4 Cellular Chondroplasty: A New Technology for Joint Regeneration
4.1 Introduction
Cellular therapy involves the isolation, expansion, and transplantation of human cells for the replacement or regeneration of injured tissues. It is one of the most significant tissue repair strategies in the emerging discipline of regenerative medicine. The technical elements of cellular therapy, the isolation, expansion, cryopreservation, and delivery of viable cells, have their origins in work over preceding decades in transplant medicine. The transplantation of whole organs, including kidney, lung, liver, and heart depended on the development of new surgical techniques and protocols for immunosuppression. This new medicine also depended critically on the development of methods for preserving the viability of tissues and cells ex vivo. Ultimately this has led to the use of culture-expanded cell preparations (either patient derived or donor derived) for tissue repair and there are several examples of such therapies that have received regulatory approval in recent years. These include Apligraf, 1 the first approved allogeneic cellular product marketed as a skin equivalent; Carticel, 2 an autologous chondrocyte implant for cartilage repair; Provenge, 3 a cellular immunotherapy consisting of the patient’s own dendritic cells for the treatment of late-stage prostate cancer; and Prochymal, 4 a mesenchymal stem cell (MSC) treatment for acute graft versus host disease.
Cellular strategies have also been applied in joint repair for the treatment of acute cartilage injury, meniscal repair, and for osteoarthritis (OA), with some measurable success (for review, see Barry and Murphy 5 ). Many of these efforts have been motivated by the apparent incapacity of cartilage to repair itself 6 and the observation that an initial trauma is likely to lead to sustained degeneration of the joint over many decades. 7 Further motivation for these new cell engineering strategies was provided by the complexities and variable success of osteochondral allografts. 8 The cell types that have been tested in joint cell therapy have been mainly either chondrocytes or MSCs.
4.2 Chondrocyte Transplantation
Chondrocytes have been widely studied and one of the early efforts in this regard was that described by Grande et al 9 to investigate the healing effects on injured cartilage of chondrocytes grown in vitro. This study showed that defects in rabbit articular cartilage which had received autologous chondrocyte transplants had a significant amount of cartilage reconstituted compared with ungrafted controls. In many ways, this was a pioneering effort in the new field of cellular therapy and it opened the door to a multiplicity of new studies, new technologies, and exciting outcomes. A landmark in testing chondrocyte transplantation for patients was reported by Brittberg et al. 10 These researchers isolated and cultured chondrocytes obtained from an intact area of the injured knee during arthroscopy and treated patients ranging in age from 14 to 48 years with full-thickness cartilage defects that ranged in size from 1.6 to 6.5 cm2. The cultured chondrocytes were injected into the defects which were covered with a sutured periosteal flap taken from the proximal medial tibia. Fourteen of 16 patients with femoral condylar transplants had good-to-excellent results and biopsies indicated that a large proportion of femoral transplants had a hyaline appearance.
Two new paradigms in tissue engineering were thus incorporated into these procedures, namely, the use of expanded autologous cells and the use of a biomaterial to enhance cell delivery and retention. In this case, the biomaterial was a flap of autologous periosteum sutured to the defect to retain the cells. A variation of this strategy, arising from the additional morbidities associated with periosteal harvesting, was the use of collagen membranes in the so-called matrix-induced autologous chondrocyte implantation (MACI). In this technique, the cultured chondrocytes were seeded on a collagen membrane which was implanted into the defect using fibrin glue. 11
Several attempts have been made recently to assess, either in a clinical trial or in a retrospective fashion, the outcome of autologous chondrocyte transplantation procedures. In a major recent study, 12 827 of 869 patients who had undergone autologous chondrocyte implantation (ACI) with periosteum or MACI were evaluated in terms of functional outcomes. The study showed that overall graft survival was 78% at 5 years and 51% beyond 10 years and suggested that ACI for the treatment of osteochondral defects of the knee was an effective approach. Similarly, Macmull et al looked at the outcome of ACI in 31 adolescent patients. 13 They found an improvement in pain scores and functional testing. The authors concluded that ACI was appropriate in the management of carefully selected adolescents with symptomatic chondral and osteochondral defects. Schneider et al 14 also examined 116 patients (49 women and 67 men of mean age 32.5 ± 8.9 years). They found an improvement in functional scores and pain levels in patients when they compared preoperative to postoperative levels. These researchers also concluded that it was a safe and clinically effective treatment.
Despite this success, it does appear that ACI is limited to acute, defined chondral lesions and is unlikely to provide a regenerative solution for more severe joint conditions such as those associated with OA. It may also be the case that ACI, while it stimulates a cartilage repair response, may have limited ability to alter the environment of the injured joint in a more globally regenerative way. There is an additional concern relating to the requirement to harvest intact cartilage as a source of autologous chondrocytes. ACI is a cell replacement technology whereby the transplanted chondrocytes provide cellular building blocks for replacement tissue. When applied to a defined lesion, this makes sense. In the context of more extensive degenerative joint disease, it may have less application and alternative cellular strategies are needed.
