2.4 Instrumentation (2.4.1 – 2.4.3)
Spectrum of stability Piet de Boer
How to achieve absolute stability
How to achieve relative stability
Locked internal fixators (LCPs fixed with locking head screws)
Plates and plate techniques David J Hak
Tension band techniques Matthew Porteous
Intramedullary techniques Merng Koon Wong
Extramedullary techniques Michael Baumgaertner
Authors Piet de Boer, Daniel Saris, David J Hak, Matthew Porteous, Merng Koon Wong, Michael Baumgaertner
2.4 Instrumentation
2.4.1 Spectrum of stability Piet de Boer
When a bone fractures there is always a disruption in the continuity of the bone. Complete fractures that are displaced also demonstrate instability. When the patient loads the injured limb, as for example putting weight on a fractured tibia, there is gross movement of the bone ends. This movement causes pain and may result in a delayed union or nonunion. If the instability remains uncontrolled it will almost certainly result in the fracture healing in a shortened or angulated position, such that function may not fully recover even after the bone has united. Instability may therefore cause pain, delayed or nonunion, and poor-end function due to malunion or an inability to mobilize the injured limb.
Treatment of a fracture always involves stabilization of the fracture. There is a range of stability that can be achieved with treatment, varying from gross movements on loading the fracture site to movements that can only be detected by specialized instruments. This is what is meant by the spectrum of stability. For practical use, however, surgeons define only two types of stability at a fracture site after treatment, absolute stability and relative stability.
Types of stability
Absolute stability means that when a bone is loaded with a physiological load, for example partial weight bearing with crutches, no detectable movement occurs at the fracture site. Relative stability means that there is a degree of controlled movement at the fracture site on physiological loading. Relative stability provides the amount of movement required to stimulate the natural healing process of callus formation but not so much as to involve a risk of nonunion. Both types of stability are sufficient for the patient to be able to mobilize the affected limb and adjacent joints without significant pain.
Absolute stability
The bone healing that occurs after a fracture has been fixed to achieve absolute stability is fundamentally different from the normal healing processes seen in fractures treated with relative stability. When absolute stability is obtained fractures heal by bone growing directly across the fracture site and no callus formation is seen (Fig 2.4-1). This type of healing is termed direct bone healing (sometimes called primary bone healing). Direct bone healing is a slow process which closely mimics the processes of remodeling seen following natural bone healing. Because it is slow, the fracture relies on the mechanical implant used to fix it for a much longer period than those fractures treated using a technique which stimulates the production of callus.

For bone to be able to grow directly across the fracture site, it is essential that there is no movement between the fracture ends, and the gap between them must be small. To achieve this, the fracture must be anatomically reduced and the bone ends compressed together. Direct bone healing also requires a good blood supply to the bone so it is essential to minimize periosteal stripping and soft-tissue damage around the fractured bone. Excessive soft-tissue stripping at or around the fracture site results in islands of dead bone which do not unite, regardless of the stability given to them, until revascularization occurs.
Relative stability
Treatment which achieves relative stability leads to healing with callus. This is sometimes referred to as indirect or secondary bone healing. Callus formation requires a degree of controlled movement at the fracture site and, as with absolute stability techniques, preservation of blood supply is critical to fracture healing. Anatomical reduction is not required but in diaphyseal or metaphyseal fractures the correct length, axis, and rotation of the limb must be maintained or restored to allow normal function once fracture union has occurred. Callus formation is a faster process than direct bone healing. The fracture is dependent on the implant for stability for a shorter period than when absolute stability techniques are used.
2.4.1.1 How to achieve absolute stability
Absolute stability requires anatomical reduction, compression across the fracture site, and the preservation of blood supply.
Reduction technique: An open direct technique is usually required to obtain anatomical reduction. The fracture is exposed and reduced under direct vision. Restoration of length may be made using an ancillary aid, such as a femoral distractor or external fixator, but the final reduction is usually made using pointed reduction forceps or a similar bone clamp.
Surgical approach: A formal surgical approach is usually indicated. It is possible to achieve anatomical reduction of simple fractures using minimally invasive techniques and specialized reduction clamps, but in nonspecialized practice an open surgical approach is generally indicated.
Fixation techniques: The most common way of achieving compression at a fracture site is with the use of plates or screws. The lag screw is the standard working tool used to obtain absolute stability (Fig 2.4-2). Compression at the fracture site can either be obtained using the design of the screw, ie, the partially threaded cancellous bone screw, or by over drilling the near cortex—the classic cortical lag screw (see chapter 2.4.2). The insertion of lag screws necessarily involves some soft-tissue dissection and great care must be taken not to devitalize bone fragments with the instrumentation used for the insertion of the screw. A plate is normally required to reinforce lag screw fixation of a long bone, and in these circumstances is described as a protection (or neutralization) plate. A plate of identical design can also be used in a different way to obtain compression and achieve absolute stability (see chapter 2.4.3). Specialized ring external fixators can also be used to achieve absolute stability but this technique is usually reserved for nonunions and is rarely indicated in acute trauma.

