2.9.1 Computer-assisted closed techniques of reduction and fixation
1 Introduction
Formal internal fixation of acetabular fractures traditionally has required large surgical exposures. These exposures can be associated with significant complications, including infection, wound-healing problems, major blood vessel or nerve injury, denervation or devascularization of the abductors, and heterotopic ossification. These complications largely are related to the surgical exposure itself rather than to the initial traumatic injury. Therefore, it seems reasonable to consider percutaneous stabilization of selected acetabular fractures to avoid exposing the trauma patient to the morbidity associated with an extensive surgical exposure.
The following fractures may be amenable to minimally invasive surgical fixation:
Nondisplaced (< 2 mm) but potentially unstable fractures involving the weight-bearing dome: Transtectal transverse fractures with roof arcs measuring < 45° fall into this category [1]. Tornetta [2] described a technique for stressing nondisplaced fractures under image intensifier guidance that helps confirm potential instability in slightly displaced fractures. Percutaneous stabilization of these fractures may prevent late fracture displacement and allow beneficial early mobilization of the trauma patient. Transverse fractures sometimes present as nonunions after attempted closed management and may be amenable to late stabilization with lag screws.
Slightly displaced fractures (3−5 mm) with gap displacement that may be reduced with percutaneously placed lag screws: In the absence of translational displacement, certain anterior column, transverse, anterior column/posterior hemitransverse, and posterior column fractures can be adequately fixed with large-diameter partially threaded lag screws placed perpendicular to the fracture lines to effect a reduction of the residual displacement through compression of the fracture gap.
Displaced fractures (> 5 mm) that fit into one of the above two categories following a closed reduction maneuver: Transverse and column fractures with subluxation or dislocation of the femoral head occasionally may assume an acceptable position following a maneuver to reduce the femoral head beneath the weight-bearing dome. The surgeon also may apply preoperative or intraoperative traction to improve the reduction. A temporary external fixator may be used to apply distraction through both trochanters, or an instrumented fracture table may help to reduce hip subluxation before fixation in transverse and posterior column fractures. Selected anterior column fractures also may be reduced with Schanz pin joysticks placed in both iliac wings and the reduction may be held with temporary external fixator during percutaneous fixation.
Displaced both-column fractures with good secondary congruity: In the both-column fracture that has medialized with gap displacement but has good secondary congruity, the fracture can be stabilized in situ with two cannulated screws placed into the anteroposterior columns. This can prevent further fracture displacement and eliminates the need for prolonged traction or restricted mobilization of the patient. Reduction can sometimes be improved with lateral and longitudinal traction on a fracture table with a lateral traction attachment. Percutaneous stabilization allows the elderly patient with the typical geriatric both-column fracture to be mobilized immediately; the hip may later be reconstructed, if necessary, by elective total hip arthroplasty after the patient’s medical status is optimized.
Displaced acetabular fractures in morbidly obese patients: In this situation, the trauma surgeon may be willing to accept somewhat greater final fracture displacement than usual, as the perceived risk of surgical complications related to a formal surgical exposure is increased. Precise percutaneous lag screw placement may provide some reduction and stabilize the fracture adequately to allow mobilization, while minimizing risks related to an extensile surgical exposure. It may be more prudent to accept 3 mm of articular displacement in a large patient than to strive for an anatomical reduction through an extensive open exposure.
Fractures of the posterior wall of the acetabulum are rarely amenable to percutaneous reduction and fixation. Given the anatomy of the sciatic nerve in proximity to the fracture fragment, it is difficult to safely place percutaneous screws across posterior wall fractures without risking nerve injury. Open reduction is also usually necessary to adequately debride the hip joint and reduce marginal impaction. The unstable posterior wall fracture/dislocation remains an indication for formal open reduction and internal fixation using a buttress plate, with or without supplemental lag screw or spring plate fixation.
The surgeon planning percutaneous fixation of any acetabular fracture must be mindful that these techniques have not yet been validated in randomized clinical trials. The early clinical results of percutaneous fixation have been encouraging, both in decreasing hospital stay and morbidity in longitudinal studies and in case reports for selected fractures [3−7]. These techniques should be performed only by experienced acetabular fracture surgeons in the event that a change in surgical plan and conversion to an open procedure is necessary. A thorough knowledge of the nuances of pelvic osseous and soft-tissue anatomy is necessary to plan trajectories and safely place percutaneous screws; advanced appreciation of radiographic anatomy is essential when relying on nonstandard image intensifier projections to provide guidance [8]. The surgeon must always be prepared to revert to a formal open approach in the event of inadequate imaging or inadequate reduction for percutaneous technique. It is unlikely that an inadequately reduced fracture treated with percutaneous technique will have better outcome than a well-reduced fracture treated with standard open technique in the absence of complications related to an open approach.
