33 Laser Scanning Confocal Microscopy and Laser Microdissection
Laser scanning confocal microscopy and laser microdissection are the most indispensable cell image acquisition and isolation tools incorporating advanced laser technology into specially designed optical microscopes. In this chapter, we will outline the technical principles and present applications of these two advanced technologies in biomedical sciences. When we look at the Nobel Prizes in the past decade, cellular and molecular biology have been the topics being awarded the Nobel Prize in chemistry, physiology, or medicine almost every year. However, compared to those of nervous and circulatory systems, cellular and molecular studies of bone, cartilage, and tendon cells in the musculoskeletal system are much left behind. Cell-to-matrix interaction seems to be a much more critical issue in bone, cartilage, and tendon as these tissues are always under tensile or compressive forces. With confocal laser scanning microscopy (CLSM), the cellular activities of chondrocyte, osteoblast, or other bone cells can be monitored in situ while these cells are still interacting with their normal surrounding matrix. By applying laser micro-dissection technique, deoxyribonucleic acid (DNA), ribonucleic acid (RNA), and protein of cells from various site of skeletal tissue, such chondrocytes from resting, proliferative, and hypertrophic zones of the growth plate, can be isolated for advanced molecular analysis.
33.1 Basic Principles and Applications of Laser Scanning Confocal Microscopy
CLSM is a technique to obtain high-resolution and high-contrast optical images by using point illumination and a spatial pinhole to eliminate out-of-focus light in specimens that are thicker than the focal plane. The key feature of confocal microscopy is its ability to acquire in-focus images from selected depths, a process known as optical sectioning. Images are acquired by point-by-point scanning and reconstructed with a computer, allowing three-dimensional reconstructions of topologically complex objects. For opaque specimens, this is useful for surface profiling, whereas for nonopaque specimens, interior structures can be imaged. For interior imaging, the quality of the image is greatly improved when compared to conventional fluorescent microscopy because in-focused image information from multiple depths in the specimen is superimposed.
The principle of confocal microscopy was originally patented by Marvin Minsky in 1957, and it took more than 30 years of development for CLSM to become a standard technique. 1 In 1978, Thomas and Christoph Cremer designed a laser scanning process that scanned the three-dimensional surface of an object point-by-point by means of a focused laser beam and created the overall picture by electronic means similar to those used in scanning electron microscopes. This CLSM design combined the laser scanning method with the three-dimensional detection of biological objects labeled with fluorophore for the first time. During the next decade, confocal fluorescence microscopy was developed into a fully mature cellular and molecular imaging technology.
In CLSM, a laser beam passes through a light source aperture and is then focused by an objective lens into a focal volume within a fluorescent specimen. A mixture of emitted fluorescent light as well as reflected laser light from the illuminated spot is then recollected by the objective lens. The recollected lights then pass through a beam splitter, separating the laser light, which is reflected away from the detector, and the fluorescent light, which is passed through the splitter to the detection apparatus, from the objects. After passing through a pinhole, the fluorescent light is detected by a photo-detector, transformed into an electronic signal that is then transferred to an imaging computer system.
As seen in Fig. 33.1, the confocal pinhole obstructs the “out-of-focus” fluorescent light. Light rays from below the focal plane come to a focus before reaching the pinhole, and then they expand out so that most of the rays are physically blocked from reaching the detector by the pinhole. 2 In the same way, light from above the focal plane is focused behind the pinhole, so that most of this light also hits the edges of the pinhole and is not detected. However, all the light from the focal plane (solid red lines) is focused at the pinhole and passed on to the detector. In turn, a sharper image is obtained when compared with conventional light microscopy because all the information that is not in the focal plane is blocked. The detected light originating from an illuminated volume element within the specimen represents one pixel in the final image. As the laser scans over the plane of interest, a whole image is obtained pixel by pixel and line by line, while the brightness of a final image pixel corresponds to the relative intensity of the detected fluorescent light. The laser beam is scanned across the sample in the horizontal plane using one or more oscillating mirrors. This scanning method usually has low reaction latency, and the scan speed can be varied as slower scans provide a better signal-to-noise ratio and result in better contrast and higher resolution. Information can be collected from different focal planes by raising or lowering the microscope stage. The computer can generate a three-dimensional picture of a specimen by assembling a stack of these two-dimensional images from successive focal planes.

Jargon Simplified: Fluorophore
A fluorophore is a fluorescent chemical compound that can re-emit light upon light excitation.
