27 Ultrasound Techniques for Imaging Bone
Fracture recovery is currently assessed using a combination of physical assessment and X-ray. 1 The limitations of this approach are a long-held concern by clinicians because both methods are subject to interobserver variability. 2, 3 In addition, signs of fracture healing are not normally visible on radiographs until after 6 to 8 weeks, when the forming fracture callus has become sufficiently dense with calcium to be visible. 4 Earlier signs of the healing process cannot be detected by X-ray, which means if complications occur in the healing process, these may go undetected for weeks or months. This prolongs rehabilitation times, causes further distress to the patient, and is ultimately costly. Furthermore, the amount of callus observed using this traditional imaging modality does not correlate well with the true state of healing or the clinical assessment of bony union. 5 As a final point, X-ray yields a two-dimensional (2D) projection image and so three-dimensional (3D) information regarding surface, volume, and depth is inherently difficult to perceive.
27.1 Overview of Three-Dimensional Freehand Ultrasound
Ultrasound as an imaging modality for monitoring fracture healing and repair can reveal details about the initial stages of healing from 1 to 2 weeks postfracture and enable earlier detection of complications. 6 Soft tissue and bone are imaged simultaneously, which allows surrounding tissue damage to be examined along with the hematoma that forms at the first stage of fracture repair. 7
3D ultrasound is yet more advantageous than conventional 2D ultrasound because it can provide effective 3D views of the anatomy that enhance interpretation and aid diagnosis. This is of particular relevance to monitoring fracture healing and bone repair where 3D details of callus formation are of considerable interest. The last 15 years have seen the emergence of 3D freehand ultra-sound as a viable technique for medical imaging, 8, 9, 10 though its potential for monitoring fracture healing remains largely undiscovered. 11
A six-degree-of-freedom position sensing device is easily attached to the transducer of a conventional diagnostic ultrasound machine.8 As the probe is moved over the object of interest, its position and orientation in space are then recorded along with the 2D ultrasound images (B-scans) to form an irregularly sampled 3D dataset describing the scan volume. Slices through this scan volume can be extracted, yielding views that would be inaccessible with conventional 2D ultrasound.
From these irregularly distributed B-scans and their positions, a regular 3D lattice volume can be reconstructed, similar to computed tomography (CT) and magnetic resonance (MR) imaging. This allows the application of 3D image postprocessing algorithms such as object segmentation, surface rendering, and volumetric registration. Further quantitative analysis of object parameters such as length, area, perimeter, and volume is also possible.
27.1.1 Position Sensing
Two types of tracking system are commonly used: electromagnetic and optical. Electromagnetic tracking comprises a transmitter that generates a magnetic field and a sensor that is attached to the probe. 12 There are two technologies for electromagnetic tracking; direct current systems use pulsed direct current to build up electromagnetic fields, whereas alternating current systems are based on alternating magnetic fields. Electromagnetic position sensors can achieve an accuracy of up to ± 0.5 mm in location and ± 0.7 degrees in orientation for the position sensor alone. However, direct current systems are sensitive to the close proximity of ferromagnetic materials, whereas alternating current systems are affected by metal because of the induced current within. In addition, attaching a position sensor to an ultrasound probe will also introduce interference and increase susceptibility to error. 13
As an alternative, optical tracking is not only immune to distortions caused by ferromagnetic or metal objects and interference, it has a greater accuracy and precision than electromagnetic tracking devices (± 0.2 mm for the position sensor alone).9 Optical tracking consists of an infrared position sensor mounted on a tripod and a tracking tool attached to the ultrasound probe. The position sensor detects infrared light emitted from several light-emitting diode markers on the tracking tool and calculates the position and orientation based on the information received from those markers, though only when there is a clear line-of-sight between the tracking tool and the position sensor.
Regardless of the tracking implemented, freehand scanning works by combining 2D B-scan images from the ultrasound machine with transducer positional information from the tracking system using dedicated software (STRADWIN, Cambridge University, Cambridge, UK) on an additional computer to compute 3D ultrasound images in near real-time.
