38 Imaging and Anatomy
38.1 Introduction
Advancing technology has made medical imaging indispensable for assessing, evaluating, and diagnosing specific conditions and injuries of the wrist and upper extremity. The diagnostic value and utility of imaging techniques are improved by choosing the type of studies that are best suited for particular tissue types, anatomical regions, and conditions. This chapter will focus on relevant wrist and upper extremity anatomy and cover how plain radiography, computerized tomography (CT), magnetic resonance imaging (MRI), and ultrasound (US) aid in defining the anatomy and the conditions of the regions.
38.2 Imaging Approach to Wrist Pathology
Imaging of the hand and wrist poses special challenges. The anatomy is complex; many of the structures involved are small. There may be an overlap in radiological findings of symptomatic and asymptomatic individuals, and many of the pathological conditions have a dynamic basis, whereas imaging is typically performed in a static manner. These challenges are best exemplified in imaging of ligamentous injuries of the wrist, where the pathological lesions often involve intricate structures that measure 1 to 2 mm in thickness and may not result in significant malalignment on static images.
Despite advances in imaging techniques, plain radiographs remain an indispensable initial investigation, which often helps narrow the differential diagnosis and guide further investigation. In many cases, no further imaging is warranted following plain radiographs. At least two orthogonal views are necessary, as radiographs only provide a two-dimensional representation of three-dimensional structures, but in practice additional views are usually obtained. The advantages of radiographs include easy access, reproducibility, high spatial resolution for osseous abnormalities, relative lack of artifact from postoperative hardware, and the ability to perform stress views. In addition, plain radiographs allow for easy side-to-side comparison in equivocal cases.
CT provides exquisite osseous detail with a high spatial resolution, but contrast resolution and soft tissue detail has been lacking for imaging wrist structures. Therefore, it is most commonly used to assess occult, complex, or intra-articular fractures, the degree and pattern of joint space loss, the extent and characteristics of osseous lesions, and imaging of postoperative hardware complications. Three-dimensional images reconstructed from the axial images are often helpful by providing an overview of the abnormality, although they typically do not increase diagnostic accuracy or confidence. CT arthrography, with instillation of intra-articular iodinate contrast material, has emerged as an adjunct technique in evaluation of ligamentous and chondral injuries, taking advantage of the high spatial resolution of CT to image the small complex structure.
MRI in many ways is well suited to imaging assessment of the wrist, offering excellent soft-tissue resolution, multiplanar capabilities, and the ability to perform a global assessment of the wrist, and is extensively used in detecting osseous, tendinous, and ligamentous injuries. However, issues related to signal-to-noise ratio and spatial resolution have somewhat limited its accuracy in comparison with arthroscopy in evaluating abnormalities of intrinsic and extrinsic ligaments and articular cartilage, and as a result MR arthrography is often used to identify injuries to these structures. Three-Tesla (3T) MRI systems and advances in coil technology have significantly increased the signal-to-noise ratio, in turn allowing for thinner slice acquisitions and improved spatial resolution. Isotropic data acquisition and high-quality multiplanar reformats of the wrist are now a reality. Despite this, imaging of ligamentous injuries of the wrist is still challenging. Seven-Tesla (7T) MRI is likely to push these boundaries further and has already demonstrated improvements in imaging of the articular cartilage with demonstration of the hyaline cartilage at the interphalangeal joints. 1
Ultrasound is also widely used for assessing soft-tissue structures. Advantages of ultrasound include a high spatial resolution especially with transducers > 15 MHz, easy side-to-side comparison, assessment of vascularity within soft-tissue masses, and the synovium as well as the ability to perform dynamic assessment. As a result, ultrasound has emerged as the optimal imaging technique for diagnosing tendon injuries. 2
Advanced imaging techniques are also being used to evaluate the neurovascular structures in the upper extremity. CT angiography of the upper limb has replaced conventional angiography in many centers. MR angiography has improved substantially over the past few years with images of high temporal resolution as well as images of high spatial resolution, clearly depicting the vascular anatomy even in the digital arteries. There has been an interest in imaging of the nerves of the upper extremity using MRI, not only to detect structural changes such as swelling and edema but also to detect functional changes by using diffusion-weighted MRI.
The choice of the imaging modality used will ultimately be guided by the clinical differential diagnosis, the findings on the initial plain radiographs, and the local clinical and radiological expertise. In the majority of cases, this decision is straightforward, but in complex situations a tailored approach is often necessary.
38.3 Anatomical Considerations
The complex anatomy of the wrist consists, in general terms, of two joints, which are appreciated on routine radiographs but better appreciated on sagittal and coronal imaging of the carpus (▶Fig. 38.1). The relatively round capitate of the distal row is well contained in the socket formed by the scaphoid and lunate of the proximal row.

