2 Establishing a Basic Research Facility in Orthopedic Surgery
The initiation of research programs requires complex decision-making as directional, logistical, and financial considerations must be evaluated. The greatest barriers to the development of new basic research facilities include available technical expertise, space, and finances. The establishment of an orthopedic laboratory should be based on research interest, expertise, funding, and the surrounding research environment. Collaboration between multiple disciplines and centers is key to the success of a research program. Here, we outline some of the major research directions in orthopedics and list the basic equipment to set up a laboratory for cellular and molecular biology, biomechanics, and tissue engineering of musculoskeletal tissues.
Because our population is aging and the rate of automobile accidents worldwide is increasing, musculoskeletal problems continue to represent a significant source of death and disability, with growing societal and economic burdens. Although our knowledge about musculoskeletal diseases has been greatly improved in the last decades, we are still facing challenges and have limited options to treat fracture nonunions, large segmental bone/joint defects, degenerative joint diseases, failed implants, and ligament injuries. Research into the normal biology of musculoskeletal tissues, the diseases and injuries associated with these tissues, and the underlying mechanisms of musculoskeletal tissue regeneration continue to gain importance. These investigations often require a multidisciplinary approach including basic cellular and molecular biology, bioengineering, biomechanics, and clinical research. Collaboration between disciplines and centers with different expertise is essential to continue to advance the field. A team of self-motivated scientists and experienced technicians is key to the success of a laboratory. The purpose of this chapter is to address issues that may be of interest to the development of new basic science research programs and initiatives. A brief review of the current and developing areas of orthopedic research is included, and the resources required for establishing a new biology, tissue engineering, or biomechanics research laboratory are described.
2.1 Development of a Basic Biology Laboratory
2.1.1 Research Directions for a Biology Laboratory
The goal of orthopedic research is to develop better treatments to prevent, cure, or slow down the progress of musculoskeletal disorders. Research into basic cellular and molecular mechanisms of skeletogenesis, skeletal tissue regeneration, and musculoskeletal disorders is critical to the accomplishment of this goal.
Embryonic Skeletal Development
The human skeleton forms through two distinct processes: endochondral ossification and intramembranous ossification. During endochondral ossification, progenitor cells differentiate into chondrocytes and form cartilage first, which is then replaced by bone. All the long bones in our body (except for the clavicle) develop through this process. Intramembranous ossification occurs through direct bone formation; examples are the flat craniofacial bones. Bone development is a highly regulated process that involves multiple transcription factors, such as SOX-9 and cbfa-1, and their downstream molecules. Since bone regeneration in adults largely recapitulates the process that occurs during embryonic skeletal development and shares similar regulating mechanisms, the findings in skeletogenesis frequently lead to novel therapeutic targets for bone repair. For example, molecules such as matrix metalloproteinase-9, matrix extracellular phosphoglycoprotein, and wnt proteins are expressed during both skeletogenesis and adult bone repair. Further research demonstrated that matrix extracellular phosphoglycoprotein and wnt proteins could promote osteogenesis in vitro or in vivo.
Bone Regeneration
Bone repair is initiated by an acute inflammatory response, which is followed by the recruitment of skeletal progenitor cells, differentiation of chondrocytes and osteoblasts, formation of bone and cartilage, and remodeling of the callus. Inflammation plays an important role in fracture healing by modulating angiogenesis, stem cell recruitment, and callus remodeling. Numerous cytokines, growth factors, and molecules are involved in fracture healing. Expression of tumor necrosis factor-α, interleukin (IL)-1, IL-6, IL-10, IL-18, vascular endothelial growth factor, and bone morphogenetic proteins (BMPs) are detected in fracture calluses. 1, 2, 3 The contribution of these molecules, cytokines, and inflammatory cells to fracture healing is a focus of current orthopedic research. Other important research topics of bone regeneration are determining the sources of repairing cells, improving bone regeneration in patients with diabetic mellitus or peripheral vascular diseases, and treating large bone defects.
Cartilage Repair
Although we have been studying cartilage repairs for decades, it still remains a challenge to regenerate cartilage with the morphology, chemical compositions, biomechanical properties, and long-term functions comparable to native cartilage. Articular cartilage has a poor capacity to repair itself after injury and tends to heal through the formation of fibrocartilage, which has inferior biomechanical characteristics to resist compression stress compared to normal articular hyaline cartilage. Further understanding of the differences between hyaline cartilage and fibrocartilage, as well as the molecular mechanisms that govern chondrocyte differentiation and extracellular matrix production, could provide clues on how to direct cells to produce hyaline cartilage instead of fibrocartilage. In this field, growth factors such as transforming growth factor (TGF)-β, insulinlike growth factor-1, fibroblast growth factor-2, and BMP-7 may be exploited to improve cartilage regeneration.
