39 Molecular Testing
Measuring expression of specific proteins can be valuable when assessing local reactions at fracture sites or when assessing progression of healing. Proteins can be measured directly, either at a tissue level (using immunohisto-chemistry) or in homogenized tissues (using Western blotting or enzyme-linked immunosorbent assays), but expression can also be measured at the deoxyribonucleic acid (DNA) or ribonucleic acid (RNA) level. Depending on the research question, one may be interested in studying a single gene, but more complex research questions may also require studying the entire genome. Single gene studies are most applicable for measuring changes in expression of a single gene over time, or for studying differences in gene expression between treatments. They are generally regarded as hypothesis-testing studies. Genome-wide assessments are preferable in hypothesis-generating studies, for instance for identifying which gene expression pathways are switched on or off in particular patients or treatment groups. This may help, for instance, in visualizing what the difference in gene expression is in patients that progress to higher disease stages versus those that remain stable, or in patients who show impaired fracture healing versus those who heal uncomplicated. This chapter provides an overview of frequently used molecular techniques in trauma and orthopedic surgery.
39.1 Isolation of Nucleic Acids
Isolation and purification of DNA or RNA is a critical step, because it delivers the input material for all molecular analyses. DNA and RNA can be isolated from any living or dead organism. Common sources used in orthopedic trauma research include whole blood, bone, or tissue samples like biopsies or surgical specimens. The preferred isolation method depends on the source, age, and size of the sample. Despite differences between the methods, their basic principle is to separate DNA or RNA present in the cell from other cellular components.
39.1.1 Deoxyribonucleic Acid Isolation
DNA isolation is a routine procedure. First, cell lysis breaks cells open and allows for release of nucleic acids from the nucleus. This can be achieved by chemical and physical blending, grinding, or sonicating the sample. Hard tissues like bone require mechanical homogenization. Lysis is carried out in a salt solution that contains detergents (e.g., sodium dodecyl sulphate, for dissolving membrane lipids) and proteases (e.g., proteinase K, for denaturing proteins). Adding RNase is optional but advisable if the molecular assay detects RNA.
Next, DNA needs to be purified, because the presence of proteins, lipids, polysaccharides, and (in)organic compounds in the DNA preparation can interfere with molecular assays. The oldest methods to separate DNA from protein use organic solvents. Lysed samples are mixed with phenol, chloroform, and isoamylalcohol to denature proteins. After centrifugation, DNA is retained in the aqueous (upper) layer, whereas phenol is at the bottom of the tube and denatured proteins form a cloudy interface. DNA is precipitated with alcohol (ice-cold ethanol or isopropanol), resulting in a DNA pellet after centrifugation. After washing the pellet with ultrapure alcohol four to five times, DNA is resolubilized in Tris–ethylenediaminetetraacetic acid (EDTA) or ultra-pure water.
Alternatively, commercial DNA isolation kits can be used. The common lysis solution contains sodium chloride, Tris (to retain constant pH), ethylenediaminetetraacetic acid (binds metal ions), sodium dodecyl sulphate (detergent), and proteinase K. DNA purification involves spin columns, which are packed with ion exchange or silica-based matrices that bind negatively charged DNA. Unbound material is removed with washing buffer, and DNA is eluted using water or a neutral pH salt solution to break down the resin-DNA bonding. Commercial isolation kits tend to take a shorter time and result in higher yield of the purified DNA.
Isolated DNA can be stored at 4°C for short-term storage or at −20°C to −80°C for intermediate-term storage. Long-term storage (more than 1 year) is best done in liquid nitrogen.
39.1.2 Ribonucleic Acid Isolation
RNA isolation follows essentially the same protocol as DNA isolation; however, protecting RNA from degradation by adding an RNase inhibitory agent is critical. The method of cell or tissue disruption affects the yield and quality of the isolated RNA. RNA is best protected if the lysis buffer is in contact with cellular contents immediately when the cells are disrupted. This could be problematic if the tissue sample is hard (e.g., bone) or if sample processing is delayed (e.g., it takes time to go to the laboratory). Therefore, samples should be frozen in liquid nitrogen or on dry ice immediately after harvesting. Larger samples may require homogenization in a dounce homogenizer. Storing samples in an RNA stabilizing solution like RNAlater reagent (storage at 4°C; Sigma-Aldrich, St. Louis, MO), RNAlater-ICE (Sigma-Aldrich), or TRIzol (storage at −20°C or −80°C; Life Technologies, Carlsbad, CA) allows postponement of RNA isolation up to months without compromising the RNA integrity.
