3.2.1 Screws
To access the videos, please follow the URL link
1 Design and function
1.1 What is a screw?
A screw is a powerful mechanical device that converts rotation into linear motion.
Most screws used for fracture fixation share several design features ( Fig 3.2.1-1 ):

Central core that provides strength
Thread that engages the bone and converts rotation into linear motion
Tip that may be blunt or sharp
Head that engages either the bone or the plate
Recess to attach a screwdriver
Screws come in different shapes and sizes and their names are based upon a number of factors ( Fig 3.2.1-2 ):

Design (eg, cannulated, locking head)
Dimension (eg, 4.5 mm)
Characteristics (eg, self-tapping, self-drilling)
Area of application (cortex, cancellous, monocortical, or bicortical)
Function or mechanism
Since the introduction of locking head screws (LHS), all other types of screws are referred to as “conventional” screws. A screw can be applied to compress a fracture surface (lag screw), fix a plate to the bone by producing compression between the plate and the bone (plate screw), or it may be used to fix an external fixator (Schanz screw) or internal fixator (locking head screw) to the bone. A position screw holds two fragments together without compression. Lag screws can be inserted through a plate or independently. The term lag screw does not describe screw design but refers to the function of compressing two fragments together.
Rotation of a screw is converted in to linear motion by screw threads. As the screw advances, the head is pressed against the bone cortex and further advancement of the screw compresses the head against the cortex, creating a preload. This preload compresses the fracture and prevents separation, while friction between the fracture surfaces and between the screw and the bone opposes displacement by shear. Lag screws are used to achieve absolute stability. In contrast, LHS have a head with a thread that matches a reciprocal thread in the hole of the plate ( Fig/Animation 3.2.1-3 ). As it advances, it forms a mechanical couple with the plate that does not rely on compression between the two elements. This produces angular stability and the plate is not pressed against the bone. The load transfer of this construction occurs via the plate, not by preload and friction. This is the same principle as that of an external fixator and the stabilizing effect of LHS and Schanz screws is based on their bending stiffness plus the mechanical couple to the plate or the external fixator frame.

A screw is an efficient tool for the fixation of a fracture by interfragmentary compression or for fixing a splint, such as a plate, intramedullary nail or fixator, to the bone.
1.2 Biomechanics
The axial force produced by a screw ( Fig 3.2.1-4a ) results from rotating it clockwise, whereby the inclined surface of its thread glides along a corresponding surface of the bone. The inclination of the thread—the pitch—must be small enough to provide purchase of the screw in bone, ie, to prevent the screw from unwinding and becoming loose ( Fig 3.2.1-1 ) and the pitch must be large enough to allow full insertion with an acceptably low number of revolutions [1].

Turning the screw within bone creates friction, which in turn generates heat. Screw design and the method of insertion will have a major influence on the amount of heat generated. This heat has the potential to cause thermal necrosis of bone with subsequent loosening of the screw and must be avoided. Thermal necrosis can also be caused by blunt drill bits or by inserting large diameter (> 2 mm) wires and pins into cortical bone without appropriate predrilling. It is the surgeon′s responsibility to avoid this.
Two forces are active, one along the circumference of the screw thread (tangential), the other along the axis of the screw (axial). The first results from the torque of insertion. The second results from the displacement along the inclined surface between screw thread and bone thread, which produces axial tension. At the same time, the axial force acting upon the inclined surface produces torque, which tries to unwind the screw. This force increases with the inclination. As the friction remains constant, the range of angle of inclination that can be used is limited.
The torque applied to a conventional 4.5 mm cortex screw during tightening is divided into three components:
50% is used to overcome friction at the screw head interface
40% is transformed into axial force
10% overcomes the friction of the thread
Thus, during bench testing, a screw inserted through a plate hole can be tightened to nearly twice the torque that an isolated screw can. The reason for this is that the cortex under the head of an isolated screw is more likely to fail because of concentrating the force from the screw head to a small contact area on the bone. In cortical bone, the countersink can be used to increase the area of contact between the head of the screw and the cortex. This reduces contact stress and the risk of local microfracture beneath the screw head, especially if the screw is inclined ( Fig 3.2.1-5 ). The relation between torque applied and axial force induced is about 670 N/Nm for a conventional 4.5 mm cortex screw. In LHS, once the screw head engages within the plate hole, practically all torque is used for locking, and the torque applied to the screw thread is minimal. The screw thread is, therefore, protected, as it will only withstand functional forces without preload. This explains the observation that in a closely monitored clinical series of more than 2,000 inserted titanium LHS not one failure occurred [1]. However, if a surgeon applies uncontrolled torque during tightening, something will have to give way. This could be the connection to the drive where, at higher torque, the hexagonal recess may strip, especially if a worn-out screwdriver is used.

It is important that the surgeon checks the quality of the screwdriver tip regularly before use.
The compression applied by a screw affects a comparatively small area of the surrounding bone. Therefore, a single screw compressing an oblique fracture does not effectively counteract rotation of the bone fragments around the axis of that screw. The area of the compression induced around the screw is small. This is of importance with respect to torque acting upon smooth osteotomy surfaces: a single screw applied to a surface does not provide much resistance against torque between the two fragments. Such situations require a second screw placed well apart from the first one and, if possible, in a different direction. The leverage then corresponds to the distance between the screws plus twice the leverage of the single screw ( Fig 3.2.1-6 ).