Fibrocartilaginous tissues, such as meniscus and intervertebral disk, are equally challenging to regenerate because of the lack of adequate blood supply and the high demand for mechanical loading. The intervertebral disk is composed of three tissues: the cartilage endplate, nucleus pulposus, and annulus fibrosus. These three types of tissue create architecture with a greater level of complexity than that of articular cartilage. Successful regeneration of intervertebral disk may require the regeneration of all three tissues in one implant. Tissue engineering will play an important role in cartilage regeneration, and the right scaffolds, cells, and growth factors need to be tested.
Soft Tissue Regeneration
Muscles, tendons, and ligaments along with blood vessels and nerves are closely associated with bone. The basic biology of muscle and muscle repair is relatively well understood compared to other soft tissues. However, further advances are needed to treat devastating diseases such as Duchenne muscular dystrophy and to improve muscle repair. The biology of tendons and ligaments is now being better understood with the identification of key molecular pathways and cells involved in these tissues. Like muscle and bone healing, tendon and ligament healing is initiated by an inflammatory response that may be modulated to stimulate repair. The key issue with current tendon repair strategies is the difficulty in achieving functionality that is equal to that of the preinjured state, which makes it necessary to explore new growth factors and cells to accomplish the task. In addition, we need to optimize the mechanical stimuli and postsurgical rehabilitation. Tissue engineering may provide promising solutions to tendon or ligament repair in difficult cases such as anterior cruciate ligament (ACL) rupture.
Arthritis
Rheumatoid and degenerative arthritis affects a large proportion of the population and are leading causes of disability. The role of inflammation in the pathogenesis of arthritis has been well established, and several groups of drugs have been developed to tackle inflammation and thus modify the progression of disease. One example is the use of tumor necrosis factor-α antagonists in rheumatoid arthritis. Recent studies have also focused on the role of stem cells in the pathogenesis of arthritis and on the therapeutic effects of mesenchymal stem cells (MSCs) on arthritis, taking advantage of the immune-regulatory effects of MSCs.
Mesenchymal Stem Cells
Stem cell biology is a hot topic in the field of orthopedic research. Experimental studies have shown that stem cells that contribute to fracture healing could derive from peripheral circulation and bone marrow. Transplanted MSCs can differentiate into osteoblasts and chondrocytes in fracture calluses. In addition, MSCs have paracrine effects by expressing potent growth factors and cytokines that modulate angiogenesis, inflammation, and stem cell recruitment/differentiation. The paracrine effects of MSCs in fracture healing have not been fully determined. There is evidence showing that MSC-conditioned medium can improve fracture healing. 4 Current research on MSCs is hindered by the lack of specific surface markers and the inefficiency of in vivo cell tracking techniques.
One recent major achievement in stem cell research is the discovery of induced pluripotent stem (iPS) cells. The iPS cells were first established by retrovirus-mediated transduction of four transcription factors (c-Myc, Oct3/4, SOX2, and Klf4) into mouse fibroblasts or human fibroblasts. The current trend is to use fewer transcription factors or small chemical compounds to reprogram somatic cells. iPS cells have the potential to differentiate toward osteoblasts, 5 indicating their value in bone regeneration. However, the risk of tumorogenesis of iPS cells needs to be addressed prior to clinical application.
2.1.2 Infrastructure and Equipment of a Biology Laboratory
The infrastructure required to run an orthopedic basic research laboratory is similar to any other biological laboratory. Fume hoods are required to vent noxious and dangerous chemicals. An animal housing facility is necessary if work will be performed on any in vivo model. If work is to be performed on established or primary cell lines, then a separate cell culture room should be considered. By isolating cell culture facilities, reduced foot traffic around the incubators and hoods will aid in keeping cultures free of bacteria and mold. Another part of the laboratory should be set aside for processing, sectioning, and staining of histological specimens. This area should be located in a “dust-free” area away from drafts that will create difficulty handling ribbons of histology sections. Work with radioactive materials can be made safer by defining and restricting use of these materials to dedicated areas of the laboratory. Similarly, a dedicated imaging suite that contains all of the microscopes that will be used for documentation and analysis of data will allow undisturbed specimen viewing, will allow the room to be darkened for specialized imaging such as epifluorescence, and will reduce the amount of dust that accumulates on working parts of the microscope. Basic equipment for a biology laboratory is listed in Table 2.1.