4.3 Mesenchymal Stem Cells
MSCs may provide such an alternative cellular strategy in cartilage repair. 5 Here we discuss the biological properties of MSCs and their potential role in maintaining joint homeostasis. We also explore the evidence which suggests that MSC transplantation may contribute to joint health.
MSCs are precursors of connective tissue cells and can be isolated from many adult organs. Much of what we currently understand about MSC biology derives from the 1960s and work by Friedenstein et al on fibroblastic cells with the capacity to differentiate into osteocytes from the stromal compartment of bone marrow. 15 , 16 These cells adhered to tissue culture plastic and were able to form colonies from a single cell, often referred to as colony-forming units fibroblastic. They were also capable of generating multiple skeletal tissues in vivo. 17 MSCs have since been isolated and characterized from many other human sources including adipose tissue, 18 skeletal muscle, 19 umbilical cord blood, and Wharton jelly. 20 , 21 MSCs from many tissues share the capacity to differentiate into cells of connective tissue lineages in vitro, most notably bone, fat, cartilage, and muscle. The wide tissue distribution of MSCs suggests that they derive from a perivascular niche 19 and several isolation techniques have been used to identify clonal progenitor cells in blood vessels in various tissues which have characteristics identifying them as MSCs. 22
A series of phenotypic definitions has been proposed for these cells based on surface marker expression, differentiation potential, and the ability to secrete paracrine factors (Table 4.1). The purpose of these definitions is to (1) understand the biological attributes of isolated and expanded MSCs, (2) understand how these may associate with the capacity of the cells to stimulate a repair response when transplanted in vivo, and (3) provide release tests that will be applied when preparing cell products that will be assessed clinically. Several problems are immediately evident. While the currently proposed surface markers 22 provide accessible testing strategies and have been proposed as measures of “potency,” it is not at all clear if they bear any relationship to the in vivo activity of the cells. Nor do they provide any means of measuring heterogeneity, a problem that may hamper efforts at preparing and testing MSCs for larger studies. Furthermore, the capacity of MSCs to differentiate and to define lineages may have little relevance in terms of their therapeutic activity. In fact, there is a growing understanding that the fundamental property of MSCs that gives them therapeutic potential may be their capacity to secrete potent factors at the site of injury. Consequently, there may be a need to define new parameters for testing MSCs for clinical use based primarily on their secreted profile.
Surface markers | Differentiation potential | Secreted factors |
CD44+ | Osteogenic | VEGF, Ang-1, SDF-1, PDGF, TSG-6, bFGF, FGF-7, IL-1, IL-6, IL-10, MCP-1, TGFβ, PGE-2, IDO, M-CSF, HGF, MMP-9, SFRP, thymosin β4, plasminogen, Tenascin C, thrombospondin |
CD73+ | Adipogenic | |
CD90+ | Chondrogenic | |
CD105+ | Myogenic | |
CD11 b– | ||
CD14– | ||
CD34– | ||
CD45– | ||
CD79a– | ||
HLA-DR– | ||
Abbreviations: VEGF, vascular endothelial growth factor; Ang-1, angiopoietin 1; SDF-1, stromal cell-derived factor 1 ; PDGF, platelet-derived growth factor; TSG-6, tumor necrosis factor-inducible gene 6 protein; bFGF, basic fibroblast growth factor; FGF-7, fibroblast growth factor 7; IL-1, interleukin 1; MCP-1, monocyte chemotactic protein 1; TGFβ, transforming growth factor beta 1; PGE-2, prostaglandin E2; IDO, indoleamine 2,3-dioxygenase; M-CSF, macrophage colony-stimulating factor; HGF, hepatocyte growth factor; MMP-9, matrix metalloproteinase 9; SFRP, secreted frizzled-related protein 2. |
4.3.1 Mesenchymal Stem Cells in the Joint
There are several compelling reasons why MSCs may be useful agents of joint repair. The first arises from a series of studies performed by several groups, assessing the nature of endogenous MSC populations within joint tissues. It is evident that every tissue within the knee contains a reservoir of MSCs or MSC-like cells. Self-renewing MSCs with a potential to differentiate along the chondrogenic, osteogenic, and adipogenic pathways were described in the adult human synovial membrane by De Bari et al. 23 They have also been detected in the synovial fluid compartment in healthy and OA joints. 24 , 25 Interestingly, the number of cells recovered in the synovial fluid is increased in OA and rheumatoid arthritis patients 26 and following ligament injury. 27 The yield of cells also seems to increase with severity of disease. 28 MSCs can also be readily isolated from the synovial fat pad 29 and from the anterior cruciate ligament. 30 MSCs also reside in the meniscus, 31 form colonies efficiently, and possess strong chondrogenic activity. Mesenchymal-like progenitor cells have also been described in the surface zone of articular cartilage. 32 These were true precursor cells, distinct from dedifferentiated chondrocytes isolated from the same tissue, being clonogenic and proliferative and possessing high telomerase activity. 33 These cells also display trilineage differentiation potential. 34
Thus, it is clear that every tissue within the diarthrodial joint contains a reservoir of MSCs. While the biological activity of these tissue populations is not fully defined, it is certainly possible to generate some speculative ideas about their function. It seems likely that they contribute to (1) joint development and (2) tissue turnover and homeostasis by providing reservoirs of progenitor cells and (3) act as immunomodulatory or anti-inflammatory sentinels.
