2.7 Specific surgical approaches and technique
1 Introduction
Prior to the classic work by Judet and coworkers [1, 2] and Letournel [2–6], there was little understanding of the complex pathoanatomy and proper surgical management of acetabular fractures. However, following the introduction of their classification scheme and novel surgical approaches, the last three decades have seen improvements in surgical approaches, techniques of reduction, and implants, leading to more consistently good results [1–5, 7–16]. However, the management of these difficult fractures remains a challenging problem.
The care of the patient with a pelvic injury begins with prompt resuscitation performed by a trained multidisciplinary trauma team and accurate assessment and stabilization of any life-threatening injuries. Once the patient has been stabilized, definitive treatment should only be undertaken by a surgeon experienced in handling such fractures.
A patient’s functional outcome following an acetabular fracture is dependent on several factors—some controllable but others injury related and inevitable [5, 9, 12, 13, 16–19]. Of the surgical factors, the most important is obtaining an anatomical reduction of the articular surface and fixation stable enough to allow early range of motion while avoiding both acute and late complications. The achievement of these goals can be a demanding and frustrating task, which cannot be accomplished without the following resources [20]: (1) An experienced surgeon who uses atraumatic techniques, including the preservation of the blood supply to the femoral head and articular fragments without causing undue additional trauma; (2) a knowledgeable nursing team able to produce readily the proper and necessary reduction and fixation devices, including the specialized pelvic reduction clamp, instruments, and implants; (3) an accomplished anesthesiologist able to paralyze the patient and able to respond to any eventuality; (4) ± a neurophysiologist to provide nerve monitoring; and (5) an image intensifier table and the ability to obtain intraoperative image intensification or plain x-ray views of the pelvis and hip joint. A thorough understanding of the complex anatomy of the pelvis is necessary before surgical treatment because surgical outcomes significantly depend on an accurate diagnosis and an appropriately chosen and well-executed approach.
2 Indications for surgery and perioperative considerations
Because malreduction or subluxation of the hip joint can lead to abnormal loading of the articular cartilage with subsequent joint arthrosis [4, 5, 9, 11, 12, 14, 21–25], acetabular fractures should be treated with the same principles granted to other intraarticular fractures. Therefore, the primary goal in their management is to perform an accurate reduction of the articular surface to obtain a congruent hip joint and therefore restore normal joint mechanics. To this end, the decision to perform surgery is based on many factors including the patient, the personality of the fracture, and the capabilities of the surgical team.
However, the patient’s medical condition (associated injuries, comorbidities, etc), physiological age, and functional needs are also key determinants of the treatment algorithm. For example, the presence of an unstable pelvic ring injury in combination with a displaced acetabular fracture poses not just a greater risk physiologically to the patient but also a challenge to the surgeon with respect to accurately reducing the acetabular fracture. Suzuki et al [26] called this a “devastating dyad,” which comprised 5% of all pelvic ring and acetabular injuries requiring admission in their series. They found that, in addition to these patients being significantly more injured compared with those who had sustained an isolated displaced acetabular fracture, residual displacement of the acetabulum after surgical treatment was often the result of incomplete reduction of the pelvic ring injury. Transverse-type patterns accounted for 61.2% of all acetabular fractures in this combined group with the most frequent pattern a transverse acetabular fracture with associated anterior disruption of the ipsilateral sacroiliac joint. Based on their findings, these authors recommended that accurate reduction of the posterior pelvic lesion be achieved before reconstruction of the acetabulum.
Knowledge of the acetabular fracture pattern can help assess the likelihood of other body system injuries as well as the physiological state of the patient with respect to blood loss [27, 28]. Posterior wall, posterior column, and posterior column plus posterior wall injuries are typically caused by axial and posteriorly directed loads as opposed to other acetabular fracture patterns, which are caused by more lateral or trochanteric loads. In one study [28] this latter group was noted to have a statistically higher association with retroperitoneal hematomas (33% vs 13%), and injuries to the spleen (12% vs 4%), liver (11% vs 5%), vascular (9% vs 0%), kidney (6% vs 1%), and bladder (8% vs 1%) compared to the former. In contrast most injuries associated with axial fracture patterns were skeletally based. Incidences of other injuries were not significantly different between the lateral and axial force groups. This included lung (28% vs 23%), lower extremity fractures (35% vs 38%), upper extremity fractures (23% vs 19%), spine fractures (17% vs 16%), and traumatic brain injuries (11% vs 7%). Interestingly, in this study [28] transverse plus posterior wall fractures exhibited intermediate characteristics between axial load and the remaining lateral load patterns. Another report [27] found that patients with isolated acetabular fractures were just as likely as those with isolated pelvic fractures to receive blood transfusions within the first 24 hours of admission. Importantly, both column, anterior column, anterior column with a posterior hemitransverse, and T-type fracture patterns were more likely to receive a blood transfusion (56%) than other fracture types (28%; P = .003). Both-column (8.8 units) and anterior column with a posterior hemitransverse (6.4 units) fractures required the largest volumes of blood.
A significant patient variable is obesity. This is a growing epidemic in many industrialized countries and presents a significant challenge to the pelvic surgeon. Acetabular fractures in these patients can be more difficult to reduce and complication rates are high. Porter et al [29] compared a nonobese group of patients with groups of obese (BMI > 30 kg/m2) and morbidly obese (BMI > 40 kg/m2) patients. Anatomical radiographic reductions were achieved in 72% of the nonobese patients, 70% of the obese patients, 72% of the nonmorbidly obese patients, and 61% of the morbidly obese patients. On postoperative computed tomographic (CT) scans, an acceptable reduction was obtained in 47% of the nonobese patients, 44% of the obese patients, 47% of the nonmorbidly obese patients, and 31% of the morbidly obese patients. This same group [30] also found that morbidly obese patients required an average operating time of 293 minutes with greater interoperative blood loss vs 250 minutes in nonmorbidly obese patients. Wound complications (46% vs 12%; P < .0001) and an overall complication rates (63% vs 24%; relative risk, 2.6) were also higher than in nonmorbidly obese patients.
Among the most challenging of comorbid situations is the presentation of a displaced acetabular fracture in the gravid patient. However, a team-oriented approach and the judicious use of radiographic imaging in these patients can minimize the risk to the baby while achieving an acceptable articular reduction. Porter et al [31] reported eight such cases (four posterior wall fractures, three transverse plus posterior wall fractures, and one posterior column fracture) with fetal gestational ages ranging from 5–26 weeks. At the time of surgery, patients with an isolated posterior wall fracture pattern were positioned laterally while the remaining patients were positioned prone. Care was taken to support and appropriately pad each patient’s abdomen and fetus. When a right lateral decubitus position was required, extra care was taken to support the gravid uterus to prevent compression of the vena cava. All surgeries were performed using a Kocher-Langenbeck (KL) surgical exposure and image intensifier used sparingly. No attempt was made to intraoperatively shield the abdomen from image intensification imaging or monitor the fetus. Postoperatively, both the patient and fetus were immediately evaluated by the obstetric service in the postanesthesia care unit. Infant delivery averaged 27 weeks from the time of acetabular fracture stabilization and all pregnancies reached 36 weeks. Apgar scores were normal in each child including one twin delivery.
With respect to the injury pattern itself, indications for operative fixation include displacement of the articular surface, joint incongruity, unacceptable roof arc measurements, incarceration of an intraarticular fragment within the hip joint, and any subluxation of the femoral head [12, 23, 32–38]. Although most [1, 5, 9, 11–15, 17, 32, 39–57] now agree that anatomical reduction of the weight-bearing surface and concentric reduction of the hip are essential for long-term satisfactory results, the degree of hip joint incongruity that can be tolerated still is not known. This may be due to the limitations of plain films (less accurate and poorer interobserver reliability) compared with CT in the diagnosis and assessment of acetabular fractures [50, 58–60].
Helfet and Schmeling [45] observed that an articular step off of > 2 mm or a gap of > 3 mm was associated with a fourfold increase in joint space narrowing at early follow-up. This was confirmed in a prospective study by Matta [9] who achieved an anatomical reduction in 71 of 262 acetabular fractures, with 83% of these patients having good or excellent outcomes at an average follow-up of 6 years. Of 29% with an imperfectly reduced acetabulum, good or excellent results were obtained in 68% of cases if the defect measured 2–3 mm, and only 50% if > 3 mm. Malkani et al [47] and Hak et al [43] have used anatomical specimen models to further support 2 mm or less as the appropriate prerequisite for an acceptable reduction. Considering the importance of the articular reduction with respect to outcome, percutaneous techniques, while having some value in acetabular fracture surgery, should only be considered in certain circumstances [61–65].
However, while achieving an anatomical reduction is critical, this alone may not be sufficient to restore function and achieve a good outcome. While the quality of the reduction appears to be the most important factor with respect to outcome, some [9, 14, 66]have found that the most clearly predictive initial factor is actually damage to the femoral head. Furthermore, numerous studies [17–19, 67]have linked comminution and impaction with poor results. Kreder et al [19] followed up 128 patients treated operatively for a posterior wall acetabular fracture and assessed outcomes using the Musculoskeletal Functional Assessment, Short-Form 36 scores, and x-rays. They found that their patients had profound functional deficits compared with the healthy population and that the fracture pattern, marginal impaction, and residual displacement of > 2 mm were associated with the development of arthritis, which related to poor function and the need for hip replacement.