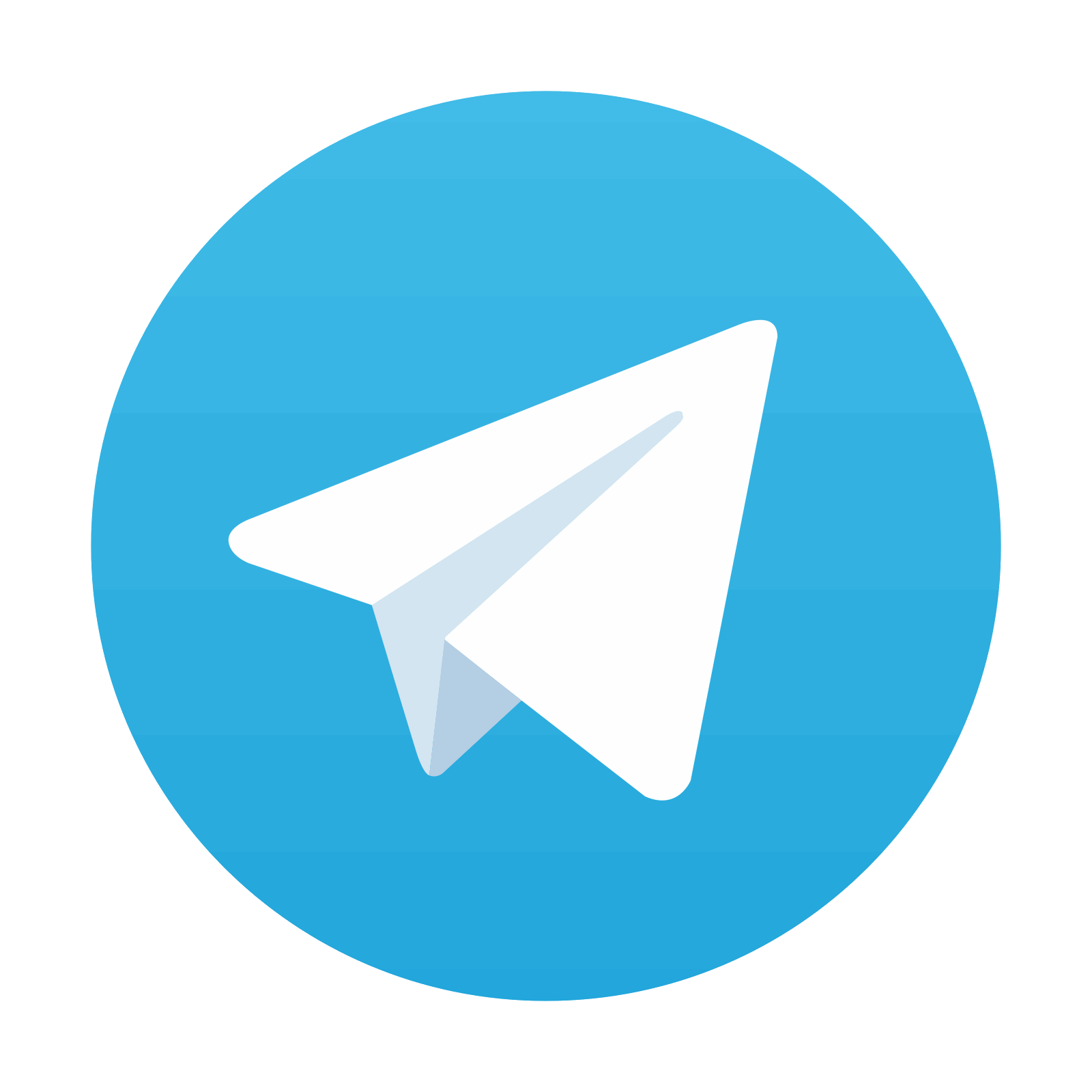
Stay updated, free articles. Join our Telegram channel

Full access? Get Clinical Tree
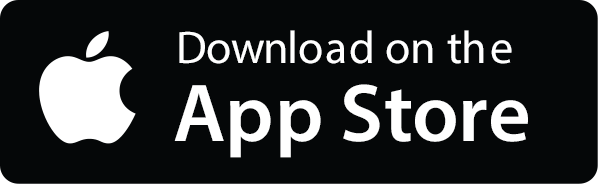
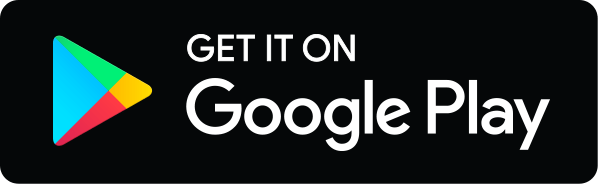