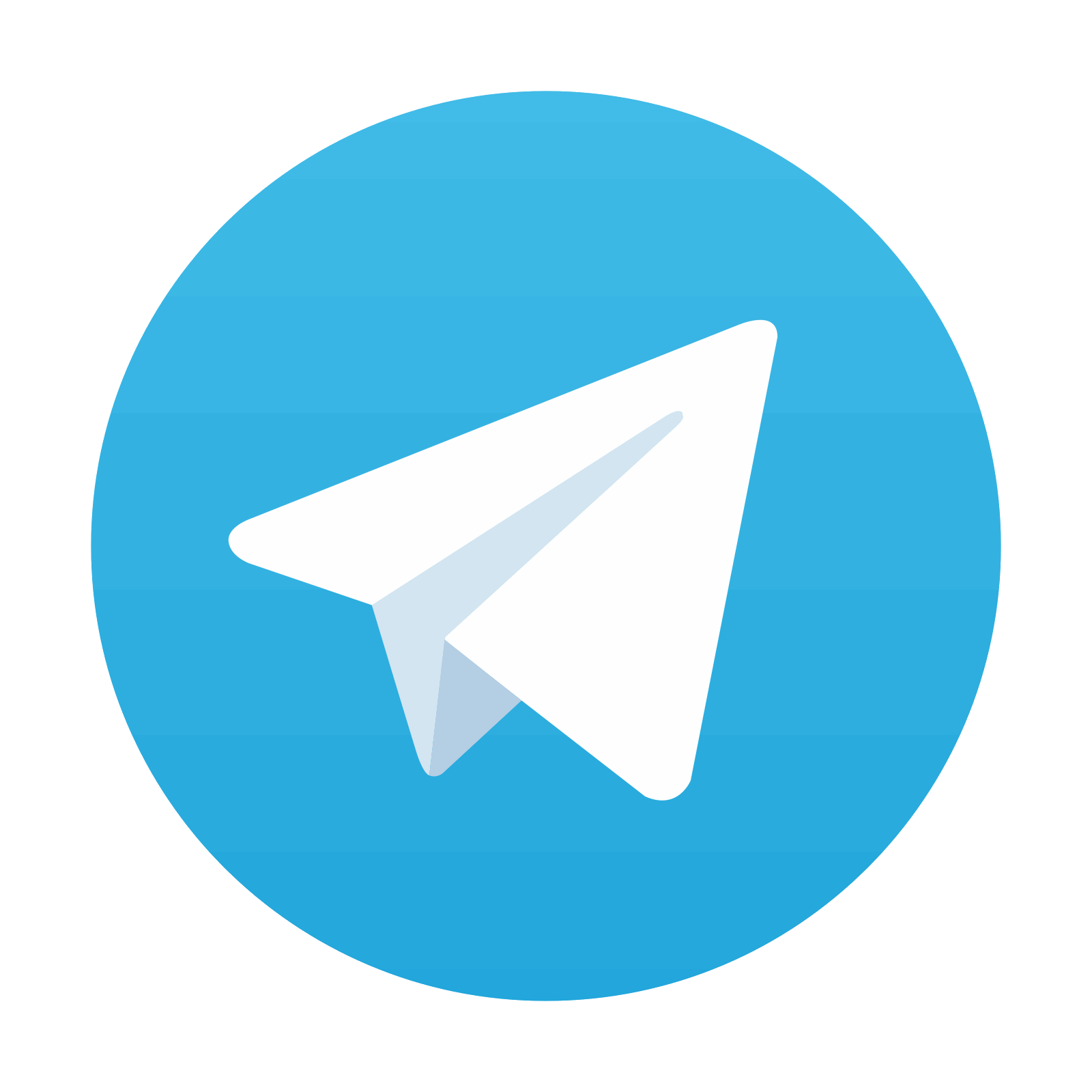
Stay updated, free articles. Join our Telegram channel

Full access? Get Clinical Tree
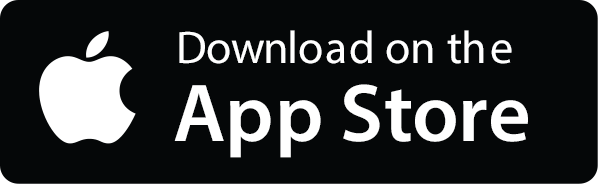
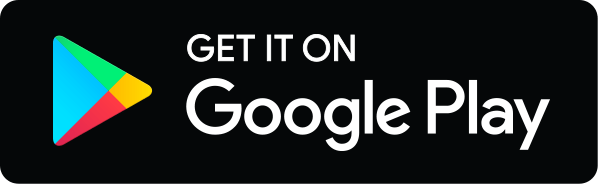
