continue to enhance the inflammatory response ( Fig 4.2-1 ). Interestingly, the local area affected by the injury is topographically contained by contraction bands that limit the repair process to the injured area. This intensifies the inflammatory effect within the zone of injury and protects normal tissue from the inflammatory cascade (chapter 10.3.3) [20, 21].
The proliferative phase starts at the earliest 7–10 days after the insult. The cytokine stimulus from the inflammatory cells triggers satellite cells that are dormant in the basal lamina. These muscle progenitor cells are activated and join the damaged muscle cells to promote healing and reconstitution of the muscle unit. This process of regeneration is at its climax ~ 2 weeks following the injury ( Fig 4.2-2 ) and concludes ~ 2 weeks later [20, 21].

The formation of scar tissue within the damaged muscle is the hallmark of the final phase of remodeling and maturation ( Fig 4.2-3 ). Granulation tissue is formed very early in the repair process under the influence of fibronectin and fibrin released from the hematoma. Fibroblasts migrate to the injured area and predominantly secrete fibronectin that subsequently increases the strength and elasticity of the repair tissue. As the proliferative phase draws to an end, the fibroblasts begin to produce type I collagen. Transforming growth factor β (TGF-β) and other growth factors stimulate this response, and collagen type I strengthens the fibrotic scar. The remodeling of the muscle is complete once the fibrotic scar has matured. However, the muscle never returns to its preinjury state as it will always be bridged by scar tissue [20, 21].
Healing of muscle is improved by a brief period of immobilization after injury. This helps to minimize contraction of the muscle edges as well as to decrease the size of the hematoma, which subsequently results in a smaller fibrotic scar [22].


Extensive muscle loss will obviously hamper the function of a particular muscle unit. If there are other muscles remaining, the injured muscle unit may still be able to contribute to the overall function. However, the joint affected by the muscle loss may subsequently suffer from an imbalance of forces since formerly antagonistic muscle groups are now relatively unopposed.
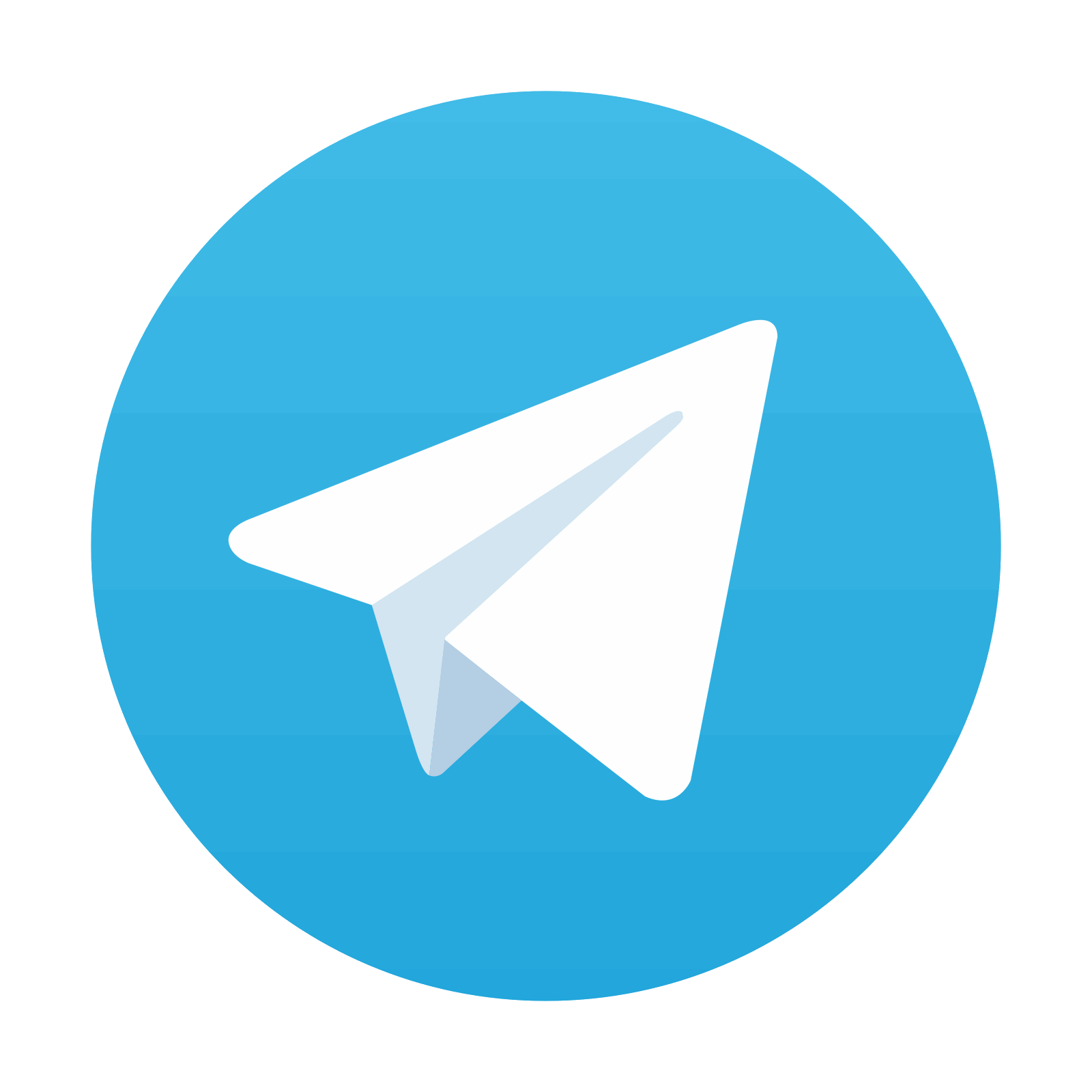
Stay updated, free articles. Join our Telegram channel

Full access? Get Clinical Tree
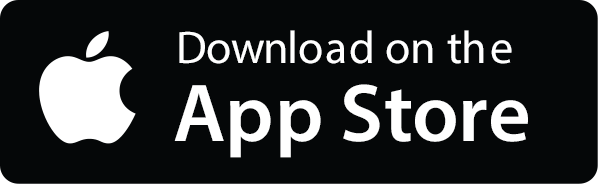
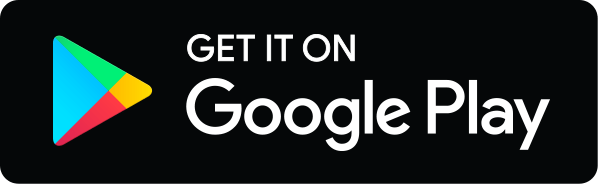