1.3 Screw thread
There are three types of screw threads used for the AO technique:
The cortex screw thread is designed for application in diaphyseal bone and is available in different sizes.
The cancellous bone screws have a deeper thread, a larger pitch, and a larger outer diameter than the cortex screws. Their applications are in metaphyseal or epiphyseal cancellous bone.
The LHS used with the locking compression plate (LCP) ( Fig 3.2.1-4b ) are characterized by a larger core diameter and a comparatively shallow thread with blunt edges. These result in increased strength and a larger interface between screw and bone compared with conventional screws [2]. The head of the screw is also threaded. The shallow profile of the thread demands precise insertion technique with the power drive to prevent toggling that will result in a reduction of purchase at the screw thread – bone interface [3]. The advantages and hazards of the LHS are described in detail in chapters 3.3.2 and 3.3.4.
When inserting LHS, the last revolutions must always be done by hand using a torque-limiting screwdriver.
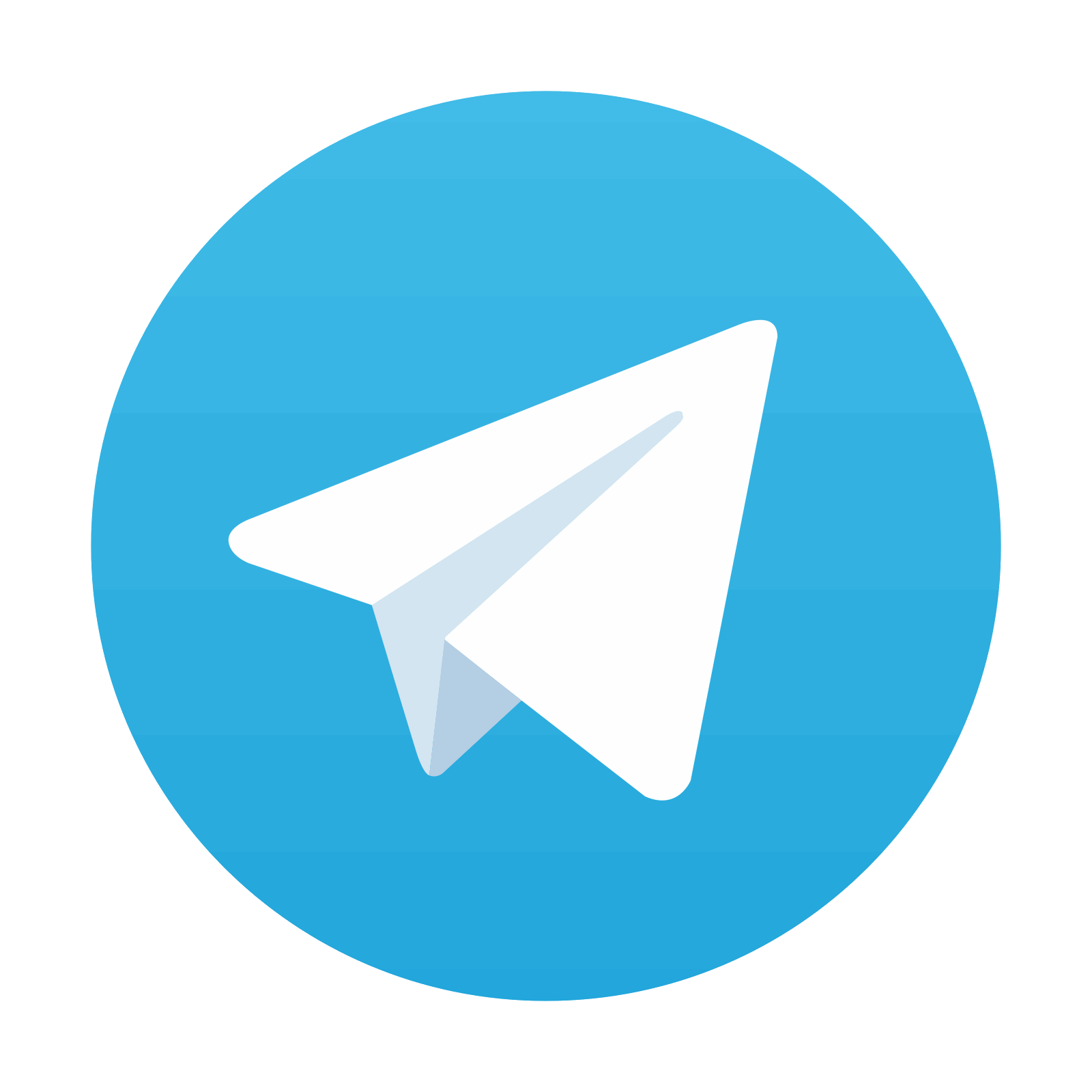
Stay updated, free articles. Join our Telegram channel

Full access? Get Clinical Tree
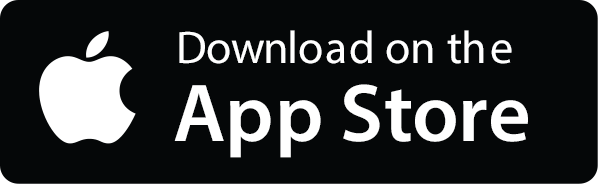
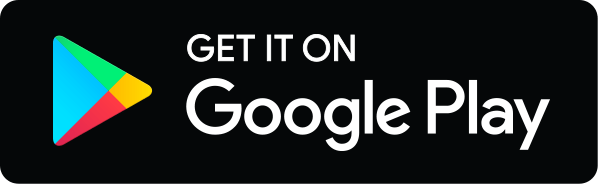