Flexion and extension of the wrist occurs at both the radiocarpal and the midcarpal joints (▶Fig. 38.2). But these motions, as well as radial and ulnar deviation, are oversimplified when considered in only two dimensions, as they do not address the multiple degrees of freedom that occur between and within the carpal rows. Wrist motion is dictated and restricted by the anatomical shapes of the bones and by the position, tension and integrity of the inter- and intracarpal ligaments and the joint capsule that are crucial to carpal stability. Advances in CT technology, with faster gantry rotation, smaller detectors, and extended coverage now allow for isotropic volumetric acquisitions to be performed through the whole wrist in a fraction of a second. The isotropic datasets (voxels of same dimension in all planes) allow for high-quality reformats and three-dimensional images. In addition, the high temporal and spatial resolution can be utilized to perform true dynamic assessment of wrist biomechanics. Similar experimental work is being undertaken using MRI, and these techniques should enhance our understanding of normal as well as pathological wrist biomechanics. 3 Although fluoroscopy has long been used for dynamic assessment of wrist motion, the advantage of cross-sectional techniques such as CT and MRI for kinematic assessment is the lack of superimposition of multiple bones and the ability to assess the motion of each individual bone.

The TFCC and the distal radius form a shallow socket for the proximal carpal row (▶Fig. 38.3) which is defined not only by the shapes of the radius and TFCC but also by the capsule and the extrinsic ligaments between these structures and the proximal row (see hereafter).

The proximal row is commonly referred to as the intercalated segment in reference to its interposed position between the distal carpal row and the radius/TFCC. The proximal row, consisting of the scaphoid, lunate, and triquetrum, itself forms a socket for the capitate and hamate of the distal row (▶Fig. 38.4). The structural integrity of bones or their ligaments of the proximal row is important to this relationship, and any laxity or disruption results in instability patterns and eventual arthritis. Bone anatomy of the carpus is ideally assessed by CT and three-dimensional (3D) imaging, which is useful for visualization of pathology such as fracture nonunions and osteophytes. Imaging the ligaments of the proximal row remains a challenge given the small size and structure of the ligaments themselves. Conventional MRI has a limited sensitivity for tears of the scapholunate (SL) (60%) and lunotriquetral (LT) (30%) ligaments. Even at 3T there is a modest improvement in accuracy of detecting SL and LT tears. 4 MRI arthrography results in increased sensitivity and specificity, and MRI in radial and ulnar deviation also appears to improve detail but may be limited by coil geometry. 5 CT arthrography appears to be the most accurate imaging technique for diagnosing SL (sensitivity 95%, specificity 96%) and LT (sensitivity 90%, specificity 100%) tear, but this technique lacks sensitivity for assessment of other soft-tissue structures. This technique also has the highest inter observer reliability. 6

The proximal carpal row forms a socket (▶Fig. 38.4 and ▶Fig. 38.5) for the distal row. The socket is deepened by the wrist capsule and ligaments that cross the midcarpal joint, but despite the constraints, the midcarpal joint is inherently lax. The major extrinsic ligaments on the dorsum of the wrist are the radiotriquetral (RT or radiocarpal) and the dorsal intercarpal (DIC) ligaments (▶Fig. 38.6). The RT ligament originates on the dorsum of the radius as far radial as Lister’s tubercle and inserts in the tuberosity on the dorsum of the triquetrum. This anchor point on the triquetrum is an important bony landmark and is also the origin of the DIC ligament, which crosses the wrist to insert into the dorsum of the trapezium and scaphoid. The RT and DIC ligaments lengthen in opposing directions during radial and ulnar deviation of the wrist. 7 The ligaments can be preserved during surgical approaches to the wrist. The DIC crosses the waist of the capitate and not the central portion of the midcarpal joint. The joint is contained only by the capsule in this region, allowing for motion but also leaving potential instability of the region.