The location of the acetabular fracture also is important. Matta et al [9, 23, 33, 34] have shown that an intact 45° roof arc and a congruent hip joint are mandatory to achieve a satisfactory result. Other authors [12, 38, 68, 69] have noted that fractures involving > 40% of the posterior wall will result in instability, whereas those affecting < 20% of the wall are stable. Building on this knowledge, Olson [35] developed strict criteria for consideration of nonoperative treatment: (1) the articular surface must be intact in the superior 10 mm of the joint on CT; (2) without traction, the femoral head must remain congruent with the superior acetabulum on the AP and 45° oblique views; and (3) a minimum of 50% of the posterior wall articular surface must be intact.
Yet such objective criteria cannot be considered absolute, as a number of recent reports [36, 38] have demonstrated that although satisfying the preceding guidelines, some fractures still require stabilization because of dynamic instability. This particular entity can be identified by performing dynamic stress views while the patient is sedated or anesthetized. Hip joint stability determined by an experienced surgeon using dynamic stress image intensifier with the patient under general anesthesia appears to be predictive of a good result.
Grimshaw and Moed [37] followed up 18 such patients treated nonoperatively after a posterior wall fracture for a minimum of 2 years. The average modified Merle d’Aubigné score of their entire study group was good, and all 15 patients’ x-rays demonstrated a congruent joint with a normal joint space and no evidence of posttraumatic arthritis.
Finally, Olson et al [51] have found that small defects in the posterior wall produce major changes in the articular contact area that may predispose to late posttraumatic degenerative changes even in the presence of clinical stability. Another controversy involves displaced both-column fractures that demonstrate secondary congruence, which some have argued may not routinely require operative reduction and stabilization, especially in the older patient [5, 12, 36, 44, 48, 70]. However, one biomechanical study of anatomical specimen [71] has shown that abnormal increases in stress concentration still occur in the dome of the acetabulum adjacent to the fracture line in such cases.
3 The geriatric patient
With the geriatric patient population, concerns arise regarding the health risks posed by performing a major surgical procedure on an older patient with multiple medical comorbidities and potentially a fragile physiological reserve. Additionally, there are the limitations of poor bone stock, a potential inability to aggressively rehabilitate the patient, and questions as to whether the patient’s functional needs even dictate fracture reconstruction. Therefore, nonoperative treatment with or without delayed total hip arthroplasty (THA) or possibly primary THA with or without reconstruction of the acetabulum are options. However, outcomes of non-operative treatment of acetabular fractures are often just as dismal as they are in the younger patient population. Thus age alone should not be a contraindication to surgical reconstruction of an acetabular fracture. Indeed, many studies [17, 57, 70, 72, 73] have shown good functional outcomes after open reduction and internal fixation of these fractures in the elderly. Additionally, to minimize stress on these potentially fragile patients more limited approaches can be considered. For example, using only the lateral two windows of the ilioinguinal approach can lead to a significant reduction in both blood loss and operative time [73].
However, the elderly are at risk of fracture patterns that are associated with poor outcomes [18, 17, 67], and the quality of reduction is strongly related to age, with a decrease in accuracy noted in older patients [9, 13, 14]. At the same time, while a perfect anatomical reduction of the acetabular fracture should always remain a goal—in the elderly this may not be as clinically important as it is in younger patients. Miller et al [70] evaluated 45 patients with a mean age of 67 years (range, 59–82 years) at the time of surgery. X-rays in 26 patients (58%) identified an anatomical reduction, 13 (29%) reductions were imperfect, and 6 (13%) were poor. Not surprisingly the maximum displacement identified on CT revealed that no reduction was anatomical; 23 (51%) were imperfect and 22 (49%) were poor, although the displacement was not always at the weight-bearing dome. In contrast to what would be expected from these radio-graphic findings, SF-36 scores showed functional outcomes comparable with those of the general elderly population, with no correlation with the radiological reduction.
In another review, Carroll et al [57] identified 93 elderly patients (mean age, 67 years) in a prospective database who had undergone operative management of an acetabular fracture. At an average follow-up of 5 years, the overall rate of hip replacement was 31%. Poor fracture reduction (P < .02), the development of avascular necrosis (P < .001), and previous contralateral hip arthroplasty (P = .02) were statistically associated with the need for secondary surgeries. However, functional outcomes measured by the Musculoskeletal Functional Assessment, Short Musculoskeletal Functional Assessment, and SF-36 compared favorably with similar scores reported for acetabular fractures in younger populations and age-matched “non-injured” norms. The authors concluded that there was an acceptably low rate of major complications in their patient group with nearly 70% achieving functional outcomes similar to age- and injury-matched control subjects without the need for secondary surgeries.
Anglen et al [17] also found that anatomical reduction of an acetabular fracture in 27 patients with an average age of 71.6 years (range, 61–88 years) closely correlated with good to excellent radiographic results, and that functional outcomes were similar to age-matched control subjects. However, they also identified an important and specific radiographic finding (superomedial dome impaction) that was predictive of failure. Named the “Gull sign,” patients in this subgroup had inadequate reduction, early fixation failure, or medial/superior joint narrowing and subluxation. Other studies have also identified high rates of comminution and impaction in the elderly [17–19, 74], femoral head injury [9, 66, 74], and the presence of a subchondral insufficiency fracture [67] as risks for a poor outcome, and therefore, relative indications to consider arthroplasty.
Ferguson et al [18] used a prospective database to compare the clinical details and patterns of displaced acetabular fractures in 235 patients who were older than 60 years with a second group of 1074 patients younger than 60 years. The incidence of elderly patients with acetabular fractures increased by 2.4-fold between the first and second halves of their 27-year study period (10% vs 24%, P < .001). Fractures with displacement of the anterior column were significantly more common in the elderly compared with the younger patients (64% vs 43%, P < .001). Common radiological features (many predictive of poor outcomes in other studies) of fracture patterns in the elderly included a separate quadrilateral-plate component (50.8%) and roof impaction (40%) in the anterior fractures; and comminution (44%) and marginal impaction (38%) in posterior wall fractures. Along these same lines, Kreder et al [19] recommended consideration of immediate total hip replacement for patients older than 50 years with marginal impaction and comminution of the wall, since 7 (54%) of 13 such patients in their study required early hip replacement.
Using the above at-risk criteria (when complicating features significantly diminished the likelihood of a favorable outcome after open reduction and internal fixation of a displaced acetabular fracture), Mears and Velyvis [74] performed acute THA in 57 patients with a mean age of 69 years (range, 26–89 years). The mean time from the injury to arthroplasty was 6 days (range, 1–21 days). Their indications included intraarticular comminution as well as full-thickness abrasive loss of the articular cartilage, impaction of the femoral head, and impaction of the acetabulum that involved > 40% of the joint surface, including the weight-bearing dome. At a mean follow-up of 8.1 years the average Harris Hip Score was 89 points and 79% of their patients had an excellent or good outcome. During the initial 6 postoperative weeks, the acetabular cups subsided an average of 3 mm medially and 2 mm vertically but then stabilized, and none were loose at the latest follow-up. Six patients had excessive medialization of the cup but none had late loosening or osteolysis. Nine cups (16%) had notable polyethylene wear but none were revised. There were three late procedures: one for revision of a malaligned cup because of recurrent dislocations, one for removal of hardware from the greater trochanter, and one for excision of heterotopic bone.
For complex fracture patterns, either augmentation of the cup [75], specially designed cups [74, 76], or separate reconstruction of the acetabular fracture as a combined hip procedure [77, 78] can achieve good results. In a retrospective clinical study Tidermark et al [75] treated ten patients with acetabular fracture (mean age, 73 years) with primary THA supported by a reinforcement ring (Burch-Schneider Antiprotrusion Cage) and autogenous bone grafting. The mean operating time was 159 minutes (range, 125–185 minutes) and mean intraoperative blood loss 1,100 mL (range, 700–1,600 mL). At an average follow-up of 38 months, the reinforcement ring was stable, the bone graft had completely incorporated, and there were no signs of loosening of the acetabular component or stem in any of the patients. Good functional outcomes were noted with all patients still independent of ambulators despite a slightly increased need for walking aids.
Boraiah et al [77] described a combined hip procedure where in a single stage, open reduction and internal fixation for a displaced acetabular fracture was performed followed by primary THA by a team that included both a fellowship-trained orthopedic trauma surgeon and a fellowship-trained adult reconstructive surgeon. Their group included one transverse, one anterior column with a posterior hemitransverse, one both-column, and 15 posterior wall fractures. Of 15 posterior wall fractures, one was associated with a posterior column fracture, one with a dome fracture, two with transverse fractures, and nine with femoral head impaction. Of 18 patients in the study, 14 were followed up for more than 2 years (average, 3.9 years; range, 1–10.1 years). All but one patient healed successfully. One patient required revision and placement of a constrained prosthesis due to early failure of acetabular component. Harris Hip Scores ranged from 78–99, with a mean of 88. The x-rays showed an average medial displacement of 1.2 mm (range, 0–3 mm) and an average vertical displacement of 1.3 mm (range, 0–4 mm), but no radiographic evidence of acetabular component loosening.