Surgical navigation (computer-assisted surgery) has matured over the past two decades and is now available in most hospitals. Images obtained from a standard or 3-D C-arm may be stored on a navigation workstation and used for precise placement of screws without relying on repetitive or real-time imaging. The new capability of obtaining a computed tomography (CT)-like dataset intraoperatively using cone-beam CT technology on a C-arm has eliminated the need to rely on a preoperative CT for navigation, as has been done in the past. In addition, C-arm-based 2-D (image intensification) and 3-D navigation are self-registering, eliminating the need for a time-consuming registration step to match the patient’s anatomy to the model. Navigation facilitates accurate screw placement while minimizing radiation exposure to both patient and surgeon. These systems were initially expensive, but the cost of bringing this technology into a hospital has decreased to about $100,000.
2 Early experience
Percutaneous acetabular fracture fixation was first performed under CT guidance by placing the patient into a standard CT scanner. With this technique, both the patient and the rest of the operating room (eg, scrub nurse, instruments, bean bag, anesthesiologist, surgeon, and radiologist) were brought into a standard CT suite. Patients were logrolled into the lateral position, and the CT gantry angled perpendicular to the fracture. The patient was prepared and draped in the usual fashion, and run back and forth into the scanner to identify appropriate starting points and trajectories for screws. Additional CT images were obtained after placing needles and later guide wires along the path of intended fixation before placing the actual implant (cannulated screw). Final screw position and fracture reduction by lag screw technique could be easily confirmed with final CT images ( Fig 2.9.1-1 ). Twenty-five patients were treated using this technique from 1990 to 1995 [9]. Most fractures stabilized with this technique were anterior column, transverse, and combined (anterior column/posterior hemitransverse) types and usually were stabilized using two or three large-diameter 7.0 or 7.3 mm cannulated screws. Using lag screws placed perpendicular to the plane of fracture displacement, the average preoperative displacement of 7 mm was reduced to 2 mm. For patients with isolated injuries, the average hospital stay after fixation was 2 days. Three patients with residual displacement of 5−8 mm eventually underwent total hip arthroplasty and were considered failures in patient selection. In the remaining patients, for whom a minimum of 2-year follow-up was available, the average self-administered hip score was 89 of 100 possible points [10]. This series helped to define the indications for minimally invasive acetabular fracture fixation and also provided support for continued clinical use of the technique.

The CT-guided technique had several limitations. Performing an invasive procedure in a room that was not designed for this purpose was a concern; in fact, one screw of the 60 placed became infected and required late removal. Screws also had to be placed in plane with the CT gantry; this limited the possible screw trajectories, as the gantry can only be angled about 20° before contacting the patient. Reduction maneuvers and traction could not be easily applied inside the CT scanner. For these reasons, only 16% of patients with acetabular fractures admitted to the author’s institution during the study period could be treated using this technique. Even when proficiency is achieved, the procedure often required more than 45 minutes for each screw inserted. Patients were also exposed to significant doses of radiation during the procedure, as up to 45 individual CT slices per screw were sometimes required for accurate placement.
Standard C-arm image intensification (without relying on cross-sectional CT imaging) is sometimes sufficient for percutaneous placement of screws about the pelvis and acetabulum. The biggest limitation of this technique is that imaging is available in only one plane at a time. The surgeon must position the implant in one view, and then obtain additional images in other planes for “trial and error” placement of guide wires or screws. Significant operating room time is expended while the image intensification technician positions the C-arm to supply views in multiple planes, both during and after implant placement. Although most surgeons are comfortable with this technique for hip fractures and intramedullary nailing, this procedure exposes both the patient and surgeon to significant radiation doses. When working around the complex anatomy of the pelvis, an erroneous first pass of the guide wire can potentially have disastrous consequences when relying on only one planar image at a time for guidance.
For all these reasons, it became clear that a new technology was needed that would allow minimally invasive acetabular fracture fixation to be brought back to the operating room, while also allowing more versatile screw trajectories with less radiation exposure and greater first-pass accuracy. The advent of computer-assisted orthopedic surgery (CAOS) provided this technology.