The volar intercarpal ligaments (▶Fig. 38.7) are more numerous and complex than their dorsal counterparts. The radioscaphocapitate ligament originates at the radial styloid, crosses the scaphoid waist, and inserts into the volar aspect of the capitate. Between the long and short radiolunate ligaments is the intra-articular ligament of Testut (radioscapholunate ligament), which contains blood vessels to the SL region. Ulnolunate and LT ligaments form the volar aspect of the TFCC and stabilize the proximal row. The ligaments on the radial and ulnar aspect of the wrist converge and insert on the capitate to form an inverted V. As seen dorsally, there are no ligaments to cross the central portion of the midcarpal joint on the volar side. Until recently, there has been little attention paid to imaging of extrinsic ligaments in the radiology literature in comparison with assessment of intrinsic ligaments. 8 , 9 However, isotropic MRI acquisitions with slice thicknesses of 0.5 to 0.6 mm can clearly identify the extrinsic ligaments in all three planes especially when combined with MR arthrography. Ultrasound has also been used to evaluate the extrinsic carpal ligaments. 10 However, the accuracy of imaging in identifying tears of extrinsic ligaments has not been adequately assessed.

Volarly, the carpal ligaments coalesce with the wrist capsule to form the base of the carpal tunnel (▶Fig. 38.8). The carpal tunnel contains the median nerve and nine flexor tendons to the digits. The carpal tunnel can be assessed by ultrasound 11 and by MRI if soft tissue pathology is suspected. 12 The fibrous transverse carpal ligament (flexor retinaculum) comprises the roof of the tunnel that supports to structural concavity of the carpus; the ligament can be quantitated by US. 13 On its ulnar side, the transverse carpal ligament splits to form the superficial and deep walls of Guyon’s canal, containing the ulnar nerve and artery (▶Fig. 38.8b).

The two major blood vessels to the hand (the radial and ulnar arteries), and their anastomotic arches and distal vessels can be assessed by conventional arteriography as well as by CT angiography and MR angiography (▶Fig. 38.9a–d). An appreciation of the anatomy is important for assessing ischemia of the hand, replanting amputated and traumatized regions of the hand, and planning vascular bone or soft tissue transfers.

Dorsally, the extensor tendons are contained in their respective six compartments (numbered from radial to ulnar direction) by the extensor retinaculum (▶Fig. 38.10). The retinaculum is a continuation of the antebrachial fascia. It has an oblique orientation and encloses the tendons onto the distal radius for the first three compartments, or to the carpus for compartments four to six. The first compartment contains the multiple tendon slips of the abductor pollicis longus (APL) and the extensor pollicis brevis (EPB). These tendons may be separated by an intracompartmental septum; the tendons insert into the base of the metacarpal and the base of the proximal phalanx of the thumb, respectively. The intracompartmental septum is best evaluated by ultrasound. The EPB is the ulnar border of the anatomical snuffbox. The second compartment contains the tendons of the extensor carpi radialis longus (ECRL) and brevis (ECRB). The ECRL is radial to the ECRB; they insert into the second and third metacarpals, respectively. The second and third compartments are separated at Lister’s tubercle on the dorsum of the radius. The third compartment contains the extensor pollicis longus (EPL) tendon, which uses Lister’s tubercle as a fulcrum; the tendon crosses the tendons of the second compartment, defines the ulnar border of the anatomical snuffbox, and inserts in the distal phalanx of the thumb. The first three compartments have the periosteum of the distal radius as their floor. This makes the tendons vulnerable to tendonitis, rupture following radius fracture, or irritation from orthopaedic hardware. The third compartment or the interval created between the third and fourth compartments is commonly used as a surgical approach to the distal radius or to the wrist.