All techniques used to achieve absolute stability carry a significant risk of devitalizing bone. A good, gentle surgical technique is therefore more critical in absolute stability than in relative stability techniques.
2.4.1.2 How to achieve relative stability
Relative stability can be obtained at a fracture site by various means.
Plaster of Paris: This is still the most commonly used means of obtaining relative stability. Plaster of Paris provides stability toward bending and twisting forces but cannot prevent fracture shortening, if the fracture pattern is such that shortening tends to occur. The foremost advantage of plaster of Paris is the preservation of the soft-tissue envelope around the fracture site. The disadvantage is that plaster immobilizes the joints above and below the fracture site preventing their mobilization.
Intramedullary fixation: This is a classic splinting technique which results in relative stability being achieved at the fracture site. Intramedullary nails preserve the soft tissues around the fracture site when an indirect reduction technique is used and the fracture site is not opened. X-ray imaging during surgery with an image intensifier is essential. Intramedullary nails provide excellent stability with regard to bending forces but do not provide any stability with regard to twisting forces or shortening unless locked. Locked nails provide excellent relative stability and are the implant of choice for diaphyseal fractures of the tibia and femur (Fig 2.4-3).

External fixators: These also achieve relative stability. They can be constructed in various ways. Changing the number of pins, their site, size, and the number and position of the bars used to connect the pins can radically change the amount of stability given to any treated fracture site (Fig 2.4-4). External fixators preserve the soft-tissue envelope around the fracture site and are particularly useful when fractures are associated with extensive soft-tissue damage, ie, open fractures.
Plates and screws: These can also be used to achieve relative stability. The plate is applied to the reduced fracture and screws are inserted into the main bone fragments above and below the fracture site. No lag screws are used. An anatomical reduction is not necessary, but achieving the correct length, axis, and rotation of the bone is essential. Used in this way, the plate is known as a “bridging plate” (Fig 2.4-5).

If reduction can be obtained by indirect means, such as an external fixator, then an incision may be made above and below the fracture site, the plate is slid between the two incisions and fixed to the two main bone fragments with minimal disturbance of the soft tissues around the fracture. This technique is known as minimally invasive plate osteosynthesis (MIPO). The advantage of this technique is that it preserves the soft-tissue envelope around the fracture site. The disadvantage is that it is technically difficult to achieve and malunions are not uncommon.

2.4.1.3 Locked internal fixators (LCPs fixed with locking head screws)
The most modern design of plates allows use of screws that lock into the plate, namely locking head screws. Once this occurs the plate screw unit becomes a single piece of metal. The locking compression plate (LCP) has screw holes for both locking head screws and conventional screws (Fig 2.4-6). Like other plate types, the LCP can be used to achieve absolute or relative stability depending on how it is applied. When an LCP is used to achieve absolute stability, a lag screw can be inserted either through the plate or independent from it to achieve compression at the anatomically reduced fracture site. The plate then functions as a protection plate. Conventional or locking head screws can be used in this mode depending on the quality of the bone. To act as a compression plate, the first screws inserted have to be conventional ones (see chapter 2.4.3). Subsequent screws used to reinforce the plate’s initial grip on the bone can be conventional or locking again depending on the bone quality.