33.2 Imaging Modes
33.2.1 Two-Dimensional Imaging: Single Optical Section
The simplest single optical sectioning is x-y scanning. The optical section is the basic image unit in confocal microscopy. Data can be collected from fixed and stained specimens in single-, double-, triple-, or multiple-wavelength illumination modes, and the images collected from multiple-labeled specimens will be in register with each other to produce a two-dimensional image. The thickness of the optical section is determined by the magnification, the numerical aperture of the objective used, and the diameter of the pinhole, as shown in Table 33.1. With most confocal microscopy software packages, optical sections are not restricted to the perpendicular lateral (x-y) plane, but can also be collected and displayed in transverse planes. Vertical sections in the x-z and y-z planes (parallel to the microscope optical axis) can be readily generated by most confocal software programs. Thus, the specimen appears as if it had been sectioned in a plane that is perpendicular to the lateral axis. In practice, vertical sections are obtained by combining a series of x-y scans taken along the z axis with the software, and then projecting a view of fluorescence intensity as it would appear should the microscope hardware have been capable of physically performing a vertical section.
Objective | Pinhole diameter | ||
Magnification | Numerical Aperture | (1 mm) | (7 mm) |
60 × | 1.40 | 0.4 | 1.9 |
40 × | 1.30 | 0.6 | 3.3 |
40 × | 0.55 | 1.4 | 4.3 |
25 × | 0.80 | 1.4 | 7.8 |
4 × | 0.20 | 20 | 100 |
Jargon Simplified: Optical Sectioning
Optical sectioning is the process by which a suitably designed microscope, such as confocal laser scanning microscope, can produce clear images of a focal plane deep within a thick sample without performing physical sectioning.
33.2.2 Three-Dimensional Imaging: x-y-z Series
A z-series is a sequence of optical sections collected at different levels perpendicular to the optical axis (the z-axis) within a specimen. Z-series are collected by coordinating step-by-step changes in the fine focus of the microscope with sequential image acquisition at each step. Then, a z-series can be further processed into a three-dimensional representation of the specimen using various volume visualization techniques. In terms of three-dimensional reconstructions of topologically complex objects, some models such as surface rendering are useful for surface profiling of opaque specimens, whereas other models can be used for revealing the interior on nonopaque specimens.
33.2.3 Four-Dimensional Imaging: Time Lapse and Live Cell Imaging
Living tissue preparations or other specimens exhibiting dynamic phenomena present the possibility of using CLSM to collect time-lapse sequences of three-dimensional (x, y, and z) data to be combined with time (t) as the fourth dimension. These four-dimensional datasets can be viewed using a four-dimensional viewer program. Such programs allow stereo pairs taken at each time point to be constructed and played back as a three-dimensional movie.
Imaging living tissues with CLSM is substantially more difficult than imaging fixed specimens, and it is not always a practical option because the specimen may not tolerate the conditions involved. Table 33.2 lists some of the factors to be considered when imaging live cells with CLSM compared with those on fixed cells. 3 Some specimens simply will not physically fit on the stage of the microscope, or they cannot be kept alive on the stage during observation. The phenomenon or structures of interest may not be accessible to the objective lens field of view.
Successful imaging of live cells requires extreme care to be taken throughout the imaging process to maintain tolerable conditions on the microscope stage. Photo damage from the illuminating laser beam can be cumulative over multiple scans, so the exposure to the beam should be kept to the minimum. Antioxidants such as ascorbic acid are commonly added to the culture medium to reduce oxygen, which can be released in the excitation of fluorescent molecules, causing free radicals to form and cell death. It is usually necessary to carry out extensive preliminary control experiments to assess the effects of light exposure on fluorescent-labeled cells. Following the imaging tests, the continued viability of the living specimens should be evaluated. Embryos, for example, should continue their normal development after the imaging process, and any abnormalities caused by the laser scanning should be determined.
In order to minimize the photo toxicity effect, multiphoton confocal fluorescence microscopy has been developed. This special technique allows imaging of living tissue up to a depth of 1 mm.3 Multiphoton excitation is defined as a process in which the energy for the excitation of a single molecule comes from two or more photons with lower energy and longer wavelength that are absorbed at virtually the same time. Each photon needs to contribute only part of the total energy required for the fluorophore excitation, provided that the combined amount of energy is sufficient. One advantage of this technique is that the excitation light scattering by tissue is reduced as a result of longer wavelengths, allowing excitation to penetrate more deeply into the tissue than visible light.
Specific requirements to sustain the life of cells have to be met for each individual cell type that is to be imaged. Most cell types require a stage heating device and possibly a perfusion chamber in which the proper carbon dioxide level can be maintained through the entire process of imaging.
Many physiological processes and events take place faster than they can be captured by most CLSMs, which have image acquisition rates typically on the order of one frame per second. CLSMs using acousto-optical devices and a slit for scanning are faster than the galvanometerdriven point scanning systems, and are more practical for physiological studies. This is known as a spinning disk confocal microscope. These faster designs combine good spatial resolution with good temporal resolution, which can reach up to 30 frames per second at full screen resolution, near video rate. The conventional slower single point scanning microscope systems can achieve the best temporal resolution only by scanning a much reduced area on the specimen. If full spatial resolution is required, the frames must be collected less frequently, losing some temporal resolution. On the other hand, the confocal systems using disks are capable of imaging fast physiological or other transient events.
