2.4 Instrumentation(2.4.4 – 2.4.6)
2.4.4 Tension band techniques Matthew Porteous
Using the tension band principle to achieve absolute stability is a concept that may be difficult to understand.
The principle is based on the fact that any structure that is loaded asymmetrically has some areas within it under tension and others under compression. Imagine an elephant on each end of a child’s seesaw; the beam will break at the pivot. Intuition tells us that the fracture will start on the upper surface of the beam. This is the part that is under the most tension (Fig 2.4-28). Wood and steel (like bone) are weaker in tension than in compression, so this is where the point of failure occurs. We use exactly the same principle when trying to break a dry stick. We bend the stick, creating tension on the convex surface and compression on the concave surface (Fig 2.4-29). Failure starts on the tension surface and travels rapidly through the stick, from there to complete the fracture.

The same dynamics may occur if weight is placed on the top of a solid column. If the weight is placed centrally, the whole column is loaded in compression with the load equally distributed throughout the column. However, if the load is placed off to one side (asymmetrically), the side nearest the weights remains in compression but the opposite surface is under the most tension. While in the middle part of the column there is an area that is neither under tension nor compression (Fig 2.4-30).

2.4.4.1 Tension band as a plate
A similar situation arises in the human femur, which is loaded eccentrically because of the offset of the femoral head and neck to the long access of the bone. This means the lateral side of the bone is under tension and the medial side is under compression when the patient walks (Fig 2.4-31a). In a simple transverse fracture of the femur, the failure starts on the lateral side because the collagen component of bone (which resists tension) is much weaker than the mineral component (which resists compression). Indeed after a simple transverse fracture, the bone is still able to resist compression. If the fracture can be reduced and a way can be found of taking up the tension, for example a plate (Fig 2.4-31b–c), then loading the femur (eg, walking on it) will actually increase the compression at the fracture site. For this to function the medial (compression) cortex must remain intact (Fig 2.4-31d–e). It will not work if the fracture is multifragmentary.


Although a lateral plate on a transverse femoral fracture is a convenient example of a tension band and an acceptable way of managing this injury, intramedullary nails are preferred as they work equally well, cause less soft-tissue damage, and can be used for almost all femoral diaphyseal fracture patterns. The tension band principle is sometimes applied in the management of subtrochanteric femoral fractures with a blade plate (Fig 2.4-32).
Tension band fixation provides absolute stability at the fracture site, but compression is also “dynamic” in that the greater the load applied the greater the compression across the fracture. This causes confusion, but these two concepts are not mutually exclusive. When someone lifts his or her left foot off the ground, the right foot does not move (absolute stability) but the load under the foot doubles (increased compression).

2.4.4.2 Tension band wiring
The most common application of tension band in trauma surgery is the tension band wire used for managing olecranon and patella fractures.
Biomechanically the olecranon is an inverted seesaw, with the distal humerus acting as the pivot while triceps and brachialis muscles pull on each side of the proximal ulna (Fig 2.4-33a). The dorsal surface of the olecranon is therefore under tension and the ventral surface under compression. A simple transverse fracture can be held accurately, reduced with two parallel K-wires. The tension band is supplied by a figure-of-eight looped wire over the tension surface, anchored by the K-wire ends proximally, and a hole through the ulna distally. The wire is tightened equally on both sides by twisting to apply compression. Once fixed, any pull on the triceps muscle increases the dynamic compression across the fracture site (Fig 2.4-33b).
The situation in the patella is similar. This time it is the insertion of the quadriceps and patellar tendons onto the superficial surface of the patella which provides the load and the femoral condyles which acts as the pivot, setting up tension at the superficial and compression at the deep surface of the patella. Failure occurs in tension, usually caused by a fall on the front of the knee. Provided the fracture is a simple transverse one and there is no fragmentation of the deep cortex, it can be reduced over two parallel K-wires and a superficial tension band wire applied round the ends of these wires (deep to the tendons) is tightened to provide compression. The pull of the quadriceps then increases dynamic compression across the fracture as the knee flexes and extends (Fig 2.4-34).
The tension band principle can be applied in other fractures when the anatomy provides for eccentric bone loading, and the fracture is only in two parts, other sites where this applies include the medial malleolus and the base of the fifth metatarsal.
2.4.4.3 Conclusion
Tension band fixation provides absolute stability at a fracture site, although the amount of compression between the fracture fragments may vary with the load applied and is therefore considered to be dynamic. Tension bands can only be used when the compression cortex opposite the tension band is intact. If it is not, then an alternative method of fixation must be used.


