23 Function of the Wrist Joint
23.1 Introduction
The hand gives meaning to the upper extremity. Without a hand, the upper limb is like a crane without a hook at the end of the cable. For the hand to be functional, however, the proximal articulations must be both mobile and stable. 1 If the upper limb has poor mobility, the hand cannot be placed where it is required. If the proximal articulations lack stability, the hand will give way when attempting to grip, pinch, or lift an object. Thus to understand upper limb function, one must investigate both how the upper limb joints move (kinematics) and how they sustain load without yielding (kinetics). 2 , 3
The wrist has often been regarded as the least important articulation of the upper extremity—a joint that can be eliminated without creating much functional impairment. Certainly, the implications of having a stiff wrist are not as substantial as having the shoulder or the elbow arthrodesed. This, however, should not be taken to the extreme of considering the wrist as a disposable joint. When specifically asked for, patients with a fused wrist admit serious difficulties with many activities of daily living, such as turning a door knob, washing one’s back, dusting lower surfaces, rotating the steering wheel, or beating an egg. 4 , 5 The wrist provides precision, delicacy, and effectiveness to most hand actions. The wrist allows adjusting the position of the hand in ways that are not conceivable without the carpus. Furthermore, without a wrist capable of throwing stones with accuracy, the early chimpanzees would have not been able to defend themselves from faster predators, and probably human evolution would have not been the same. 6 Indeed, the wrist is not an irrelevant articulation that can be eliminated without paying a functional penalty.
The wrist is a complex composite joint. To explain its function, several mechanical models have been suggested: the wrist as two interconnected rows (proximal and distal), as a system of three interdependent columns (lateral, central, and medial), or as a ring of four linked units (distal row, scaphoid, lunate, and triquetrum). 3 Unsurprisingly, none of these models has been able to explain all the intricate mechanisms of the joint, which, according to many authors, is one of the most complex in the human body. 3 , 7 , 8
Aside from placing the hand in the best possible position to manipulate objects, the wrist is also responsible of controlling the moment arms of most extrinsic tendons of the hand. 9 , 10 , 11 This is achieved by means of pulleys. Palmarly, the carpal tunnel, acting as a pulley, keeps the flexor tendons as close as possible to the center of rotation of the joint in order to minimize their moment arms. Dorsally, six osteofibrous compartments prevent the extensor tendons from subluxing medially or laterally during wrist flexion, or from displacing dorsally during wrist extension. Controlling proper positioning of these tendons relative to the radius is another important task assigned to the wrist that cannot be underestimated.
For the wrist joint to function properly, it must have: (1) normally shaped joint surfaces with adequate orientation to guide carpal bone motion (▶Fig. 23.1a–d), (2) a system of ligaments providing primary mechanical stability (▶Fig. 23.2a, b), (3) a network of intracapsular mechanoreceptors supplying unconscious proprioceptive information from inside the joint, and (4) finely tuned reflexes ensuring proper neuromuscular control of carpal alignment. 7 , 8 To discuss these issues, this chapter has been divided into two sections. The first section discusses carpal bone motion (kinematics), and the second analyzes force transmission across the carpus, wrist proprioception, and the mechanisms of wrist stabilization (kinetics).