Three techniques can be used for the subsequent RNA extraction: (1) In organic extraction methods, samples are homogenized in a phenol-containing solution. Centrifugation separates the sample into a lower organic phase, a middle phase that contains denatured proteins and genomic DNA, and an upper aqueous phase that contains RNA. RNA is collected from the aqueous phase by alcohol precipitation and rehydration. (2) Filter-based, spin basket formats are the second option: Following lysis in an RNase inhibitor–containing buffer, the lysate is loaded onto a membrane (glass fiber, silica, or ion exchange membrane) that binds RNA. Centrifugation passes unbound lysate through the membrane. After washing, RNA is eluted from the membrane and collected in a tube by centrifugation. (3) In magnetic particle methods, samples are lysed in a solution containing RNase inhibitors and allowed to bind to 0.5 to 1 µm magnetic particles. Magnetic particles with bound RNA are collected by applying a magnetic field. After washing, RNA is released into an elution solution and the particles are removed.
Because isolated RNA is sensitive to RNase degradation, samples should be stored in an RNase-free environment, preferably in single-use aliquots (to prevent degradation due to repeated freezing and thawing). For short-term storage, RNase-free water (with 0.1 mM EDTA; treated with diethylpyrocarbonate) or Tris-EDTA buffer (10 mM Tris-HCl, 1mM EDTA, pH 7.0) are mostly used as storage solution. RNA is generally stable at −80° C for up to a year without degradation. For long-term storage, RNA samples may also be stored at −20°C as ethanol precipitates (in 50%, 70%, or 80% ethanol). Complementary DNA (cDNA) (see next section) is more stable and can be kept at −20°C.
39.1.3 Complementary Deoxyribonucleic Acid Synthesis
Although most molecular assays are designed for studying DNA, they can also be used for RNA. In such cases, RNA first has to undergo reverse transcription (RT). In the RT reaction, single-stranded RNA is reverse transcribed into cDNA. For the RT reaction, 1 to 2 µg of RNA (total cellular RNA or poly[A] RNA) is first incubated with a primer (either oligo[dT], random [hexamer] or gene-specific primer) at 70°C to denature the RNA secondary structure, and then quickly cooled on ice to allow the primer to anneal to the RNA. Next, deoxynucleotide triphosphates, RNase inhibitor, reverse transcriptase, and RT buffer are added to the reaction. The RT reaction is extended at 42°C for 1 hour, followed by a final heating to 70°C to inactivate the enzyme. If necessary, the template RNA can be removed by treating the RT reaction with RNase H. The cDNA resulting from the RT reaction is ready for use in molecular assays.
39.1.4 Quality Control of Isolated Deoxyribonucleic Acid and Ribonucleic Acid
It is advisable to perform three quality controls on isolated DNA and RNA. The first is to determine the quantity, the second is to check the purity, and the third is to confirm integrity.
DNA and RNA concentrations and purity are traditionally assessed using ultraviolet spectroscopy. Absorbance is measured in quartz cuvettes at 260 and 280 nm (A260 and A280). DNA and RNA have their absorption maximum at 260 nm, and their concentration is linearly related to the A260. An A260 reading of 1 is equivalent to a concentration of 50 µg/mL double-stranded DNA, 40 µg/mL single-stranded RNA, and 37 µg/mL single-stranded DNA. Because the A260 is most accurate between 0.1 and 1, it may be necessary to dilute the sample. DNA and RNA may also be quantified using fluorescence tagging methods with dyes that are specific to DNA or RNA. This improves the sensitivity, but is also more expensive and time-consuming.
The A260/A280 ratio reflects the sample purity. Pure DNA and RNA have A260/A280 ratios of 1.8 and 2, respectively. A ratio of 1.8 to 2.1 indicates sufficient purity of the sample. It is good to note that at higher pH, the A280 decreases while the A260 is unaffected, resulting in an increased ratio. Because spectroscopy does not discriminate between RNA and DNA, it may be necessary to treat RNA samples with RNase-free DNase to remove contaminating DNA.
For small samples, the NanoDrop ND-1000 UV-Vis Spectrophotometer (Thermo Fisher Scientific Inc., Waltham, MA) may be preferred. It provides a scan of the absorbance from 200 nm up to 350 nm, and therefore provides data on both the nucleotide concentration and purity. A sample as small as 1 to 2 µL is pipetted directly onto the measurement pedestal. Within 10 seconds, the absorbance scan, A260, A280, A260/A280 ratio, and nucleotide concentration is shown on screen and archived on the computer.