3 Computer-assisted orthopedic surgery (image- guided surgery)
Computer-assisted orthopedic surgery makes use of stored imaging data to provide guidance during surgical procedures. Patient-specific imaging data is harvested using conventional techniques (CT or image intensification) and stored on a computer workstation. The computer system can then track special surgical instruments relative to the stored imaging data, providing the surgeon with information about the position of the instruments and implants relative to the patient’s anatomy.
Surgeons are experienced in minimally invasive techniques using 2-D video output during either arthroscopy or C-arm image intensification. And CAOS takes this a step further by providing access to either a 3-D dataset (3-D CT guidance, or 3-D navigation) or multiple simultaneously displayed stored image intensification images (virtual image intensification). Most current image-guided surgery systems use an optical tracking system (digital camera array) to follow both the position of the patient and special surgical instruments during the course of the procedure ( Fig 2.9.1-2 ). The instruments are fitted with light-emitting diodes or reflectors that can be precisely localized by the camera. The predicted position of the surgical instruments is then displayed on a computer monitor relative to the position of the stored images. This process is known as surgical navigation. In the author’s experience, optimal accuracy is provided by tracking the drill guide used to place guide wires for cannulated screws, rather than by tracking the drill itself.
The first 3-D CT systems were used in intracranial neurosurgery in the late 1980s. These systems relied on a stored patient-specific CT dataset to build a virtual model of the patient’s anatomy. Datasets of surgical plans or trajectories may be devised and stored preoperatively. Through a process known as registration, the surgeon would then match the patient’s anatomy to the virtual model. The computer could then display the position of instruments, such as probes or drill guides relative to the virtual model. A 3-D CT system became available for spine surgery in the early 1990s. With minor software and instrument modifications, this system was first adapted for pelvic and acetabular fracture fixation in 1996. Approval for clinical use was granted in 1997 following accuracy validation in a cadaver study [11, 12].

Virtual fluoroscopy (C-arm navigation) was refined and approved for clinical use in 1999 [13]. Before this time, the distortion inherent in analog C-arm images prevented their use in surgical navigation. It was not until software algorithms were developed to allow rapid warping or correction of analog C-arm images to make them optically correct that these stored images could be used for guidance. A standard C-arm unit is used to harvest multiple 2-D images in the operating room. A camera tracks the position of the C-arm unit during image acquisition, and the images are warped, stored, and displayed on a workstation. Up to four individual stored images may be displayed at any one time. The camera can then track special instruments and displays their position relative to each of the stored images. The arrival of image intensifier navigation greatly broadened the potential applications of CAOS in orthopedic trauma. Computer- assisted technique can be applied to essentially any procedure that traditionally relies on intraoperative C-arm imaging for fracture reduction and implant placement. Laptop computers are now powerful enough to run the software for navigation, and most systems have a sterile touchscreen interface that can easily be manipulated by the surgeon.
Several manufacturers now produce motorized portable C-arm units that are capable of rotating 180° or more to harvest a 3-D dataset. While not strictly equivalent to standard CT, this so-called cone-beam technology produces high-quality cross-sectional imaging intraoperatively that can be used to assess fracture reduction. When interfaced with a navigation system, the surgeon may view two orthogonal cross-sectional images in real time relative to the position of a navigated drill guide. This technique is referred to as 3-D navigation and differs from the CT-guided technique used in the past. The 3-D navigation has essentially eliminated the need to rely on a preoperative CT scan for navigation guidance, as was done in the past. Early experience suggests that 3-D navigation may be superior to 2-D navigation for placement of periacetabular screws, in terms of accuracy and total radiation exposure [14]. Regardless of the surgeon’s choice of navigation technique, the newer C-arm-based navigation systems have essentially replaced the earlier techniques that relied on a separate preoperative CT scan.
Surgeons generally have been relatively slow to embrace computer-assisted technology, primarily due to concerns about cost and complexity of the systems. Many surgeons are unaware that these systems and software packages are now commercially available for trauma. The European orthopedic community appears to be adopting the use of these systems more quickly than surgeons in the United States. The initial enthusiasm for these systems concentrated on total joint and spine applications, and hundreds of systems were installed in hospitals in the United States. Interest in the total joint applications has waned, and it now appears that orthopedic trauma may benefit the most from advances in navigation. Most large hospitals now have one or more navigation systems in place, and transitioning to navigated trauma surgery requires only a modest investment in instruments and software. As surgeons-in-training are exposed to this technology, it is expected that utilization will increase.