2.4.5 Intramedullary techniques Merng Koon Wong
Intramedullary nail stabilization of a fracture is intended to provide relative stability at the facture site, and therefore produce conditions for fracture union by indirect healing with formation of callus. Callus implies that there is controlled movement at the fracture site under functional loading. This chapter looks at the design of intramedullary nails and some technical issues around their insertion, and considers their local and systemic physiological impact.
2.4.5.1 Nail design
The mechanical strength of an intramedullary nail depends on the design. The original Küntscher nails had a clover leaf design with an open slot which enabled the nail to grip the bone in the absence of locking bolts (Fig 2.4-35). However, the slot greatly reduced the strength and torsional stability of the nail. The introduction of locking bolts which ensure excellent mechanical linkage between nail and bone led to the development of stronger unslotted cylindrical and solid nails.

Other factors that increase a nail’s mechanical strength include the nail diameter and the thickness of the wall of a hollow nail. The material that the nail is made of is also important; steel nails are stronger but have greater rigidity than titanium ones of the same dimensions.
An intramedullary nail acts as a splint. Left unlocked it is good at resisting bending in any direction, but resistance to torsion and telescoping at the fracture site is only provided by the friction between the reduced fracture fragments and the contact between the nail and the bone above and below the fracture site. This may be enough to maintain reduction in a simple transverse fracture, but in multifragmentary fractures it is always insufficient (Fig 2.4-36). For this reason all nails should be locked by locking bolts (screws) proximally and distally to provide adequate resistance to torsional and compressive forces.

The resistance to bending deformation is a function of the working length of the intramedullary nail. The working length is defined as the distance across the fracture site between the nearest points where the nail engages the bone. In a loose-fitting nail or a multifragmentary fracture, the engagement between bone and nail is provided by the locking bolts, and the working length is the distance between those at the top and the bottom (Fig 2.4-37). With a tight-fitting nail in a simple middle-third diaphyseal fracture where the nail grips the diaphyseal bone on each side of the fracture site, the working length is much shorter. The longer the working length the more movement will occur at the fracture site when the fracture is loaded.

2.4.5.2 Surgical technique
Reaming
Today’s nails are either solid or cannulated, and both types can be inserted either reamed or unreamed. The decision to use reaming rests with the surgeon and is part of the preoperative plan.
Reaming the intramedullary canal removes irregularities and circumferential layers of bone from the endosteal surface, thus enlarging the diameter of the canal and allowing insertion of a larger nail. However, it damages the endosteal blood supply which is normally responsible for supplying the inner two-thirds of the shaft of a long bone (Fig 2.4-38). Blood supply is diverted to the periosteum to compensate for this. Reaming can also generate high pressure within the intramedullary cavity of the bone which forces debris into the venous circulation. This is believed to increase the systemic inflammatory response implicated in the pathogenesis of acute respiratory distress syndrome (ARDS) and multiple organ failure (MOF). The outcome of this is not yet fully understood. In healthy patients with single fractures the systemic effects of reaming are rarely a clinical problem. However, in severely injured patients or those with a significant lung injury, reaming should be kept to a minimum or avoided (see chapter 2.9).