The extensor retinaculum is primarily at the level of the carpus at the fourth compartment. More proximally, the compartment contains the posterior interosseus artery and nerve at its floor. The compartment also contains the extensor digitorum communis (EDC) tendons, the extensor indicis proprius (EIP) tendon, and the relatively common anomalous tendons 14 , 15 to the fingers. Distally the EDC tendons are interconnected by three distinguishable types of juncturae tendinum 16 and continue into the extensor hoods on the dorsum of each of the fingers, which also receives contribution from the intrinsic muscles of the hand. 17
The fifth extensor compartment contains the extensor digiti minimi (EDM) tendon to the small finger. The compartment is directly over the distal radioulnar joint (DRUJ) (▶Fig. 38.10) and can be used as a surgical approach to the joint and to the dorsal aspect of the TFCC. The extensor retinaculum continues over the sixth compartment, containing the extensor carpi ulnaris (ECU) tendon, and finally inserts at the ulnar border of the wrist.
The proximal scaphoid, together with the lunate form a socket for the capitate, but the distal portion of the scaphoid is adjacent to the capitate to stabilize the carpal rows. Distally the scaphoid articulates with the trapezium and trapezoid (▶Fig. 38.11). Dorsally the scaphoid has a ridge that extends obliquely around the bone (▶Fig. 38.11b). The ridge is the site of the capsular attachment through which branches of the radial artery provide the vascular supply to the majority of the bone. The ridge develops osteophytes (▶Fig. 38.11c) with age and carpal collapse that may impinge on the dorsal rim of the radius.

Dorsally, the strongest portion of the SL ligament attaches to the ulnar aspect of the scaphoid ridge (▶Fig. 38.12a) to the dorsoradial edge of the lunate. The other key structural part of the SL ligament is the volar portion which receives the ligament of Testut. The SL ligament itself is C-shaped in cross-section, with the stout dorsal and volar portions at opposite sides and the membranous portion of the ligament between them. The dorsal and volar bands of the SL ligament are best visualized on MR arthrography, in either the coronal or the axial planes (▶Fig. 38.12b).

Volarly, the radioscaphocapitate ligament crosses the waist and supports the scaphoid (▶Fig. 38.13). The flexor carpi radialis (FCR) tendon passes ulnar to the scaphoid tubercle and continues within a groove on the trapezium to its insertion at the base of the second metacarpal. One corner of the transverse carpal ligament attaches in the adjacent region of the trapezium. The ligament also attaches to the scaphoid tubercle and crosses the carpal tunnel to insert into the hook of the hamate and pisiform.

On the ulnar aspect of the wrist, the distal ulna articulates with the TFCC; the TFCC forms a shallow socket for the lunate and triquetrum (▶Fig. 38.14), which in turn articulate with the distal carpal row. The TFCC is major stabilizer of the DRUJ and, together with the capsule of the joint, contains the ulnar head in the shallow sigmoid notch of the radius (▶Fig. 38.14b). The central portion of the TFCC is cartilaginous (▶Fig. 38.14c) and prone to degenerative changes with age (▶Fig. 38.14d), particularly in individuals who have positive ulnar variance. Although the various components of the TFCC, particularly the cartilage, can be easily assessed with routine MRI, the accuracy for detection of TFCC tears has been variable, with reported sensitivities and specificities of 44 to 100% and 52 to 100%, respectively. 18 , 19 The reported variation is partly dependent on the magnetic field strength, whether routine MRI or MR arthrography is used and whether the tears are through the cartilaginous disc or through the ulnar side of the TFCC. 20 In general the accuracy is higher at 3T, with MR arthrography and in evaluation of radial sided tears. In particular, the clinically significant ulnar-sided tears and particularly partial undersurface tears have been notoriously difficult to detect, even with MR arthrography with reported sensitivities as low as 17%. 21 The ligamentum subcruentum can result in high signal intensity between the foveal and ulnar styloid attachments of the TFCC, mimicking a tear. 21 The volar and dorsal radioulnar ligaments are the main stabilizers of the DRUJ, whereas the ECU sheath and the volar ulnocapitate, ulnolunate, and ulnotriquetral ligaments stabilize this aspect of the carpus. The dorsal radiotriquetral ligament, although not a part of the TFCC structure, coalesces with the ECU subsheath to insert into prominence on the dorsum of the triquetrum.