After an aggressive medical workup, Herscovici et al [78] treated 22 elderly patients using a combined hip procedure. There were nine transverse plus posterior wall patterns, seven anterior column with a posterior hemitransverse patterns, and six both-column injuries. The procedure involved a standard open reduction and internal fixation technique followed by immediate THA under the same anesthesia. The procedures averaged 232 minutes with a blood loss of 1163 mL. At an average follow-up of 29.4 months, hip motion averaged 102° of flexion, 32° of abduction, and 16° of adduction. Harris Hip Scores averaged 74. Five patients required revisions for osteolysis or multiple hip dislocations.
4 Timing of surgery
Fractures of the acetabulum often pose many diagnostic and technical problems. Therefore, acute open reduction and internal fixation rarely are indicated. Exceptions to this include dislocations that cannot be reduced by closed means, an incarcerated intraarticular fragment following closed reduction, and unstable posterior dislocations that cannot be held in the reduced position because of the marked deficiency of the posterior wall. The former should generally be considered a surgical emergency and a reduction of the dislocation performed as soon as possible after the injury. However, the exact minimal interval to reduction of a dislocated hip may be less important than previously believed. In one study, Bhandari et al [56] reported that the quality of fracture reduction was the only significant predictor of radiological grade, clinical function, and the development of posttraumatic arthritis (P < .001) in 109 patients who had an acetabular fracture associated with a posterior dislocation of the hip.
Progressive or sciatic nerve palsy that develops after reduction of the dislocation also should be considered a surgical emergency. Rare indications for emergent surgical intervention include an open fracture of the acetabulum and an anterior column fracture associated with a femoral artery lesion [20]. In all other circumstances, the timing of surgery is more dependent on stabilization of associated visceral, skeletal, and soft-tissue injuries, the completion of all imaging studies necessary for careful preoperative planning, and the availability of an experienced surgeon. A difficult procedure then can be performed on an elective basis with a more experienced operating team, usually between day 2 and day 5 postinjury. Although deferral of operative treatment for up to 2 weeks usually provides only a minor compromise to the ease of surgical reduction [5, 9, 12, 14, 79], delays of more than 1 week should be avoided if possible. One study [80] has noted an average time to surgery of 11 days for fractures with an acceptable reduction, whereas another [81] demonstrated a correlation with poorer results when surgical stabilization was undertaken after 7 days. Madhu et al [79] retrospectively reviewed 237 patients with displaced fractures of the acetabulum and found that the time to surgery was a significant predictor of radiological and functional outcome for both elementary and associated displaced fractures of the acetabulum (Letournel classification). An anatomical reduction was more likely if surgery was performed within 15 days for elementary patterns and 5 days for associated patterns. Additionally, a good to excellent functional outcome was more likely when surgery was performed within 15 days for elementary fracture patterns, and by 10 days for associated fracture types. Beyond 2 weeks, an anatomical reduction becomes progressively harder to obtain because of the increasingly difficult task of meticulously taking down varying amounts of callus, organizing hematoma, and granulation tissue [3, 5, 11, 12, 14, 39, 40, 42, 48, 55, 82–88]. Letournel himself found that by this time, his rate of anatomical reduction had dropped from 75–62% of cases [5]. Dean and Moed [88] noted poor outcomes in three of four patients with posterior wall acetabular fractures undergoing surgical treatment beyond 3 weeks. Two of the three total hip arthroplasties performed were required within only 18 months of the initial reconstructive effort.
5 Preoperative planning
Because patients with acetabular fractures often present with associated visceral injuries and skeletal injuries to such structures as the pelvis, femur, and ipsilateral knee, the preoperative evaluation needs to begin with a thorough physical examination and appropriate trauma workup. Accurate assessment of the patient’s neurological status also is mandatory because the incidence of preoperative, post-traumatic sciatic nerve compromise following acetabular fractures has ranged from 12–38% [5, 11, 32, 34, 89–94]. For example, Giannoudis et al [94] found that 27 (19.9%) of 136 patients who underwent open reduction and internal fixation of an acetabular fracture had some form of sciatic nerve injury. At initial presentation, 13 patients had a complete foot-drop, ten had weakness of the foot, and four had burning pain and altered sensation over the dorsum of the foot. Of interest, nine of the 13 patients with a foot-drop had evidence of both a proximal acetabular (sciatic) and a distal knee (neck of fibula) nerve lesion, which the authors termed “the double-crush syndrome.” At the final follow-up, clinical examination and electromyography (EMG) studies showed full recovery in five of the ten patients with initial muscle weakness and complete resolution in all four patients with sensory symptoms (burning pain and hyperesthesia). However, at a mean follow-up of 4.3 years, only two of the 13 patients who presented initially with complete foot-drop had some improvement in sensory and motor function, while no improvement was seen in the others, including all nine with the double-crush lesion.
Another important part of the preoperative workup includes both prophylaxis and surveillance for deep vein thrombosis (DVT), which has been reported to occur in as many as 35–60% of patients with pelvic fractures [95–98]. Patients with acetabular fractures awaiting transfer to another institution for their definitive care are at especially high risk for DVT and require prophylaxis. Steele et al [99] highlighted the importance of the early administration of chemical prophylaxis. In 103 consecutive patients prospectively studied after undergoing surgical stabilization of pelvic and acetabular fractures, the authors noted a proximal DVT rate of 10% and pulmonary embolus rate of 5%. Proximal DVT developed in only 2 (3%) of 64 patients who had received low-molecular-weight heparin within 24 hours of injury, but in 8 (22%) of 36 patients who received low low-molecular-weight heparin more than 24 hours after the injury (P < .01). Letournel and Judet [5] reported a 3% incidence of clinically evident DVT with four fatal and eight minor pulmonary emboli in a series of 569 patients, despite most receiving anticoagulant prophylaxis. In a prospective study, Geerts et al [95] demonstrated a 60% incidence of DVT in patients with primary lower extremity orthopedic injuries, and Kudsk et al [96] observed a 60% incidence of silent DVT by venography in patients with multiple trauma immobilized 10 days or more. Montgomery and et al [100] used magnetic resonance venography (MRV) to evaluate 45 consecutive patients with displaced acetabular fracture, noting 24 asymptomatic thrombi in the thigh and pelvis of 15 patients (33%).
Although most studies have found MRV to be highly specific and sensitive [98, 100–103], some have questioned its value [104–106]. Stover et al [105] reported a low incidence of MRV and CT venography detected thromboses in their series of 30 subjects. Deep vein thrombosis was detected in five patients; however, only one subject had DVT identified by both imaging techniques and only one of the five identified DVTs was confirmed by standard contrast venography. Other authors have questioned whether the efficacy of present day screen techniques merits their use at all. Borer et al [106] reviewed 973 patients with pelvic or acetabular fracture treated over two consecutive 2-year periods: the first with routine screening and the second when routine screening had been discontinued. Discontinuation of screening for DVT did not change the rate of pulmonary embolus in their patients with closed fractures of the pelvis or acetabulum (1.4% without screening vs 2% with routine screening). The overall rate of pulmonary embolus was 17 (1.7%) of 973 patients, and the rate of fatal pulmonary embolus was only 3 (0.31%) of 973 patients. Finally, they calculated that the estimated cost of the 237 MRVs and 302 diagnostic ultrasounds performed during the first half of their study was $464,532, indicating a significant cost incurred for questionable value.
Despite the present ongoing controversy regarding optimal preoperative prophylaxis and surveillance, during the preoperative period we prefer low-molecular-weight heparin combined with an intermittent pneumatic compression device, and MRV to rule out DVT [97, 100, 101]. If a significant clot is diagnosed, most patients undergo placement of an inferior vena caval filter and are treated with intravenous heparin before surgery. While controversy exists with respect to prophylactic inferior vena cavals because they appear to be safe and effective in preventing pulmonary embolus in patients with pelvic trauma and established venous thrombosis, their use should be considered for polytrauma and intensive care unit patients or when anticoagulation is an issue [107–109].
A thorough understanding of the complex pathoanatomy involved is extremely important before attempting operative fixation. An accurate diagnosis of the basic fracture pattern can be accomplished with three basic roentgenograms described by Judet et al [1]: an AP view of the pelvis, an iliac oblique view of the acetabulum, and an obturator oblique view of the acetabulum. This initial roentgenographic survey, with accurate delineation of all fracture lines, provides vital information that dictates the treatment plan. If surgery is indicated, this information also helps the surgeon select the optimal surgical approach. To this end, the classification scheme of Letournel helps describe the fracture and determine the surgical approach, while having substantial inter observer reliability (kappa > 0.7) when used by surgeons who have been taught how to interpret the images or by those who treat acetabular fractures on a regular basis [110]. The addition of conventional CT scans with thin slice (1–3 mm) axial cuts allows for better appreciation of the extent of injury. Although CT provides little additional information regarding simple column fractures, it is particularly helpful in evaluating the integrity of the dome, posterior lip, sacrum, sacroiliac joint, and femoral head; and delineating the rotation of the columns or presence of intraarticular bony fragments [35, 50, 58–60, 111, 112]. Although obtaining both the plain films and a CT scan may seem redundant, intraoperative decision making and technique depends on a clear understanding of the former. These are superior for evaluating hip joint congruency and can be compared with intraoperative and postoperative images.