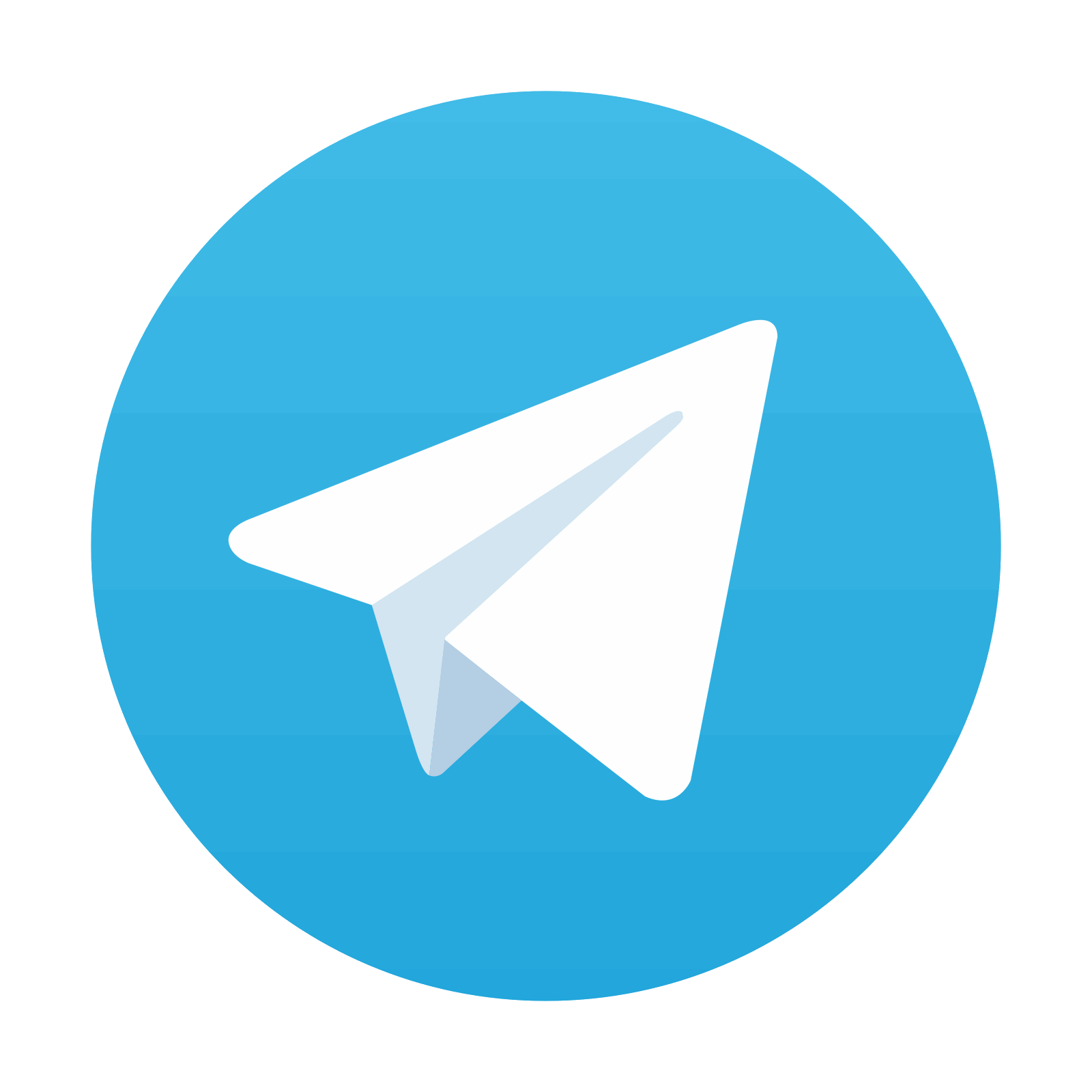
Stay updated, free articles. Join our Telegram channel

Full access? Get Clinical Tree
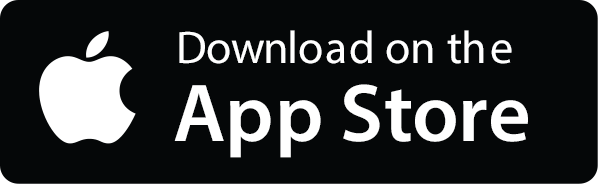
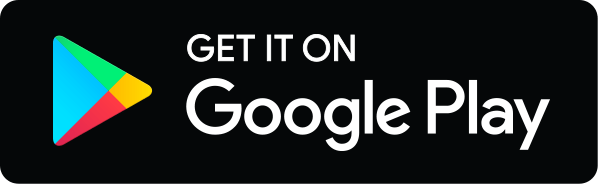