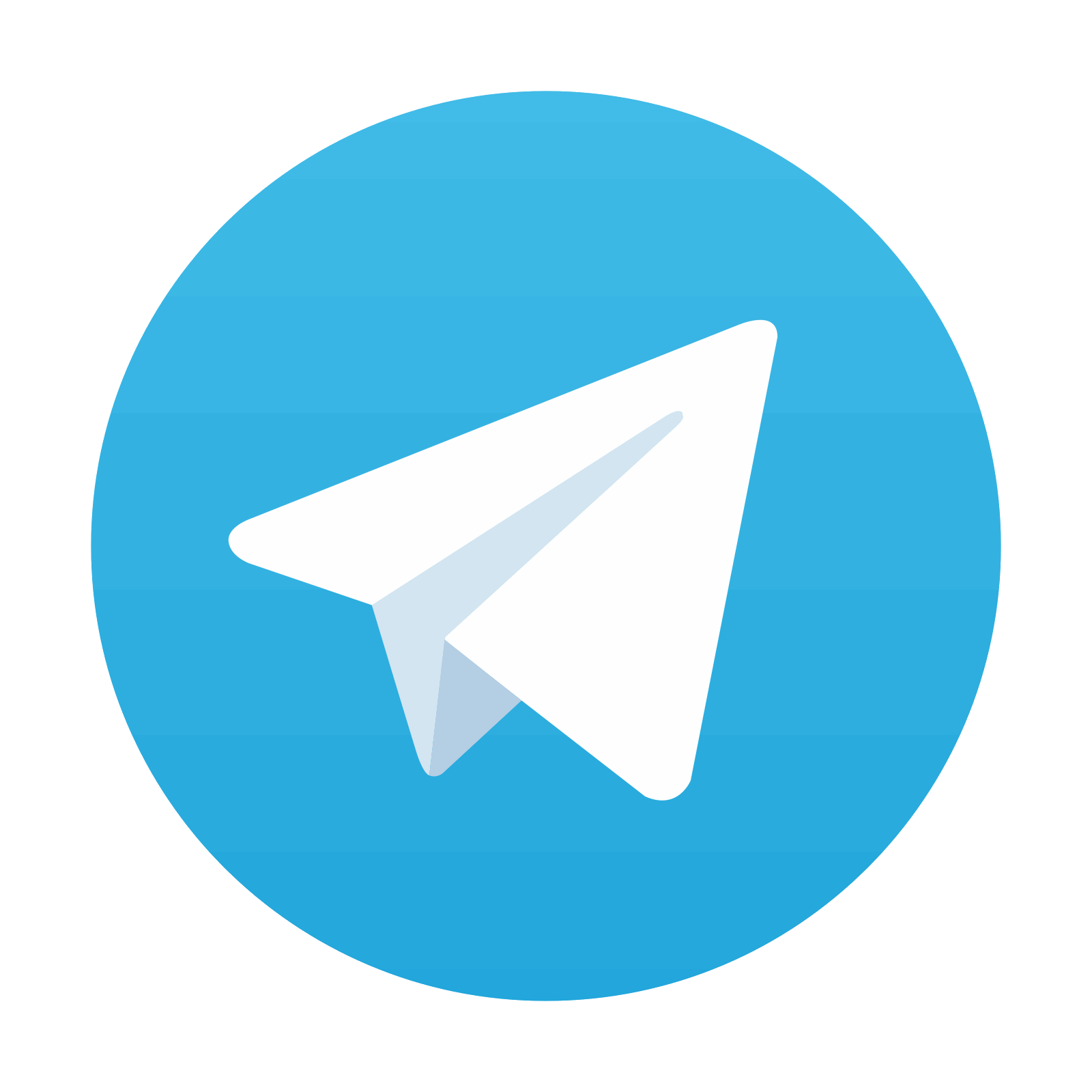
Stay updated, free articles. Join our Telegram channel

Full access? Get Clinical Tree
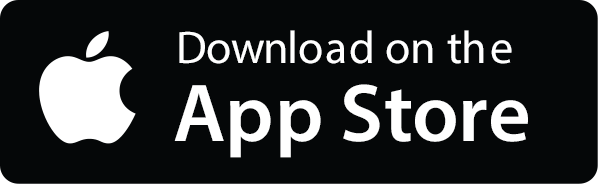
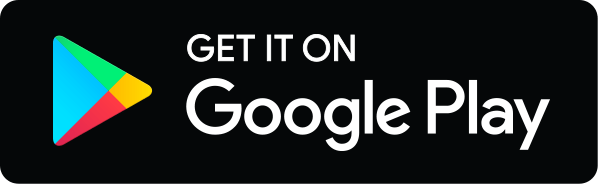