Advances in imaging software technology have led to the development of 3-D CT, which can help provide a better understanding of the spatial relationship of the fracture pattern relative to the pelvis as a whole. Although its role is not yet altogether defined, it can be useful when viewed real time on a monitor that allows visualization of the fracture from various projections. Moreover, 3-D CT scans can be used in conjunction with plain roentgenograms including Judet views and axial CT scans for teaching to facilitate preoperative planning, and especially in late cases, such as acetabular nonunions and malunions. It is often helpful to transfer the fracture lines from these radiographic studies to a model pelvis. Such a 3-D perspective can assist the surgeon in the selection of the optimal surgical approach, reduction technique, and method of fixation for the major fracture fragments. These decisions are made with the expectation that the entire procedure can be performed through a single operative incision.
6 Selection of the optimal surgical approach
The surgeon must use a surgical exposure that will afford the best opportunity to restore joint congruency by anatomical reduction and stabilization of the articular surface, while resulting in the least morbidity. To this end, Mayo [10] has identified five major factors that affect this decision: (1) the fracture pattern; (2) the local soft-tissue conditions; (3) the presence of associated major systemic injuries; (4) the age and projected functional status of the patient; and (5) the interval from injury to surgery. Under most circumstances, the fracture pattern is the major determinant. Therefore, it is important to accurately classify the fracture using either the Letournel-Judet [3–5, 7] ( Table 2.7-1 ) or Comprehensive Classification [113] system ( Table 2.7-2 ) [7]. Importantly, any additional injuries to the pelvic ring also must be considered [26]. Finally, the selection of the proper surgical exposure also is to a large extent driven by the experience of the operating surgeon.
When open treatment is indicated, most acetabular fractures can be treated with a single more limited anterior or posterior surgical approach [4, 5, 10, 14, 37, 45, 52, 74, 114–121]. Using the technique of indirect reduction with a single nonextensile approach in 84 complex acetabular fractures involving two columns, Helfet and Schmeling [45, 116] reported a success rate of 91%, a deep infection rate of 0%, and an incidence of significant heterotopic ossification (HO) of 2%. Therefore, if an adequate preoperative evaluation of the patient and fracture pattern has been performed, then it is rare that a second surgical approach will be required. This is important because extensile exposures involve greater patient morbidity compared with single anterior or posterior approaches, including increased operative time, blood loss, infection, nerve injury abductor weakness, joint stiffness, and HO [5, 10, 11, 23, 32, 40, 42, 48, 54, 55, 66, 83, 122–127]. However, for more complex fracture patterns involving both acetabular columns and those delayed past 2–3 weeks, an extensile or combined anteroposterior surgical exposure may be necessary for visualization and reduction [3, 5, 11, 12, 40, 42, 47, 48, 52–55, 66, 82–84, 87, 115, 126–128]. An extensile approach also might be considered in lieu of the ilioinguinal should there be a nearby suprapubic catheter or colostomy, which presents a greater risk for infection [40].
Although the classic extended iliofemoral approach [3–5, 66, 128] is presently the preferred extensile exposure for the surgical stabilization of acetabular fractures, other extensile approaches have been described. Senegas et al [129] reported a transtrochanteric approach, which is a modification of the Ollier approach and allows limited exposure of the anterior column above the supraacetabular region. Another transtrochanteric approach that has been used in the past is a modification of the Gibson approach (see “Alternative to KL: The Modified Gibson Approach”), which involves a straight lateral incision [2, 54, 120, 130, 131]. Finally, some authors [66, 127, 132] have described a T-shaped modification of the extended iliofemoral approach, which involves osteotomies of the iliac crest to facilitate closure and rehabilitation. Some authors [133, 134] popularized the triradiate approach. These later approaches require osteotomy of the greater trochanter that carries the additional risk of nonunion and also has been associated with an increased risk of HO [122, 124]. Perhaps blunt trauma to the gluteal muscle mass and peritrochanteric region is the most troubling finding when planning a posterior or extended approach. Contusions and abrasions over this region may herald the presence of the Morel-Lavallée lesion. The area is usually fluctuant secondary to a large hematoma and fat necrosis developing under the degloved skin and subcutaneous tissues. Despite their closed nature, they are associated with high rates of secondary bacterial contamination and must be addressed with surgical decompression, debridement, and drainage before definitive fracture care [135, 136]. Another relative contraindication for a posterior or extensile approach is the presence of a closedhead injury, which has the potential in and of itself to lead to massive HO [135–139]. In these cases, the ilioinguinal approach has been recommended if the fracture pattern permits [139].
6.1 Fracture patterns affecting primarily one column
Fracture patterns that strictly involve only the posterior elements, such as the posterior wall (type A1), posterior column (type A2), and comminuted posterior variants are best exposed through KL approach. Prone or lateral positioning is at the discretion of the surgeon. In contrast, fracture patterns that involve primarily the anterior aspect of the acetabulum, such as the anterior column (type A3), anterior wall (type A3), and anterior column with a posterior hemitransverse component (type B3) are best suited for the anterior ilioinguinal approach. Anterior column variants, which exit in the vicinity of the iliopectineal eminence, are termed “low,” as opposed to fractures with a large iliac fragment that are described as “high” [140]. In certain cases, such as with some of the “high” variants, a more limited exposure, such as with the standard iliofemoral (Smith-Petersen) approach [20] or its modification [141] might suffice. The choice of the proper approach for more complex fracture patterns may not be as straightforward.
6.2 Transverse fracture patterns
In the case of the pure transverse (type B1) and the T-type (type B2) fractures, the choice of the optimal approach depends on the column with the greatest involvement, direction of rotation and displacement, height of the fracture relative to the femoral head, obliquity of the transverse fracture, presence of impaction, and status of the acetabular walls. The degree of displacement and extent of wall involvement is best seen on CT ( Fig 2.7-1 , Fig 2.7-2 ).
If the anterior column has the greatest displacement after preoperative assessment, then the ilioinguinal is the approach of choice. However, more commonly the posterior column demonstrates the major displacement and rotation.

In these cases, particularly with an infratectal or juxtatectal fracture, KL approach should be used. The presence of an associated posterior wall fracture also mandates a posterior or extensile approach. Among the most difficult fractures are the high transverse (transtectal) and T-type fracture patterns with involvement of the weight-bearing dome. These variants often require an extensile approach or a combined anterior and posterior exposure in order to gain adequate access to the roof of the acetabulum to facilitate anatomical restoration of the articular surface.
One study [142] provided some guidelines when determining whether the fracture should be approached from either KL or an extensile approach. The authors noted that 80% of transverse plus posterior wall fracture patterns were amenable to the former but that the presence of two of the following three conditions mitigated more for an extensile approach: (1) transtectal transverse fractures because the anterior displacement in these cases may be difficult to control from the posterior aspect of the pelvis and precise reduction of the roof is imperative; (2) associated symphysis disruption and/or contralateral pubic rami fractures as the “hinge reduction” mechanism for the ischiopubic segment is lost; and (3) the presence of an extended posterior wall pattern, where posterior visualization gives no indication of the quality of the reduction of the transverse component of the fracture. (In such cases the surgeon must look to other areas, such as the articular surface or anterior column cortex.)
Clearly, the novice pelvic surgeon should not attempt a single nonextensile approach for complex transverse and T-type fracture patterns. In cases when the surgeon is uncertain regarding his/her abilities to reduce such fractures through a single approach but wishes to proceed in such fashion, the patient should be prepared and draped in the floppy lateral position [52, 86, 114–116, 128]. This will permit the use of a combined anterior and posterior approach, if necessary. If a combined approach is planned or should the anterior column reduction be found to be impossible through KL approach, the posterior column can be reduced and stabilized from the lateral decubitus position. Following this, the patient is turned to the semisupine position to permit anterior column reduction and fixation via the ilioinguinal, iliofemoral, or other anterior approach. The disadvantage of using combined approaches in the floppy lateral position is that visualization of the fracture pattern from either approach is suboptimal. An alternative strategy is to extend an anterior limb from the midportion of the KL incision to the anterior superior iliac spine, effectively converting the approach to the extensile triradiate approach.
Finally, a simple alternative for difficult fractures, such as those with a split into the superior weight-bearing surface of the joint, is to perform a supplemental greater trochanteric osteotomy. This may facilitate the exposure without resorting to a combined or extensile approach. Removal of the greater trochanter will improve visualization of the posterior column and the superior and posterior aspects of the acetabulum, allow palpation of the anterior column, and lessen the traction on the superior gluteal vessels and nerve [20, 83, 143–147]. For T-type or transverse fractures with posterior and superior wall involvement, this technique also allows palpation and visualization of the anterior portion of the fracture.
6.3 Both-column fractures
Associated both-column (type C) fractures are the most impressive for their involvement and displacement of both the anterior and posterior columns, and their complete dissociation from the intact ilium and axial skeleton. In most cases, particularly when the posterior column is a single fragment that can be reduced anteriorly from within the pelvis, an ilioinguinal approach is in order. However, Matta [148] has warned that a clear distinction must be drawn between reduction of both-column fractures and reduction of T-shaped fractures through the ilioinguinal approach, as the latter can be difficult. This is because with both-column fractures, the joint capsule and acetabular labrum often remain firmly attached to both the anterior and posterior column fragments, allowing their reduction from within the pelvis by hinging them on their capsular attachments. In a T-shaped fracture, the posterior column is typically torn away from its capsular attachments and therefore cannot be easily hinged back into place.