23.2 Wrist Kinematics
As already stated, to manipulate an object, the hand needs to be placed in the best possible position to reach that object; this is mainly achieved by the shoulder, the elbow, and the forearm articulations. Once close to the object, the wrist is in charge of making the final adjustments in hand positioning to maximize finger efficiency. If the wrist is stiff, the hand cannot be adjusted as per the requirement for a precise and effective hand performance. Wrist mobility, therefore, is important to achieve adequate hand function.
Most descriptions of the kinematic behavior of the carpal bones have been based on observations made on cadaver or on in vivo determinations using stepwise, statically acquired CT scans of wrists from normal individuals. 12 – 19 Despite all efforts spent in these regards, we still have incomplete understanding of carpal kinematics. There are two main reasons explaining this: first, because there is not one but rather a spectrum of different, yet all normal, carpal behaviors; 12 , 15 , 16 second, because the current techniques for measuring individual carpal motion are too cumbersome and time-consuming to allow collection of data from a large number of wrists. Indeed, we lack information about the incidence and relevance of the different patterns of carpal kinematics. 14 Fortunately, a new method to assess joint motion has been recently introduced. It is the so-called four-dimensional dynamic tomography (▶Fig. 23.3). 20 , 21 This novel technology permits obtaining high-quality three-dimensional (3D) images of moving bones, in just a few minutes, with acceptable levels of radiation. Doubtless, in the near future, it will be easy for the clinician to measure individual carpal bone motion with high precision and little cost. This will allow more accurate assessment of problems (carpal dyskinematics) for which we still have little to offer.

Wrist motion may occur as a result of an external force inducing passive rotation of the carpal bones, or it may be the consequence of active contraction of the muscles crossing the articulation. This chapter will mostly concentrate on the latter. When the wrist moves, there is an interaction and accumulation of motions at different levels of the joint. Global wrist motion cannot be understood without a detailed analysis of the kinematic behavior of all its elements (▶Fig. 23.4a, b). Individual carpal bone motion is usually described as a combination of rotations along three orthogonal planes (sagittal, frontal, and transverse), taking the distal radius as a reference (▶Table 23.1). 13 , 15 , 19 Rotation along the sagittal plane determines flexion–extension (yaw angle), the frontal plane determines radial–ulnar deviation (pitch angle), and the transverse plane determines pronation–supination (roll angle). Until recently, it was believed that only the first and second types of rotation (flexion–extension and radial–ulnar deviation) could be actively produced by the tendons crossing the wrist. It was thought that intracarpal pronosupination was a passive rotation, possible only if the wrist was not loaded. Now it is known that not only the forearm muscles may actively rotate the distal row up to an average 19° pronation and 23° supination but also that such a rotation is the key to understanding the neuromuscular stabilization of the carpus. 22 , 23

Because the wrist is not a single-axis joint with collateral ligaments guiding a unidirectional arc of motion, the unconstrained wrist seldom rotates in a pure flexion–extension or radial–ulnar deviation mode. In fact, most activities of daily living (using a hammer, fishing, bouncing a ball, or lifting heavy objects) involve an oblique type of wrist motion, from extension–radial deviation to flexion–ulnar deviation; it is the so-called dart-throwing plane of motion (▶Fig. 23.5). 17 , 19 , 24 What follows is a description of the behavior of the carpal bones when the wrist moves along the more commonly used planes of motion.

23.2.1 Flexion–Extension
Flexion of the wrist is defined as a rotation that approximates the palm to the anterior aspect of the forearm. The transverse axis along which this rotation occurs is located about the proximal part of the head of the capitate near the lunate and is parallel to the palmar aspect of the distal radial metaphysis. Extension of the wrist is also a rotation about this axis but in the opposite direction: the dorsum of the hand approximates the dorsal aspect of the forearm. Any tendon crossing the wrist palmar to this axis is likely to induce wrist flexion, while extension may result from the action of any tendon dorsal to this axis. 25 This includes all wrist motor tendons (flexor carpi radialis [FCR], flexor carpi ulnaris [FCU], palmaris longus [PL], extensor carpi radialis brevis and longus [ECRB-L], and extensor carpi ulnaris [ECU]) and all finger and thumb extrinsic tendons. The efficacy of these muscles in producing flexion or extension of the wrist is directly proportional to the distance between the tendon and the axis of rotation (moment arm). The average maximal active flexion and extension in normal wrists is 59 and 79°, respectively. 26 This range varies substantially from one individual to another, being wider among hyperlax individuals. 27
The distal row is a very rigid structure formed by four bones (trapezium, trapezoid, capitate, and hamate) solidly interconnected to each other by strong and stout interosseous ligaments (▶Fig. 23.6). 28 During wrist flexion–extension, little intercarpal motion exists between the four bones; they all move synergistically, in the same direction, as if they were one functional unit. 13 – 15