Because most RNA-based analyses are quantitative assays, excellent RNA integrity is critical. RNA integrity is commonly checked with the Agilent 2100 Bioanalyzer instrument (Santa Clara, CA), which uses a combination of microfluidics, capillary electrophoresis, and a fluorescent dye that binds to nucleic acids. The Agilent RNA 6000 Nano System can quantify 25 to 500 ng/µL of RNA, whereas the Agilent RNA 6000 Pico Chip System can quantify 50 to 5,000 pg/µL of RNA. Samples and a sixband RNA ladders are loaded on the Bioanalyzer Lab Chip. Size and mass information are provided by the fluorescence of RNA molecules as they move through the channels of the chip. The instrument software generates both a gel-like image and an electropherogram (Fig. 39.1), and compares the peak areas from unknown RNA samples to the peaks of the RNA ladder to determine the concentration of the unknown samples. When assessing total RNA, integrity is expressed by the RNA integrity number, with a maximum value of 10. Significant decreases in the RNA integrity number indicate degradation of total RNA.

39.2 Polymerase Chain Reaction
39.2.1 What is Polymerase Chain Reaction?
Polymerase chain reaction (PCR) was developed only in 1983. PCR is sometimes called “molecular photocopying” because it amplifies (copies) a single or a few copies of a specific short piece of DNA sequence across several orders of magnitude, generating thousands to millions of copies of that particular DNA sequence. PCR is the most common technique used in medical and biological research laboratories including trauma and orthopedic surgery. Because most molecular and genetic analyses require significant amounts of DNA, most assays are nearly impossible without PCR amplification.
39.2.2 How Does Polymerase Chain Reaction Work?
PCR is the most simple and straightforward method for measuring gene expression. It relies on repeated heating (for DNA melting) and cooling (for enzymatic replication of the DNA) (Fig. 39.2). PCR generally amplifies DNA targets that are 100 to 1,000 base pairs long. The amplified product is called an amplicon. PCR is carried out in 200 to 500 µL volume reaction tubes in a thermal cycler. For optimal temperature conduction, thin-walled reaction tubes are preferred. Most thermal cyclers have heated lids to prevent condensation at the top of the reaction tube. Alternatively, a layer of oil on top of the reaction mixture will also suffice.

The PCR amplification mixture (usually 20 to 200 µL volume) typically contains:
DNA template that contains the DNA target region to be amplified.
Two oligonucleotide primers: These are stretches of single-stranded DNA of 20 to 30 nucleotides with sequences complementary to the flanking regions of the target sequence. Primers anneal to flanking regions by hydrogen bonding (G binds C, A binds T).
Thermostable DNA polymerase (Taq polymerase; temperature optimum at around 70°C).
Deoxynucleotide triphosphates (dNTPs; nucleotides containing triphosphate groups), the building blocks from which the DNA polymerase synthesizes a new DNA strand.
Reaction buffer containing magnesium ions and other components, providing a suitable chemical environment for optimum activity and stability of the DNA polymerase.
The amplification typically consists of the following steps:
Initialization (90 to 95°C, 1 to 10 minutes): The two DNA strands carrying the target sequence separate due to breakage of the hydrogen bonds holding them together.
Denaturation (94 to 98°C, 30 to 60 seconds): This is the first regular cycling event. Heating causes melting of the double-stranded DNA template by disrupting the hydrogen bonds between complementary bases, yielding single-stranded DNA molecules.
Primer annealing (50 to 65°C, 30 to 60 seconds): Temperature lowering allows annealing of the primers to the single-stranded DNA template. These primers are complementary to either end of the target sequence but lie on opposite strands. The annealing temperature is about 3 to 5°C lower than the melting temperature of the primers used. The polymerase binds to the primer-template hybrid and begins DNA formation.
Primer extension/elongation (72°C, 30 to 60 seconds): At 72°C and in the presence of an excess of dNTPs (DNA “building blocks” A, C, G, and T), Taq DNA polymerase synthesizes two new strands of DNA, using the original strands as templates. The extension time depends on the DNA polymerase used and on the length of the DNA fragment to be amplified. At its optimum temperature, DNA polymerase will polymerize 1,000 bases per minute. Under optimum conditions, the amount of DNA target should be doubled at the end of this step.
Repetition: Steps II to IV are repeated 30 to 40 times, depending on the number of copies of the target sequence in the original DNA sample.
Final elongation (70 to 74°C, 5 to 15 minutes): This (optional) step is performed at elongation temperature to ensure that any remaining single-stranded DNA is fully extended.
Following PCR, the amplification product can be detected using agarose gel electrophoresis. The percentage of agarose in the gel is inversely related to the amplicon size. The size of PCR product is determined by comparison with a DNA ladder (i.e., a molecular weight marker with DNA fragments of known size) that is run on the same gel (Fig. 39.3). Presence of a band with the expected amplicon size indicates the presence of the target sequence in the original DNA sample. Similarly, absence of such a band may indicate that the original sample lacked the target sequence. PCR may be prone to (preventable) contamination, which may lead to false-positive or -negative results. Therefore, it is crucial to always include a positive and negative reference DNA standard when performing PCR.