Although the ilioinguinal is frequently the approach of choice, both-column fractures require excellent surgical access to the entire ilium, often necessitating an extensile approach such as the extended iliofemoral approach (or one of its modifications) or combined anterior and posterior approaches. These alternatives are generally preferred when patients are taken to the operating room late or when there are complex fracture patterns that extend into the sacroiliac joint (type C3), involve the weight-bearing dome, have fragmentation of the posterior column, or are associated with a posterior wall fragment large enough to require surgical stabilization. Because extensile exposures are associated with the highest incidence of HO and postoperative morbidity [5, 32, 66, 122, 125–127, 134, 149, 150], some surgeons have promoted the use of a simultaneous or sequential anterior and posterior approach to treat complex fracture patterns that involve displacement of both anterior and posterior structures [52, 84, 86, 115, 128, 151].
6.4 Operating room preparation
General, regional, or combinations—these are the types of anesthesia advocated and depend on many factors, including the institution, patient factors, length of the procedure, and the desire or need for intraoperative nerve monitoring. Obviously, regional is most preferable (not only intraoperatively but also for postoperative pain management) but requires dedicated and involved anesthesiologists as part of the team. The addition of epidural catheterization may also be beneficial because of reduced inhalation anesthetic requirement [10], reduced blood loss, and improved postoperative pain relief. However, while epidural anesthesia combined with general anesthesia has been shown to reduce hospital length of stay, improve postoperative pain control, reduce time to mobilization, and reduce intraoperative blood loss in arthroplasty patients, its benefits have been questioned by some with respect to treating a patient with an acetabular fracture. Strauss et al [152] found that the addition of epidural anesthesia added an average of 19 minutes to the anesthesia time (P = .01) but did not improve length of stay, pain scores, or time to rehabilitation. One benefit was a decreased amount of blood loss, but only 100 mL on average, which the authors noted was of unlikely clinical significance.
Because of the magnitude of the operative procedure, prophylactic antibiotics are used in all cases. The preferred drug is cephalosporin given intravenously immediately before surgery. A Foley catheter is placed in the patient’s bladder and vascular access is obtained with two large-bore intravenous catheters. Patients with advanced age or significant medical conditions may require placement of an additional arterial or central line. Although bleeding should not be profuse, the possibility of major hemorrhage is real; therefore, 4–6 units of blood should be available during the operative procedure. We also routinely use an intraoperative cell saver to minimize patient exposure to homologous blood. This permits recycling of about 20–30% of the effective blood loss [135]. While many surgeons believe that intraoperative red blood cell salvage is useful when excessive blood loss is expected, its routine use has been questioned by others. Scannell et al [153] used this technique in 32% of their 186 patients undergoing surgical stabilization of an acetabular fracture. The average volume of blood autotransfused was 345 mL. No differences were observed in the rates (58.3% vs 48%, P =.1883) or the mean volumes (770 vs 518 mL, P = .0537) of intraoperative and postoperative allogeneic blood transfusions between the salvage and nonsalvage groups. Not surprisingly, the total blood-related charges for the blood salvage group were significantly higher ($1958 vs $694, P = .0001).
With respect to positioning, the patient is placed on a radiolucent operating table that allows intraoperative traction and image intensifier. The selection of the type of table to be used is controversial and based on the surgeon’s preference. The exact setup is dictated by the surgeon’s planned exposure: prone, supine, or lateral. All bony prominences are well padded and the patient is supported on a bean bag, or gel or foam rolls. With posterior and extensile approaches, the sciatic nerve is in danger and constant vigilance and protection of the nerve is mandatory. Protection is afforded by the maintenance of knee flexion and hip extension throughout the procedure because this position lessens tension on the sciatic nerve [5, 154].
When available, intraoperative sciatic nerve monitoring using both somatosensory evoked potentials (SSEPs) [53, 93, 95, 155] and EMG [89, 156] has been shown to afford a degree of protective surveillance. Likely because of its ability to provide “real time” information, the latter has been shown to be more sensitive and specific than the former [89, 156]. However, no EMG signal is produced in the absence of nerve irritation. In contrast, the advantage of SSEP is that a monitored signal represents intact nerve function. While SSEP and EMG can be helpful, particularly to the less experienced surgeon, some have questioned their value. Two retrospective studies [157, 158] by experienced pelvic surgeons have yielded results similar to those reported in unmonitored patients as well as a high rate of false-negative readings when using SSEPs [157]. Haidukewych et al [157] found that the use of intraoperative monitoring did not decrease their rate of iatrogenic sciatic palsy. They compared 140 unmonitored acetabular fracture surgeries with 112 monitored procedures. Traumatic nerve palsies were present in 11(7.9%) of 140 unmonitored patients and 13 (11.6%) of 112 monitored patients (P = .314). There were 14 (5.6%) iatrogenic sciatic nerve palsies in 252 cases: 4 (2.9%) in the unmonitored group and 10 (8.9%) in the monitored group (P = .037). Interestingly, iatrogenic sciatic nerve injury was more common for ilioinguinal approaches in both groups. Also, for seven of the ten iatrogenic palsies in the monitored group, the intraoperative monitoring had been normal. The addition of EMG to SSEP monitoring, however, reduced the rate of iatrogenic injuries from 11.8–2.8% (P = .164).
Although controversial, these techniques should still be considered especially in patients at risk of developing an iatrogenic sciatic nerve injury; that is, those already demonstrating preoperative nerve compromise and those with a fracture pattern that includes a posterior column or wall fracture. When used, we prepare the entire extremity free and insert sterile subdermal electrodes. The sensory electrodes are inserted adjacent to the common peroneal and posterior tibial nerves and the motor adjacent to the tibialis anterior, peroneus longus, and abductor hallicus.
7 Specific approaches
Video 2.7-1 and Video 2.7-2 demonstrate the posterior Kocher-Langenbeck approach.
7.1 Posterior Kocher-Langenbeck approach
7.1.1 History
In 1958, Judet and Lagrange [2] combined the benefits of the Kocher (1907) and Langenbeck (1874) approaches to the hip to gain better access to the posterior column of the acetabulum through the greater and lesser sciatic notches ( Fig 2.7-3 ).
7.1.2 Indications
The posterior approach is recommended for isolated posterior wall fractures and isolated fractures of the posterior column. In experienced hands and with proper preoperative planning, it may also be used for transverse or T-type fractures of the infratectal or juxtatectal variety, especially those with associated posterior wall involvement.
7.1.3 Access
This approach ( Fig 2.7-3 ) provides direct access to the retroacetabular surface of the innominate bone (posterior column) from the ischium to the greater sciatic notch, including visualization of the entire posterior wall of the acetabulum. Indirect access to the quadrilateral surface is possible by palpation through the greater and lesser sciatic notches, allowing assessment after the reduction of fractures involving the quadrilateral plate and pelvic brim (anterior column). The greater sciatic notch also provides a window for the placement of specially designed clamps to manipulate and reduce these fractures.


7.1.4 Position
The patient is positioned either in the lateral decubitus position or prone on pillows or bolsters. The distinction between these two different positions is dependent on the fracture pattern or personality. The former simplifies intraoperative management, particularly for the anesthesia team, and is used primarily for posterior wall (type A1) and simple posterior column (type A2) fractures. In this position, the weight of the leg often hinders the reduction of transverse (type B1) fractures, thus mitigating in favor of prone positioning. One study [159] has noted a trend toward a greater degree of residual fracture displacement of transversely oriented fractures treated by the KL approach with the patient in the lateral position compared with those positioned prone. However, no significant differences were observed in operative time, estimated blood loss, or perioperative complications between the two groups. The hip is extended slightly and the knee flexed 90° throughout the procedure to minimize the incidence of iatrogenic sciatic nerve injury ( Fig 2.7-4 ).

7.1.5 Advantages
This approach is familiar to most surgeons who perform hip reconstruction surgery or unipolar or THA. Muscle dissection is minimal, as is blood loss, and exposure is adequate for both the posterior column and posterior wall.

7.1.6 Disadvantages and dangers
Superior gluteal neurovascular bundle
One major limitation of the KL approach is that the superior gluteal neurovascular bundle limits access to the superior iliac wing and is at risk during exposure of the greater sciatic notch and sciatic buttress. Therefore, the exposure is limited, especially if the transverse limb extends into the area of the greater sciatic notch [20]. Injury to the bundle can result from severe displacement of the sciatic notch because of high transverse fractures with marked medial rotation, or from an iatrogenic insult during surgery [10, 160, 161]. Letournel and Judet [5] reported an incidence of 3.5% in their series. The neurovascular bundle is at greatest risk during exposure of the greater sciatic notch and must be protected from undue traction or damage by carefully placed retractors. Furthermore, the surgical team must use extreme caution when applying traction to the gluteus medius to better visualize the lateral wall of the ilium because this act may tear the artery, with disastrous consequences, or stretch the nerve, causing permanent paralysis of the hip abductors.
Vascular injury can occur either during the exposure or fracture reduction [5, 10, 160]. Control of bleeding is crucial in this situation. Initially, packing of the area may provide hemostasis. Failing this, ligation of the bleeding vessel must be performed. The surgeon must avoid haphazardly placed vascular clips because the proximity of the superior gluteal nerve places it at risk of accidental ligation with disastrous results for the patient. Should a bleeding superior gluteal vessel retract into the pelvis in the midst of a posterior approach, an osteotomy of the sciatic notch can be performed to identify and control the bleeding vessel.