Although reaming damages the endosteal blood supply to the bone, for reasons that are not fully understood, fractures treated with reamed nails heal faster than those in which unreamed nails have been used. Therefore reaming is generally recommended, unless there is a specific contraindication.
Reaming should always be performed over a guide wire placed across the fracture and is carried out in 0.5 mm increments to a diameter of 1.0 to 1.5 mm more than the nail diameter. The reamer heads should always be rotated at full speed. How much to ream is at the discretion of the surgeon, who has to balance the need for minimizing bone resection and endosteal damage against using a nail of sufficient diameter to hold the fracture until the bone has healed without the nail breaking.
Reamer heads should always be kept sharp. The use of blunt reamer heads and the prolonged reaming of hard bone can both generate considerable heat. If the temperature is permitted to rise high enough, it can cause thermal necrosis of the bone. Prolonged rotation of a reamer head which is not making progress must be avoided. Should this occur, the reamer head must be withdrawn and the flutes of the reamer cleared of bone debris while it cools down before continuing.
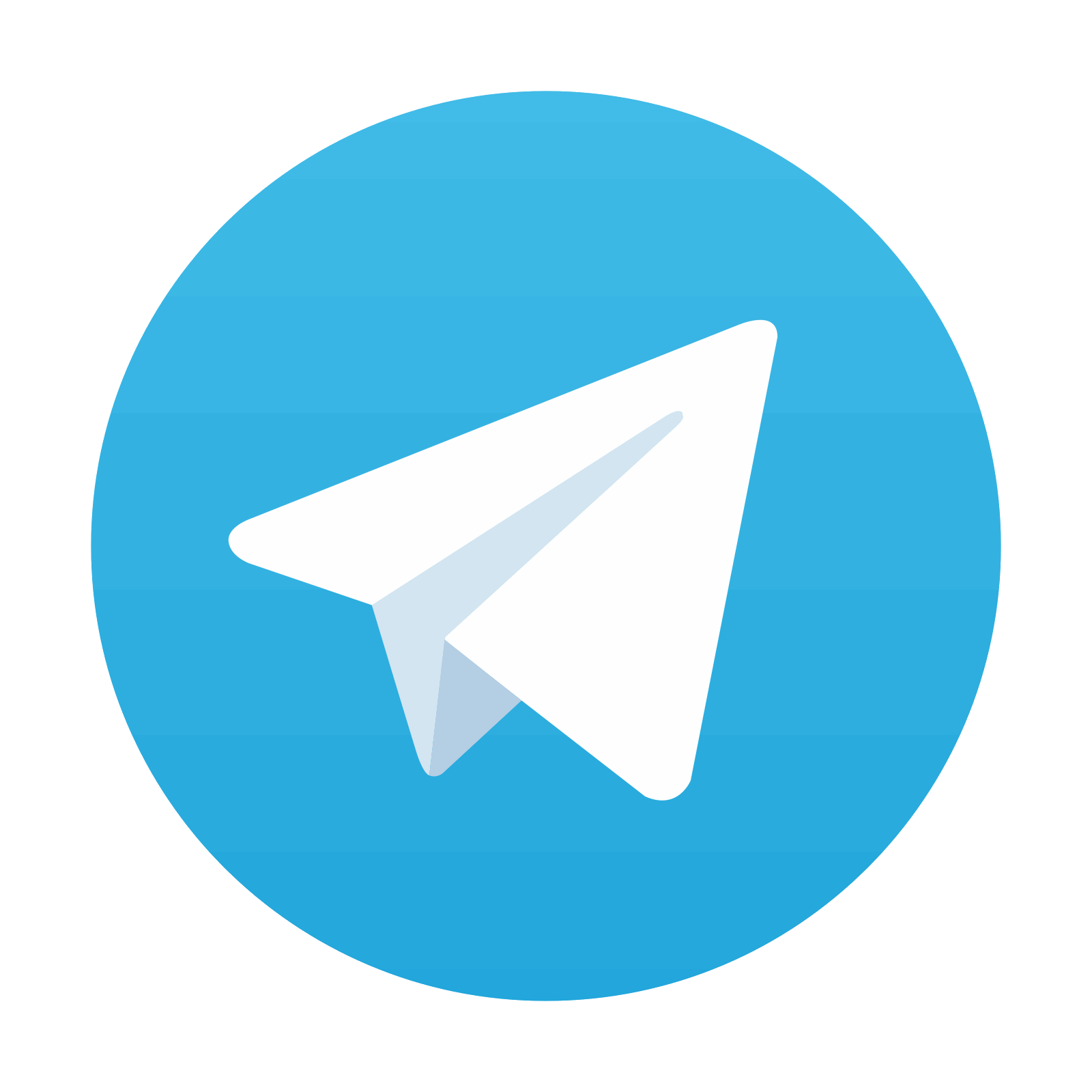
Stay updated, free articles. Join our Telegram channel

Full access? Get Clinical Tree
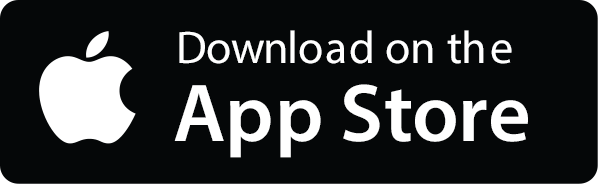
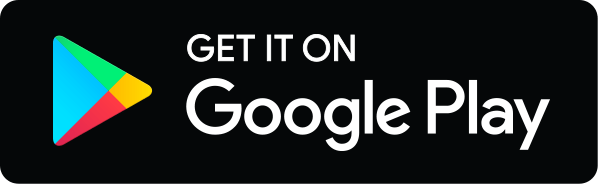