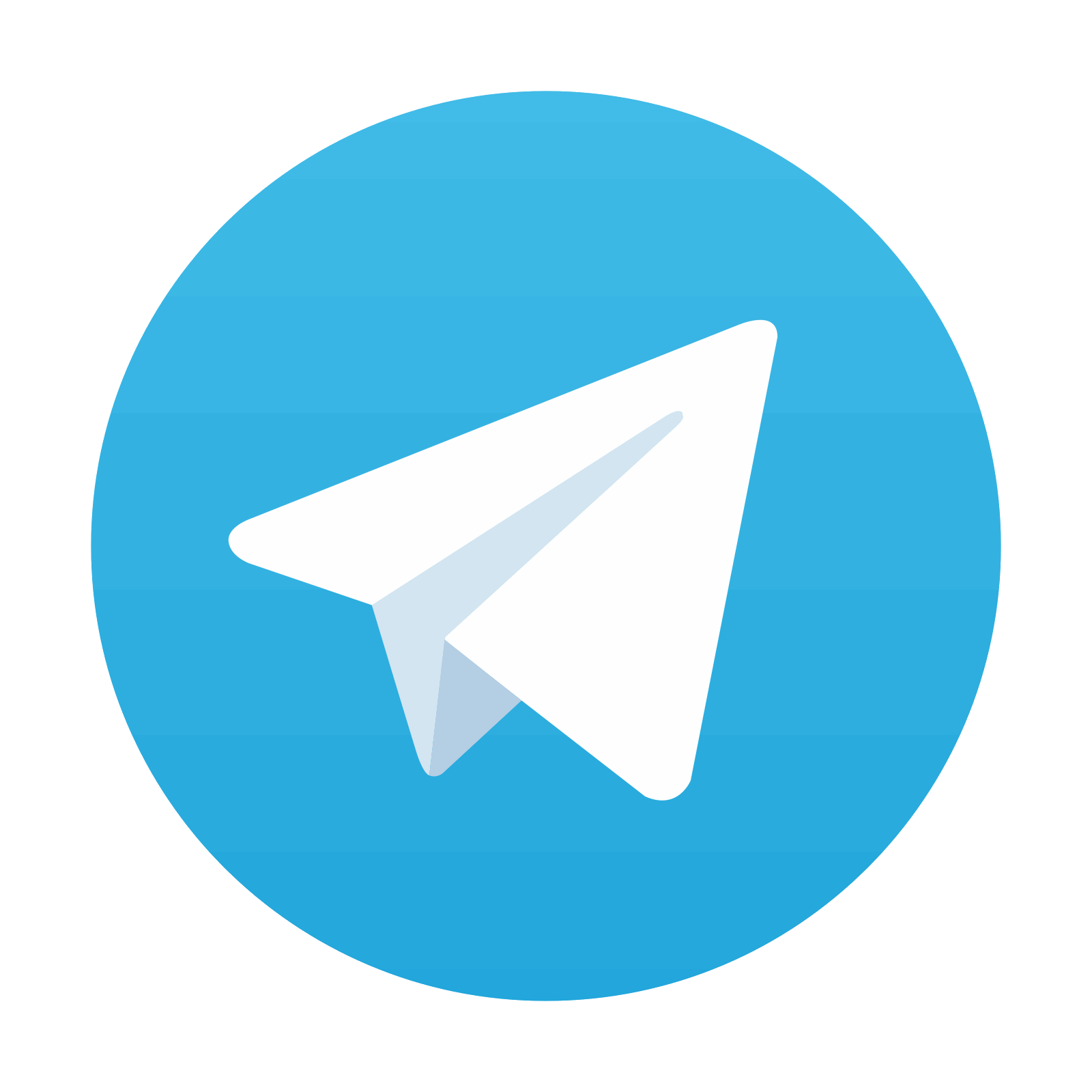
Stay updated, free articles. Join our Telegram channel

Full access? Get Clinical Tree
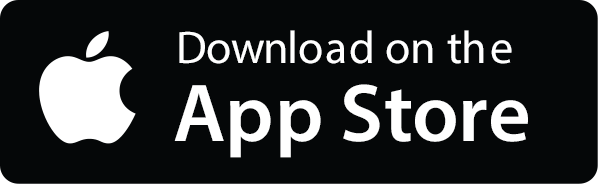
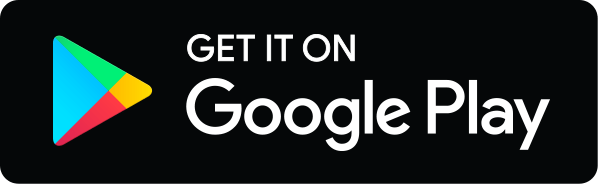