Sciatic nerve
When the KL approach is used, the sciatic nerve is always in danger and therefore must be protected at all times ( Fig 2.7-5 ). To this end, the knee joint must be flexed and the hip extended throughout the procedure, and the nerve protected by the short external rotator muscles by way of a tagged suture in the conjoint tendon. The first assistant must maintain a constant watch to ensure that any retraction of the nerve is gentle. Special retractors inserted into the greater or lesser sciatic notch with a small hook may be useful but constant vigilance is still required.
Although controversial, as discussed above [89, 156–158], intraoperative sciatic nerve monitoring is still preferred by many surgeons as it can detect sciatic nerve compromise through intraoperative monitoring of EMG activity (immediately) or via significant unilateral changes in amplitude and latency of the SSEPs. If nerve compromise is detected, then the surgical team must promptly respond by releasing traction and removing any retractors that have been placed against the nerve until the EMG activity ceases or the potentials return to baseline.
Pudendal nerve
The pudendal nerve is at risk as it exits the pelvis through the greater sciatic notch and reenters through the lesser sciatic notch. It can be injured through vigorous dissection or poorly placed retractors around the ischial spine. It also can be injured through excessive traction on a fracture table at the level of the perineal post.
Medial femoral circumflex artery
The medial femoral circumflex artery is at risk during exposure of the posterior column. Its branches are buried in the muscle of the quadratus femoris ( Fig 2.7-6 ) and can be injured if the quadratus femoris muscle is released at its insertion on the femur. The vessel emerges between the quadratus femoris muscle and obturator externus adjacent to the femur where it is at maximal risk.
Heterotopic ossification
This complication occurs after all approaches to the outer aspect of the ilium with rates ranging from 18–90% [5, 25, 32, 122, 124, 162–164]. It is frequently noted along the gluteus minimus and the debridement of any necrotic muscle in this location may decrease the risk of its occurrence [166].
Hip abductor weakness
Posterior approaches had been associated with a significant loss of hip abductor strength, possibly because of careless dissection of the gluteus maximus muscle and damage to branches of the superior gluteal nerve [166–168]. Dickinson et al [166] noted that posterior approaches were associated with a 50% or greater loss of hip abductor strength at an average 21-month follow-up, with less than half of the patients demonstrating a normal gait and seven of 22 having a residual Trendelenburg gait.
In contrast, Borrelli et al [167], although still observing that poor functional outcome was associated with muscle weakness, noted that normal muscle strength could be regained in some patients and that most demonstrated only minor changes in gait at follow-up. Building on this earlier work, in two reports Borrelli et al [60, 169] compared 15 patients with a displaced acetabular fracture treated via a posterior KL approach, to similar group treated via an anterior ilioinguinal approach. Hip muscle strength was evaluated and correlated with functional outcome using the Musculoskeletal


Function Assessment (MFA) questionnaire [60]. At an average follow-up of 44 months, patients treated via the posterior (KL) approach had an 8% overall strength deficit, including an extension strength deficit of 4%, an abduction deficit of 20%, an adduction deficit of 0%, and a flexion deficit of 7% [60]. In both studies, a relationship was noted between the hip extension/flexion work and maximum torque and hip adduction work and maximum torque and MFA score and that the greater the muscle strength recovery by the patient, the greater their functional outcome. The authors [169] therefore postulated that to maximize the functional outcome of patients after a fracture of the acetabulum that particular attention is paid to postoperative strengthening protocols. Taking their research a step further, they next correlated gait with functional outcome observing that several of the limb kinematics for the affected and the unaffected limbs were different when patients treated by an anterior surgical approach were compared with those treated by a posterior approach as well as able-bodied cohorts. When gait was compared with MFA scores, worsening function correlated with decreased kinematics and stride length. A multivariate regression analysis indicated that both hip rotation (which was associated with hip strength) and hip adductor work strength were important predictors of final MFA scores. This further supported the authors’ initial hypothesis that maximizing hip muscle strength may improve gait, and improvement in hip muscle strength and gait is likely to improve functional outcome as measured by the MFA. Of interest was that in each of these later studies [60, 169] both surgical approaches produced the same gait outcomes, suggesting that gait changes after injury and treatment are related to factors other than the surgical approach.

8 Surgical technique
Prior to the surgical incision, all bony landmarks are outlined with a sterile marking pen, including the posterior superior iliac spine, greater trochanter, and shaft of the femur. The incision for the KL approach is then centered over the posterior half of the greater trochanter. It begins 5 cm distal and lateral to the posterior superior iliac spine, curves over the tip of the greater trochanter, and travels along the lateral aspect of the femoral shaft for approximately 8 cm, ending just distal to the insertion of the gluteus maximus tendon ( Fig 2.7-7 ).
The iliotibial band then is incised up to the greater trochanter until the fascia overlying the gluteus maximus muscle is encountered. This fascia then is incised and the underlying muscle gently split in line with its fibers by blunt dissection, with two fingers aiming toward the posterior superior iliac spine ( Fig 2.7-8 ). If the gluteus maximus muscle is divided too far medially, the inferior gluteal neurovascular bundle may be compromised. Therefore, in order not denervate a significant portion of hip abductor muscle, the muscle should not be split proximal to the first neurovascular pedicle. Next, the trochanteric bursa is incised and the gluteus maximus tendon partially released (if desired) at the level of its insertion into the femur to decrease tension. At this point, it is imperative to identify and isolate the sciatic nerve, which can be consistently located along the medial aspect of the quadratus femoris muscle ( Fig 2.7-5 ). Fractures of the posterior wall or column of the acetabulum may have significant associated soft-tissue injuries, such as avulsion of the tendon of the piriformis muscle that can distort normal anatomy and place the sciatic nerve at risk for an iatrogenic injury. Once the sciatic nerve has been identified, it should be followed to its pelvic exit at the greater sciatic notch. After identifying the sciatic nerve, the short external rotators are visualized and placed on stretch by gentle internal rotation of the hip.

Exposure of the posterior column of the acetabulum is performed in a stepwise manner from the greater sciatic notch to the ischial tuberosity, avoiding injury to the sciatic nerve. The piriformis and the conjoined tendons of the obturator internus and gemelli (superior and inferior) muscles should each be isolated, tagged, released, and reflected from their femoral insertions ( Fig 2.7-6 ). It is at this junction of the procedure that an understanding of the architecture of the medial femoral circumflex artery is crucial. As the course of its ascending branch is constant in this area [170], the critical blood supply to the femoral head can be preserved by protecting the quadratus femoris muscle and releasing the external rotators a minimum of 1.5–2 cm from their insertions ( Fig 2.7-6 ; see also Fig 2.7-52 and Fig 2.7-53 ).
Once the piriformis muscle has been released, it is retracted toward the sciatic notch to gain exposure of the superior aspect of the posterior column of the acetabulum. Additional exposure of this area is provided by a Hohmann retractor inserted into the ilium under the tendon of the gluteus medius muscle (some careful stripping of the minimus may also be required). However, caution also should be taken to identify and protect the superior gluteal neurovascular bundle as it exits the greater sciatic notch in the region of the sciatic buttress. Excess traction on the bundle from too vigorous a dissection, excessive retraction on the hip abductors, or poorly placed retractors in this area may tear the artery or stretch the nerve, leading to disastrous consequences ( Fig 2.7-6 ).
Retraction of the conjoined tendons of the obturator internus and gemelli allows elevation of the obturator internus muscle by blunt dissection, exposing its bursa and the posterior column of the acetabulum ( Fig 2.7-6 ). After release of the bursa, the obturator internus muscle is then followed into the lesser sciatic notch where a blunt curved Hohmann or sciatic nerve retractor is carefully inserted. The dissection around the area of the ischial spine must be meticulous because the pudendal neurovascular bundle is at risk as it exits the pelvis through the greater sciatic notch and reenters through the lesser sciatic notch. Retraction of the conjoined obturator internus tendon gives access to the lesser sciatic notch and affords a measure of protection to the sciatic nerve and pudendal neurovascular structures, which cross over (posterior to) the tendon.
In contrast, although retraction of the piriformis tendon provides access to the greater sciatic notch, it does not protect the sciatic nerve that exits the notch deep (anterior) to the tendon. Although careful placement of blunt Hohmann retractors in the lesser and greater sciatic notches provides a clear exposure of the entire retroacetabular surface, the assistant must be vigilant regarding the sciatic nerve. This requires the maintenance of minimal and only intermittent tension, while balancing the protective soft-tissue layer of the tendon of the obturator internus between the retractor and the nerve. For complex fracture patterns, dissection should be performed to allow placement of a finger through the greater sciatic notch to palpate the medial fracture line along the quadrilateral surface. This may require release of the sacrospinous ligament or osteotomy of the ischial spine ( Fig 2.7-9 ). If further exposure of the posteroinferior aspect of the acetabulum is required, then the quadratus femoris muscle can be released from its pelvic origin along the ischium but not from its femoral insertion where the medial femoral circumflex artery is at risk. The bursa of the hamstrings overlying the ischial tuberosity can be cleared with an elevator to expose the tendonous origin of the hamstring muscles. In rare instances, when access to the superior weight-bearing surface of the acetabulum is necessary (high transtectal transverse or T-type fractures), osteotomy of the greater trochanter can be considered.