The proximal carpal row has no tendon insertions. All wrist motor tendons are inserted onto the distal row or at the base of the metacarpals. The pisiform has a tendon insertion, but it is not a true proximal row bone but rather a sesamoid to enhance the mechanical advantage of the FCU. Consequently, when one of these muscles contracts only the distal row starts moving. The proximal row does not rotate until a certain level of tension develops in the midcarpal crossing ligaments; this tension generates eccentric compressive forces in the midcarpal joint, forcing the proximal bones to move (▶Fig. 23.7). Indeed, it takes some time between the contraction of muscles and the movement of proximal row. During that period, there is only motion at the midcarpal joint. In other words, most actions around the neutral position are done without moving the radiocarpal joint.

Unlike the distal row, the bones of the proximal carpal row are less strongly bound to one another. Marked differences in the magnitude of rotation exist between the scaphoid, lunate, and triquetrum (▶Fig. 23.8a–c). As found by Kobayashi et al, 13 for a total 120° of wrist flexion–extension, the scaphoid rotates an average of 92°, while the lunate rotates 53°, and the triquetrum 69° (▶Table 23.1). Such differences in bone rotation are the consequence of the different radii of curvatures of their proximal poles: with smaller radii of curvature, the scaphoid needs to rotate more than the lunate to get to the wrist maximal range.

It is commonly believed that the axis of rotation of the scaphoid relative to the lunate is located at the center of curvature of the two bones. This is not so. As demonstrated in different studies, 13 , 19 there is an instantaneous axis of scapholunate (SL) rotation, slightly oblique, located very close to the dorsal SL ligament (▶Fig. 23.8c). In fact, scaphoid and lunate rotate not as two wheels sharing a central axis but rather about a dorsal eccentric axis, implying a “scissor like” type of rotation. If the dorsal SL ligament is so stout and strong, it is because, as a hinge, it must resist important torsions. In contrast, the palmar SL ligament is elastic, long, and oblique in order to allow larger rotation to the scaphoid (▶Fig. 23.9).