The dorsal and volar radioulnar ligaments, which are the key structural portions of the TFCC, insert in and around the fovea of the distal ulna at the base of the ulnar styloid (▶Fig. 38.15). Trauma to the ligaments or to the insertion site of the ligaments will result in DRUJ instability and is one cause for arthritis of the articular surface at the ulnar head (▶Fig. 38.15b). Standard radiography, CT, and MRI can be insensitive for detection of DRUJ instability. In clinically equivocal cases, limited CT examination through the DRUJ in pronation, neutral position, and supination has been utilized and a variety of measurements have been proposed for assessment of relationship of the ulnar head to the sigmoid notch to detect subtle subluxation. 23

The radioulnar ligaments of the TFCC have a complex structure with deep and superficial components (▶Fig. 38.16) that may not be appreciated during arthroscopy or imaging, but which can contribute to pain, instability, or clicking. The structure of the TFCC is further complicated by the volar ulnocarpal ligaments. Dorsally, the base of the ECU sheath of the sixth extensor compartment is an integral part of the TFCC (▶Fig. 38.17). Subluxation of the ECU tendon at the distal ulna requires a dynamic assessment and this is best performed by ultrasound.


Viewed from the ulnar side, the wrist has a mini anatomical snuff box bounded by the ECU and flexor carpi ulnaris (FCU) tendons (▶Fig. 38.18). The ECU continues to insert into the base of the fifth metacarpal, whereas the FCU inserts into the pisiform. The mini snuff box contains the distal ulna and ulnar styloid and the bony prominence on the ulnar aspect of the triquetrum that is enveloped by the continuation of the extensor retinaculum. The ulnar artery and nerve lie volar and slightly radial to the FCU tendon (▶Fig. 38.19), before coursing to the radial side of the pisiform at Guyon’s canal. The mini snuff box is used for injections or as an arthroscopic portal on the ulnar side of the ECU; however, the dorsal sensory branch also courses through this region (▶Fig. 38.20) 24 and is prone to injury, as are the sensory branches of the radial nerve on the radial aspect of the wrist.



The elbow joint consists of three articulations: (1) the capitellum of the humerus articulates with the radial head, (2) the trochlea of the humerus articulates with the ulna, and (3) the radial head articulates with the semilunar notch of the ulna at the proximal radioulnar joint (▶Fig. 38.21, ▶Fig. 38.22, and ▶Fig. 38.23). Stability of the elbow is achieved through articular congruity and the ligaments. The ulnar (medial) collateral ligament (Fig. 38.21) consists of an anterior, posterior, and oblique portion. The anterior and posterior aspects each originate on the humerus and insert into the coronoid process and olecranon of the ulna, respectively. The ligament is subject to laxity and injury with throwing sports. The radial (lateral) ligament originates from the humerus and inserts into the supinator crest of the ulna and into the annular ligament. The annular ligament encircles the head of the radius and maintains it within the semilunar notch. At the elbow, the brachial artery bifurcates into radial and ulnar arteries and also provides recurrent vessels around the joint (▶Fig. 38.23, ▶Fig 38.24). The interosseous arteries, on respective sides of the interosseous membrane, originate off of the ulnar artery. The shoulder is a highly mobile joint and is stabilized by the joint capsule and the rotator cuff. The musculature of the cuff initiates much of the movement of the shoulder. The limited subacromial space between the acromium and the humerus is the site of impingement particularly of the supraspinatus tendon which can progress to degenerative changes. Imaging has an important role in the management of shoulder conditions. Routine radiographs alone may be all that is required for assessing trauma and osteoarthritis of the shoulder. For soft tissue abnormalities, such as rotator cuff impingement or tears, US and MRI are particularly useful (▶Fig. 38.25, ▶Fig. 38.26, and ▶Fig. 38.27). MR or CT arthrography are used for instability, and CT alone is ideal for defining bony detail (▶Fig. 38.27c). The brachial artery is readily assessed by routine angiography or CT (▶Fig. 38.28) or by US when performing regional anesthesia.