At this point, the entire posterior column can be visualized from the greater sciatic notch to the ischial tuberosity. The posterior articular surface of the femoral head usually can be easily visualized through the fractured posterior wall or column and a rent in the capsule is almost always seen. The hip capsule throughout the exposure should be preserved to as great an extent as possible to preserve the blood supply to the femoral head. Posterior wall and column fragments are identified and their edges debrided sharply. Intraarticular fragments can be removed at this point by distracting the hip joint using femoral traction with either a fracture table ( Fig 2.7-4 ) or a femoral distractor ( Fig 2.7-10 ). By internal rotation, the hip may be “redislocated” and washed of all small fragments of articular cartilage and bone [20].
After reduction of the hip joint and further exposure of the entire posterior column from the ischial spine to the greater sciatic notch, the fracture may be reduced and stabilized in an appropriate manner. Techniques from the posterior approach will depend on the specific fracture pattern, which is usually a posterior wall, posterior column, transverse, or T-type. Posterior wall (type A1) fractures are routinely thought of as simple fractures to treat. However, this belief is incorrect, as their reconstruction is often complicated by significant comminution or marginal impaction ( Fig 2.7-11 , Fig 2.7-12 ) [18, 19, 39, 50, 170, 171]. These two entities make posterior wall fractures more difficult to treat, leading to among the poorest outcomes for all fractures of the acetabulum if ignored, especially when an associated posterior column or transverse fracture also is present [5, 18, 19, 32, 39, 172]. Marginal impaction of the articular surface is relatively common after a fracture of the posterior wall, occurring in 16–47% of cases, and usually associated with a posterior hip dislocation [19, 39, 50, 170, 171]. As the femoral head dislocates, it not only fractures the posterior wall but also implodes the articular surface. Impacted fragments are readily identified on the preoperative CT scan and when exposed at the time of surgery are usually noted to be rotated 90° so that the surgeon looks directly at the articular surface ( Fig 2.7-11b and d). It is of paramount importance that large fragments be anatomically reduced at the time of surgery to maintain joint congruity.

After cleaning the joint of debris and stabilizing an associated column or transverse fracture, traction is released and the femoral head used as a template for the articular reduction. The medial aspect of each cortical wall fragment should be cleared of enough soft tissue to permit visualization of its reduction, while retaining as much of its capsular attachments as possible to preserve its blood supply. Extremely small and avascular fragments should be discarded. Next, any impacted articular fragments are gently elevated into a position congruent with the femoral head. These fragments must be carefully derotated by gently teasing them up with an elevator until they are congruous with the femoral head ( Fig 2.7-12b ). It is critical to leave as much metaphyseal bone attached to these chondral fragments as possible or it may be impossible to buttress their stability in their reduced position.


The reduction of marginally impacted fragments invariably produces a metaphyseal defect underlying the articular fragment(s), which is generally filled with autogenous cancellous bone graft. In most instances, this is easily obtained through a small window in the greater trochanter and used to buttress the reduced marginal fragments ( Fig 2.7-12c ). For large defects, structural allograft croutons can supplement the autograft. Another option is the use of bone graft substitutes, such as calcium-phosphate cement, which has been shown to partially restore normal joint-loading parameters [173]. It is extremely difficult to get any form of internal fixation into marginally impacted fragments, although focally placed bioabsorbable pins or small screws [174] can be considered. If the latter is used, they will be covered over by the reduced wall fragment(s) and thus present potential problems with their removal, should further surgery become necessary. Therefore, the maintenance of the position of reduced marginally impacted fragments is generally dependent on the bone graft or other suitable material and replacement of the main wall fragment on top. However, these marginally impacted fragments may be avascular and collapse in the postoperative period. The prognosis for the joint depends on how large these fragments are and whether good stability has been retained.
Next, the wall fragments are reduced and held in place by the straight ball spike pusher, followed by provisional fixation with K-wires. A reconstruction plate 3.5 is applied in the buttress mode over the reduced posterior wall and anchored to the ilium proximally and ischium distally. Underbending of this plate, in relation to the posterior wall, will aid in reduction of the construct and compress the fracture ( Fig 2.7-13 ). To best prevent fracture displacement, one or more lag screws (through or outside the plate) should be placed across the posterior wall into the posterior column. When the posterior wall is comminuted, it is not possible to restore all small articular fragments with individual lag screws. In this situation, one can use spring hook plates (either prefabricated plates or short one-third tubular plates with their tips cut off through a hole and the newly created prongs bent downward to create small hooks) [7, 23, 50, 140, 175–177]. These plates are affixed in a loaded fashion underneath the posterior wall buttress plate more medially, but with the spring-loaded lateral hooks providing a buttressing effect to the comminuted posterior wall ( Fig 2.7-14 ). Other options to buttress thin, small, or comminuted posterior wall fragments and thereby serve as cortical substitution include modified distal radius T-plates [178] or cervical H-plates [179].
Fractures involving the posterior column (type A2) generally result in posterior-medial displacement and internal rotation of the posterior column, as viewed from a posterior aspect. After debridement of the fracture and removal of all comminuted fracture fragments and/or organizing hematoma, posterior column fracture reduction is accomplished by correcting the rotation with a Schanz screw in the ischium and the use of a pelvic reduction clamp in the greater sciatic notch to correct the medial displacement ( Fig 2.7-15 ). The gluteal neurovascular bundle can be damaged during this maneuver, and must be monitored. Alternatively, the reduction is accomplished by screw-holding clamp applied to 3.5 mm or 4.5 mm bicortical screws (depending on the clamp) inserted into each of the main column fragments. Care must be taken to position the screws away from the area of eventual plate placement. These clamps allow distraction, debridement, and compression of the fracture. After reduction and provisional fixation with a K-wire, direct visual inspection of the posterior fracture line best assesses displacement of the column, whereas rotation is best judged by digital palpation through the greater and lesser sciatic notches. A smooth quadrilateral surface is usually indicative of correct rotational alignment. When an accurate reduction has been confirmed, a reconstruction plate 3.5 is applied from the ischium to the ilium. A lag screw across the column fracture prevents redisplacement. If combined with a posterior wall fracture, the posterior column reduction should be addressed first followed by double plating, one for the column and one for the wall, as necessary.
Transverse (type B1) fractures require techniques similar to those used for posterior column fractures. However, although the retroacetabular fracture line in a transverse fracture may appear much the same as in the posterior column fracture, in these fracture types it is necessary to reduce not only the posterior column portion of the transverse fracture but also the anterior displacement and malrotation. Displacement can be controlled using the two-screw technique ( Fig 2.7-15c ) with a screw-holding reduction clamp, as described for the posterior column fracture. The surgeon can use this clamp for distraction and debridement of the fracture line, all the way to the anterior column, and subsequently for manipulation and temporary reduction of the transverse fracture component. Rotation also can be controlled with either a Schanz pin in the ischium or pelvic reduction clamp with pointed ball tips placed into the sciatic notch. An elegant maneuver is to first secure a plate into one of the fracture fragments, and then to use the plate as a reduction tool.




After provisional fixation and inspection, stabilization is obtained with a reconstruction plate 3.5 applied to the medial border of the retroacetabular surface and lag screw(s). This plate should be overcontoured to achieve compression of the anterior column segments as it is tightened down to the posterior column ( Fig 2.7-16 ). Under contouring, as performed for posterior wall fractures, actually leads to distraction of the anterior column in transverse fractures. To prevent displacement of the anterior column, a posteroanterior column lag screw is required for all transverse fracture types. This screw usually can be placed through the posterior buttress plate and must be oriented parallel to the quadrilateral surface to avoid joint penetration. Finger palpation along the quadrilateral plate helps in assuring correct position for the screw. This should be checked with image intensification on the operating table using multiple views, especially looking at the obturator oblique to assess the screw’s position in the anterior column and to ensure its extraarticular placement.
T-shaped (type B2) fractures are among the most difficult of all fracture types to manage. It is a complex variant of the transverse fracture pattern, in which the inferior ischiopubic segment has been separated into anterior and posterior fragments by a vertical stem component. Because of this, it is impossible to control the separate anterior column fragment by direct manipulation of the posterior column fragment(s) when working through a posterior approach, except possibly by ligamentotaxis through nondisrupted joint capsule. Therefore, successful fixation of this fracture through a posterior approach is dependent on the surgeon’s ability to obtain an indirect reduction of the anterior column and to confirm an accurate reduction by palpation of the anterior column and stem component through the greater sciatic notch. To achieve such a reduction, the surgeon must be familiar with the placement of instruments into the sciatic notch, and their use to manipulate the anterior column fragment after the provisional stabilization of the posterior column. For example, a small bone hook or pusher, gently introduced along the quadrilateral plate, can be used to derotate and pull the displaced portion of the anterior column posteriorly. This reduces this segment into the acute angle between the intact proximal anterior column and the reconstructed posterior column. Once an acceptable reduction has been accomplished, definitive fixation is achieved by the application of a posterior buttress plate and posterior to anterior lag screws as in transverse fractures. Care must be taken that implants for provisional or definitive fixation of one column do not cross into the opposite column, hindering or preventing its reduction [54], and to avoid intraarticular or intrapelvic placement of the lag screws. The wound is closed in a meticulous fashion following completion of the operative procedure. The tendons of the piriformis and obturator internus muscles are reattached at the greater trochanter along with the remainder of the short external rotators. If a release of the gluteus maximus insertion has been required, this too is repaired at its insertion along the femur. Two suction drains are placed over the external rotators followed by closure of the iliotibial band and the fascia overlying the gluteus maximus muscle. A subcutaneous suction drain is inserted and the skin meticulously closed.