The axis of flexion–extension of the lunotriquetral (LTq) joint appears to be more centrally located. On average the triquetrum rotates 16° more than the lunate, but this is small compared with the 39° average rotation exhibited by the SL joint as the wrist moves from maximal flexion to maximal extension. 13
The contribution of the radiocarpal and midcarpal joints to the overall wrist flexion–extension varies substantially from one column to another. In the central column, about 31% of the overall flexion and 66% of extension occurs at the proximal radiolunate joint, while the rest occurs at the lunocapitate interval. 14 In the lateral column, the radioscaphoid joint contributes 62% of the overall flexion and 86% of the extension, while the rest occurs at the scapho–trapezial–trapezoidal (STT) joint. 14
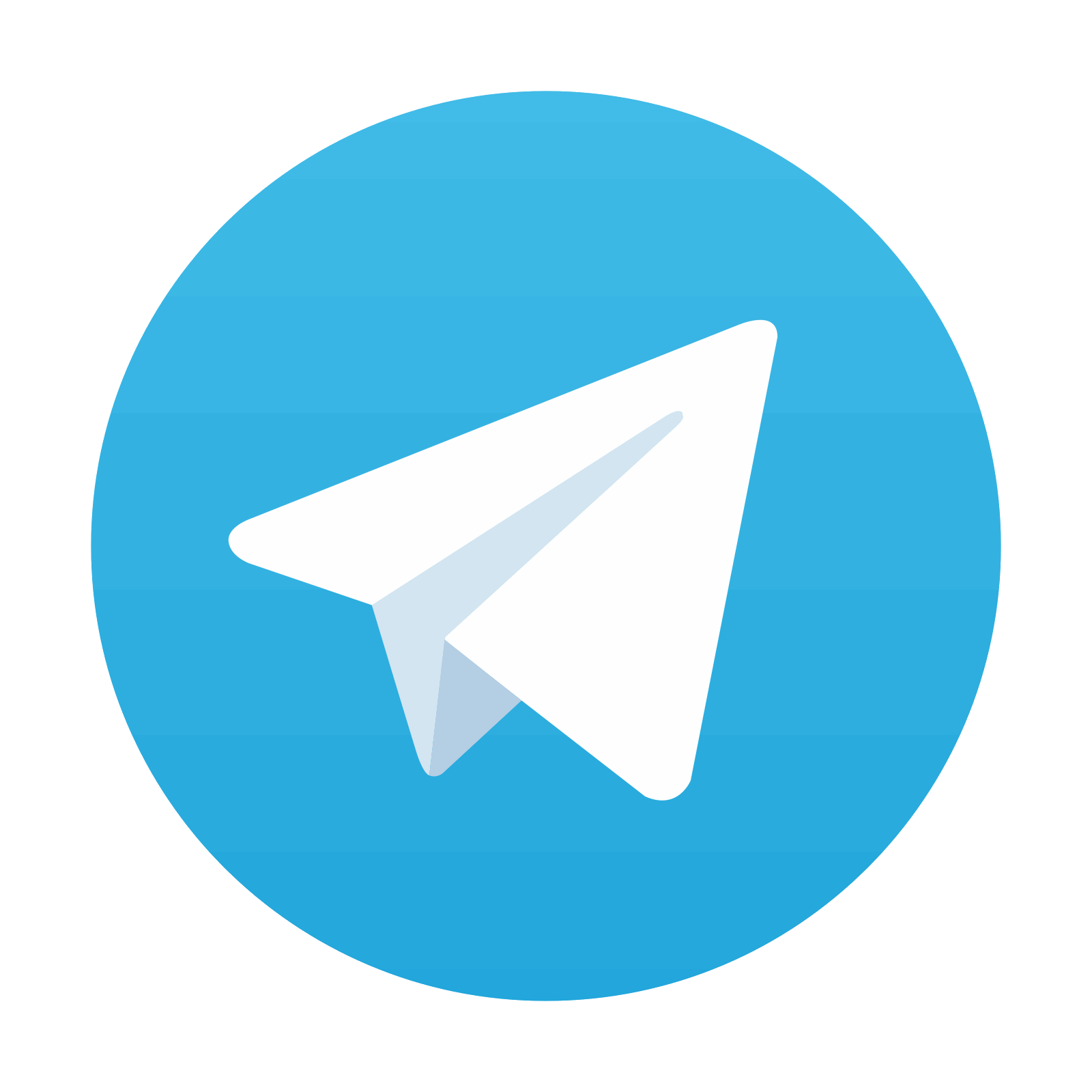
Stay updated, free articles. Join our Telegram channel

Full access? Get Clinical Tree
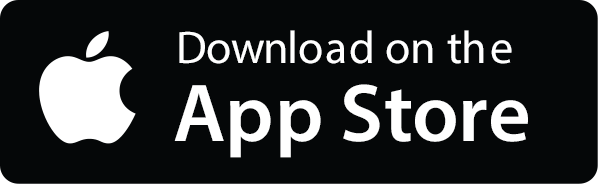
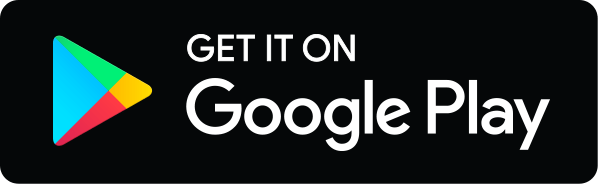