27.1.2 Image Data Transfer
Image data transfer takes place by recording radiofrequency ultrasound signals live from the ultrasound scanner. For example, using a Gage Compuscope 14200 14-bit digitizer (Cambridgeshire, UK), the analogue radiofrequency signals can be digitized after receive-focusing and time-gain compensation but before log-compression and envelope detection. Whole frames are then stored in on-board Gage memory before transferring to computer memory at 200 MB/s. Such an arrangement can operate in real-time with acquisition rates of approximately 30 frames per second and sampling at 66.67 MHz synchronous with the ultrasound machine′s internal clock. The STRADWIN software can convert the radiofrequency data to a displayable B-scan format.
27.1.3 Calibration
A 3D freehand system must be calibrated prior to use, and this is a two-step process.10 Firstly, the delay between acquired B-mode scans from the ultrasound machine and incoming positions from the optical tracking system must be calculated. Secondly, the coordinate transformation from the ultrasound scan plane to the infrared camera of the tracking system should be determined. After calibration, pixel coordinates in any recorded B-scan image are transformed into 3D space with typical error of less than ± 0.25 mm.
27.1.4 Three-Dimensional Image Interpretation
A single 3D freehand ultrasound scan can contain anywhere between 200 and 500 2D images. Views of a fracture not achievable with conventional 2D ultrasound can be obtained by “re-slicing” the 3D dataset (in a manner similar to MR or CT imaging) and examining for signs of healing such as hematoma and callus formation. 3D computer models of the fracture site are constructed by manually segmenting different soft tissues, callus deposits, bone fragments, and sections of healthy bone. Information on the progression of fracture healing from 3D ultra-sound is attained by repeating the process temporally.
27.2 Imaging Fracture and Bone Repair
3D freehand scanning retains all the flexibility and freedom of movement associated with conventional ultra-sound scanning but with the added ability to reconstruct 3D datasets of the volume of interest. The modality is well suited to monitoring fracture healing and bone repair, particularly in closed fracture to one of the long bones of the lower limb and in limb lengthening. Patients treated conservatively using a plaster cast have to be excluded as this would entail a “window” being cut in the cast to allow ultrasound scanning.
27.2.1 Three-Dimensional Freehand Scanning
3D freehand ultrasound scanning is, in our experience,11 tolerated well by patients despite some initial reservations that the contact pressure exerted by the probe on the skin surface over the fracture site might cause discomfort. It takes on average 15 minutes to position a patient and complete freehand ultrasound scanning. Time required to interpret 3D ultrasound image data is largely dependent on the ease of identification of different soft tissues, callus, and bone, and typically requires about 60 minutes to complete per scan.
Time spent segmenting scans to create 3D computer models is a restricting factor of 3D freehand ultrasound. However, recent developments in computational postprocessing techniques, such as the automatic detection of bone surfaces, 14 speed up analysis by allowing the operator to concentrate on segmenting callus material and soft tissue damage. This in turn increases the viability of 3D freehand ultrasound as a clinically useful tool.
One of the key advantages of freehand ultrasound is that the position-sensing equipment can be transferred between scanners and probes, and so existing ultrasound machines could easily be upgraded to 3D and utilized more comprehensively for musculoskeletal examinations. In addition, multiple freehand sweeps, stitched together in postprocessing, allow the imaging of complex fracture sites. 3D freehand ultrasound is a flexible and portable imaging tool that can be brought to the bedside, clinic, or theater, and is well suited to scanning elderly people as well as frail patients and children. It is also important to note that there are no restrictions for people with pacemakers or metal implants/fixators, and frequent repeat scanning is possible as there is no dose of ionizing radiation.