When an LCP is used to achieve relative stability, it is used as a bridging plate with conventional or locking head screws inserted into the two main fragments each side of a fracture, with minimal disruption to the soft tissues around the fracture. No lag screw is used. Locking head screws are particularly useful in osteoporotic bone and metaphyseal fractures.
Indications for absolute stability techniques
The key indication for the use of absolute stability techniques in the 21st century is in intraarticular fractures. Good joint function can only be obtained in intraarticular injuries if the articular surface of the joint is restored anatomically and the fracture fixed using an absolute stability technique. Only in this way can early active movements be obtained and the nutrition to the articular cartilage preserved during the healing phase.
Absolute stability is also indicated in fractures of the forearm, when any degree of malunion will result in loss of forearm rotation. These therefore require anatomical reduction. Absolute stability techniques are more likely to be used if the fracture patterns are simple and are also indicated in the treatment of nonunion and in osteotomies.
The major contraindication to the use of absolute stability techniques is the treatment of multifragmentary fractures. The main danger in applying absolute stability is devitalization of the bone while achieving reduction, thereby delaying or abolishing healing. The more fragments there are in a particular fracture the more lag screws need to be inserted to achieve absolute stability and the greater the chances are that the bone will be devitalized. Multifragmentary diaphyseal fractures should not be treated with absolute stability techniques.
Indications for relative stability techniques
The best indication for relative stability is the treatment of multifragmentary diaphyseal and metaphyseal fractures. If a good functional reduction is obtained, ie, correct length, axis, and rotation, rapid union occurs together with the restoration of excellent function.
Most diaphyseal fractures in the tibia and femur are treated with relative stability techniques using intramedullary nailing even if they have a simple fracture pattern.
Relative stability is contraindicated in intraarticular fractures and should not be used in the treatment of delayed union or nonunion.
2.4.1.4 Conclusion
Absolute stability is achieved when there is no movement at the fracture site on physiological loading. Healing occurs by bone growing directly across the fracture site. It requires the preservation of blood supply, an anatomical reduction, and interfragmentary compression. Absolute stability is indicated in the management of intraarticular fractures and in simple diaphyseal fractures apart from the tibia and femur.
Relative stability implies controlled movement at a fracture site, sufficient to generate callus formation while allowing painless mobilization of the limb. It requires the preservation of blood supply, a functional reduction, and the creation of controlled movement at the fracture site. Relative stability is indicated in the management of nonarticular fractures, especially multifragmentary fractures of the metaphysis and diaphysis.
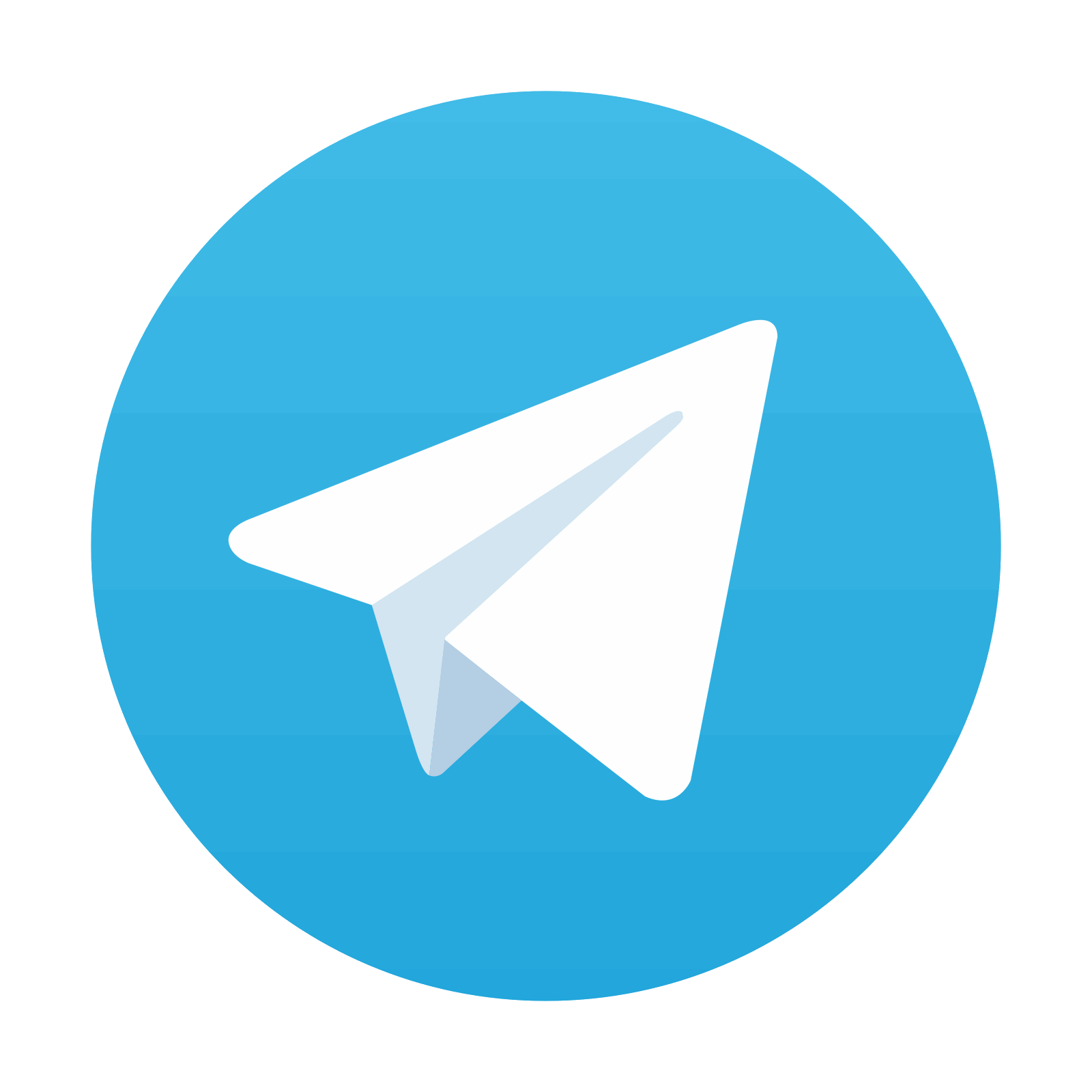
Stay updated, free articles. Join our Telegram channel

Full access? Get Clinical Tree
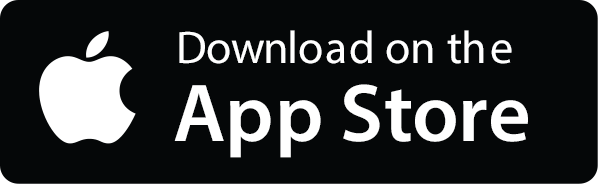
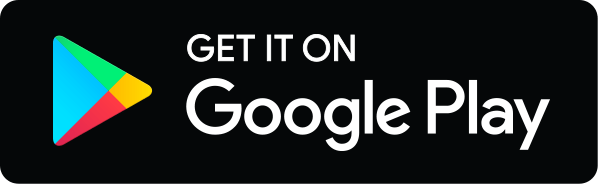