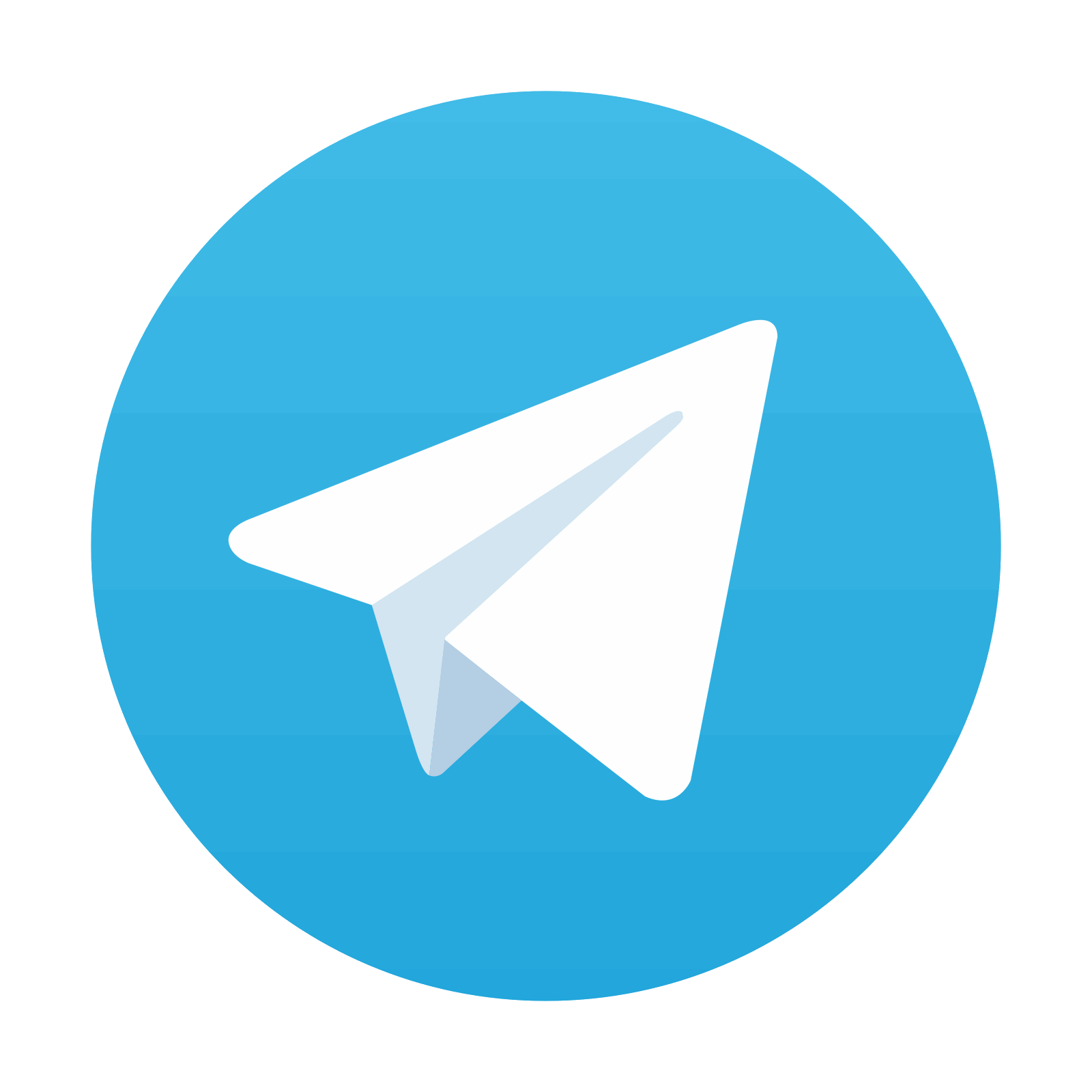
Stay updated, free articles. Join our Telegram channel

Full access? Get Clinical Tree
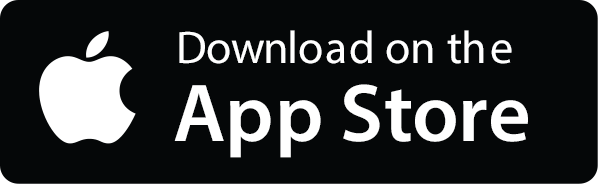
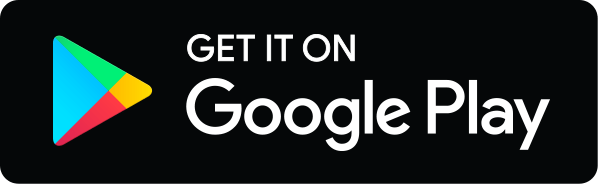