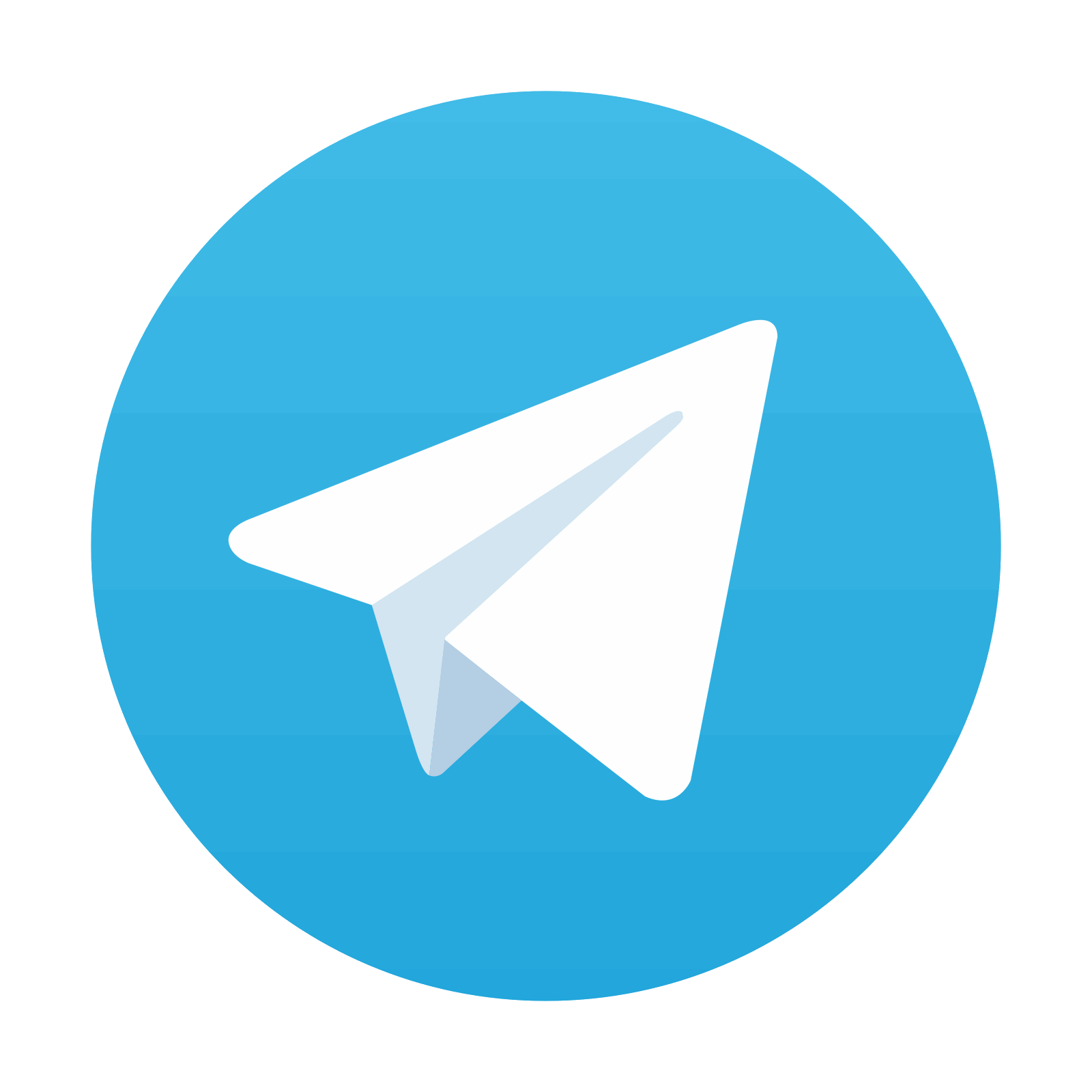
Stay updated, free articles. Join our Telegram channel

Full access? Get Clinical Tree
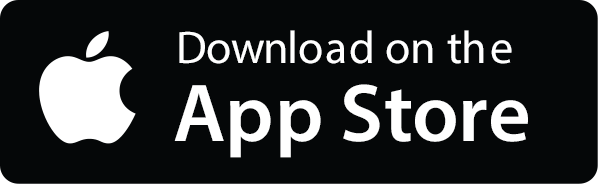
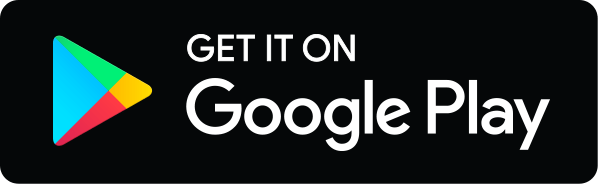