8.1 Modified limited exposure using Kocher-Langenbeck
Several authors have proposed more limited versions of KL including a gluteus maximus–splitting approach [180] or working through limited windows by not releasing the conjoint and piriformis tendons [168, 181]. Josten and Trabold [168] compared a group of nine patients who underwent such a limited approach to a group of nine who underwent the standard KL and found hip muscle strength and gait to be improved compared to the latter (not statistically significant), with a shorter operative time and similar articular reductions.
8.2 Transtrochanteric approach
8.2.1 Indications
The indications for the transtrochanteric approach are the same as those for the KL but this variation affords better visualization of higher transtectal, transverse, and T-type lesions.
8.2.2 Access
Access to the upper part of the posterior column into the greater sciatic notch is increased compared to the standard KL approach. Trochanteric osteotomy also allows visualization of the lateral aspect of the acetabular superior wall anteriorly to the anterior inferior spine of the ilium.
8.2.3 Position
This is the same as KL, with the lateral decubitus position more usual.
8.2.4 Advantages
This modification (osteotomy) extends the exposure to the anterior inferior iliac spine with improved visualization and access to the posterior column and superior aspect of the acetabulum (including the weight-bearing dome) [20, 83, 143–147]. Removal of the trochanter also removes much of the tension from the superior gluteal vessels and nerves and, thus, protects them. The standard KL approach can be extended this way at any time should increased exposure be required.
8.2.5 Disadvantages and dangers
The same neurovascular structures are at risk as in the standard KL, except that increased stripping of the gluteus medius and minimus muscles leads to a significantly higher risk for abductor weakness and/or HO [20, 124, 165, 182]. Heterotopic ossification is often noted along the gluteus minimus and the debridement of any necrotic muscle here may decrease the risk of this complication [165]. Furthermore, the unique disadvantages of this approach include the risk for nonunion of the trochanter. Also, the risk of avascular necrosis of the femoral head theoretically is increased by the potential interference with the posterior blood supply. Finally, visualization of most of the anterior column cannot be achieved except by looking into the hip joint or by palpating through the greater sciatic notch because this approach is still limited to the posterior column.
8.2.6 Surgical technique
The skin incision is identical to that for the posterior KL approach, except that the lateral portion is longer [20]. If the standard KL approach has been performed but the surgeon desires greater access, it is a simple matter to osteotomize the greater trochanter and gain access to the superior wall of the acetabulum and a portion of the anterior column ( Fig 2.7-17 ). The anterior column can be stabilized only by retrograde screw fixation (it is impossible to use a plate); however, the superior weight-bearing surface of the acetabulum will be visualized laterally, and intraarticularly by division of the capsule and traction. For this approach, initially the technique is identical to that for the posterior KL approach; then, the greater trochanter is predrilled to accept one or two screws at closure and simply is cut using a Gigli saw. The Gigli saw is much safer and avoids the risk of sawing into the femoral head. This technique as classically performed does not spare the vastus lateralis origin on the fragment and therefore requires more robust fixation. Therefore, at the time of closure, adequate fixation is obtained by two 6.5 mm cancellous lag screws (with or without tension band wires).
8.2.7 Trochanteric flip osteotomy
A preferred alternative to the classic trochanteric osteotomy above is known as the trochanteric “flip” osteotomy. It was originally described by Ganz for surgical dislocation of the hip and subsequently has been used successfully by, others [143–147]. This technique involves taking a thinner cut through the greater trochanter and leaving the origin of the vastus lateralis attached to the distal portion of the trochanter (see “Other special technical factors: trochanteric flip osteotomy with surgical dislocation of the femoral head for treatment of fractures of the acetabulum”). When using this modification, fixation can be performed with 3.5 mm screws [144, 147]. The improved blood supply and stability afforded by this technique improves the exposure and minimizes complications and may even improve results. Tannast et al [147] evaluated 54 patients with acetabular fractures treated by a trochanteric flip osteotomy at a mean follow-up of 4.4 years. An anatomical reduction had been achieved in 50 hips (93%), which was considerably higher than that seen in previous reports. There were no cases of avascular necrosis and only four patients subsequently required total hip replacement. Good to excellent results were achieved in 44 hips (81.5%) with functional outcomes better than those previously reported.

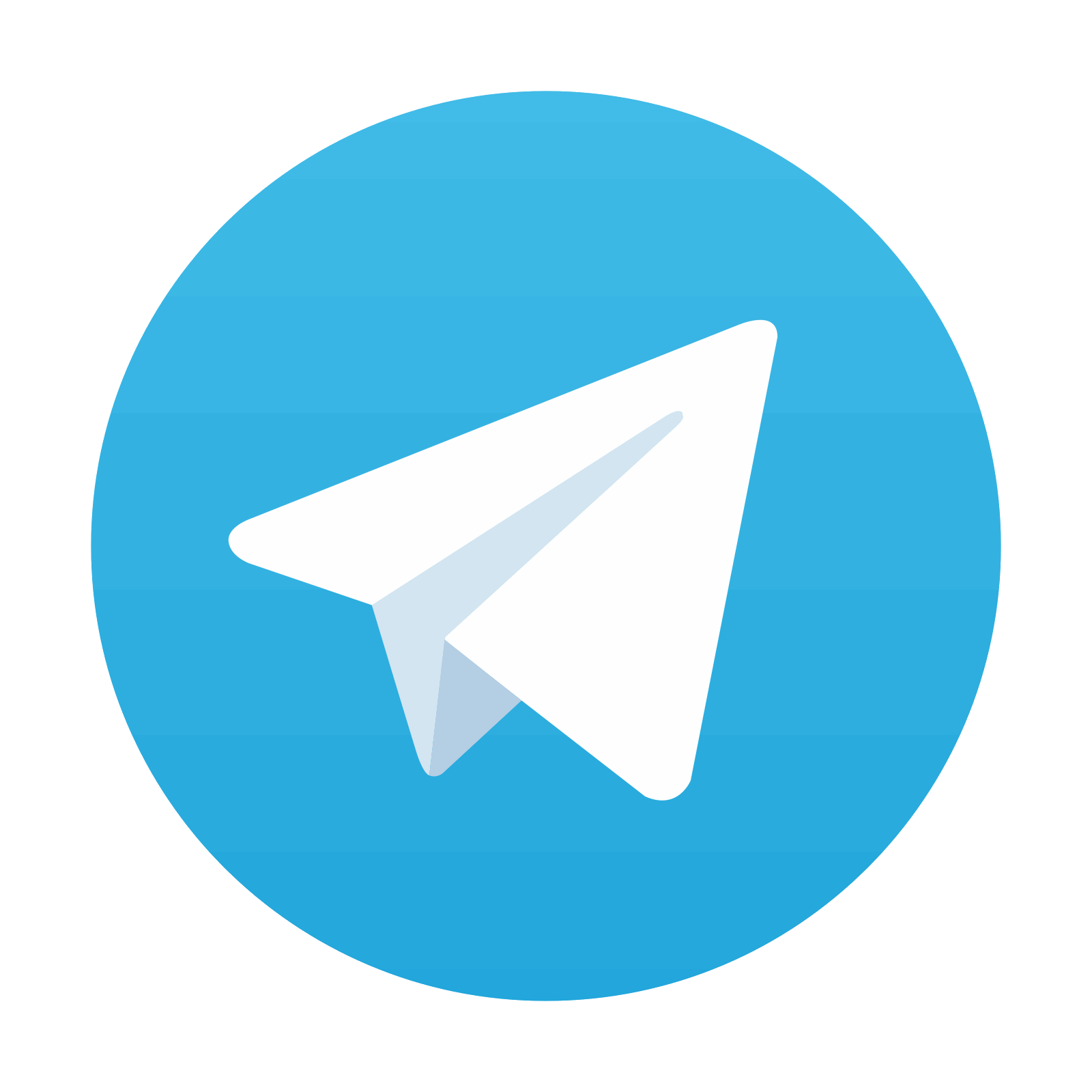
Stay updated, free articles. Join our Telegram channel

Full access? Get Clinical Tree
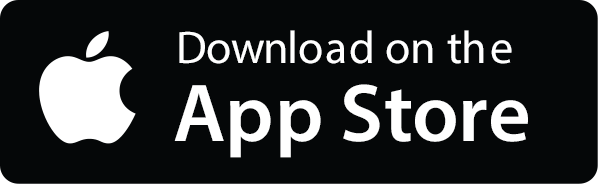
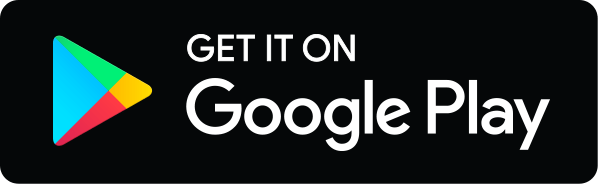
