19 Computer-assisted navigation in proximal tibial osteotomy

1 Introduction
Osteotomies around the knee are well-established methods for treatment of deformities of the lower extremity and monocompartmental osteoarthritis due to congenital or posttraumatic etiology. Numerous osteotomy procedures exist, including open-wedge technique with or without interposition of bone grafts [1], closed-wedge technique [2], and dome-shaped osteotomies. The choice of treatment method is based on clinical findings, taking into account the severity of the deformity, its location, the knee base line, the range of motion, possible rotational malalignment, patellofemoral tracking, leg-length differences, and soft-tissue condition [3].
Osteotomies around the knee, in particular osteotomies at the proximal tibia, are regarded as technically demanding surgical methods with a steep learning curve. Retrospective clinical studies demonstrate that the most frequent complication after osteotomy is insufficient postoperative alignment [4, 5]. An evaluation of recently published clinical findings reveals that only 60-80% of postoperative outcomes reach satisfactory alignment [1, 6–10]. This can often be attributed to inadequate preoperative planning or failure to examine the mechanical axis of the extremity intraoperatively. Another cause may be unstable fixation of the osteotomy during the bone healing process, resulting in secondary correction loss with revarization or valgus overcorrection. Improperly performed osteotomies or incorrect orientation of the chisel or saw blade can cause intra-operative tibial plateau fractures and injury to the neurovascular structures.
The occurrence of complications related to preoperative planning and intraoperative mistakes can be decreased by computer-assisted, navigated procedures. The exact result achieved in this way must nevertheless be securely stabilized by internal fixation so that the surgical outcome is maintained. Ideal fixation can be achieved with angular stable internal plate fixators.
Computer-assisted surgical techniques (CAS) with CT-based intraoperative guidance systems [11] and robot-assisted systems [12] have been developed for closed-wedge correction osteotomies. These systems have proven effective in aiding the surgeon to excise a precise bone wedge. Despite this advantage, these systems also include certain disadvantages, eg, high radiation dose and increased risk of infection after preoperative implantation of the marker pins. For these reasons, a new intraoperative planning and navigation system has been developed that is applied with a fluoroscopic C-arm instead of preoperative CT scans.
In addition to conventional closed-wedge osteotomy, the presented system also allows for open-wedge and dome-shaped osteotomies. It permits the user to measure the deformity precisely, to plan the osteotomy intraoperatively, and to perform the planned operation accurately under navigated guidance.
2 System components
The development of this system was based on the SurgiGATE system (PRAXIM/Medivision, La Tronche, France). An infrared camera fixed to an adjustable stand (Opto Track 3020, Northern Digital Inc, Ontario, Canada) is used for permanent tracking of optical targets that are equipped with infrared light-transmitting diodes. These diodes are attached as dynamic reference bases to relevant anatomical structures (distal tibial shaft, tibial head segment, distal femur), to the image intensifier of the C-arm, and to all relevant surgical instruments, thus permitting real time navigation. A Sun-ULTRA-10 workstation (Sun Microsystems Inc., Mountain View, Canada) is used for image processing and visualization on the monitor. This workstation is connected to the video output of the C-arm in order to receive the radiographic images. The workstation communicates with the infrared system via specially designed software.
The navigated system can be categorized into three groups according to their function:
Image acquisition and recording: Intraoperative movements and changes are tracked with the calibrated fluoroscopic C-arm, a reference measuring instrument, a control system, and a percutaneous pointer.
Navigated instruments: drill, saw, and chisel.
Dynamic reference bases (DRB): The three reference bases are attached at the distal tibial shaft, the tibial head segment, and the distal femur and record all steric changes at these anatomical structures during the operation.
3 Surgical procedure
Preoperative planning is not necessary for application of the described navigation system. The patient is placed in supine position on a radiolucent table. Two dynamic reference bases (DRB) are fixed with 3 mm K-wires to the distal femur and the distal tibia. It is important to ensure rigid fixation of these reference bases. After radiographic imaging and registration of the anatomical landmarks, the deformity is calculated intra-operatively and interactive planning of the osteotomy can be performed. A third DRB is attached to the proximal segment of the tibia before performing the osteotomy in order to monitor relative movements between the proximal and distal osteotomy segments of the tibia. The planned osteotomy and correction of the deformity are then conducted under navigated control.
3.1 Recording of image data and landmarks
At the beginning of surgery a total of six x-rays—AP and lateral view of the hip j oint, the knee j oint, and the upper ankle joint— are obtained ( Fig 19-1 ). Based on these images the anatomical landmarks are digitally captured with a 3-D reconstruction concept [13, 14]. First, the center of the hip joint is defined as the center of the femoral head using a virtual Mose circle. Second, the center of the talus is defined as the center of the ankle joint. Third, the tangent to the posterior femoral condyles is determined. In the next step, the contours of the tibial plateau are defined by the most lateral, most medial, most anterior, and most posterior point of the tibial plateau. The center of the knee joint is then calculated as the geometric center of the recorded contours of the tibial plateau. This procedure permits all anatomical bone structures that cannot be reached directly with the pointer to be registered. Alternatively, the pointer can be inserted percutaneously to digitally record the midpoint on the transmalleolar axis (distance from the lateral to medial malleolus) as the center of the upper ankle joint.
3.2 Measurement of the deformity
After registration of the landmarks, a patient-specific coordinate system is established. For geometric description of bone and joint geometry, each reference point requires its own coordinate system. The frontal plane of the femur passes through the hip and knee joint centers and lies parallel to the posterior tangent of the femoral condyles. The sagittal plane passes through the hip and knee centers and lies perpendicular to the frontal plane. The transverse plane lies in a 90° angle to the other two mentioned planes.
The functional biomechanical parameters of the extremities are measured intraoperatively. Considering the digital hip joint (H), knee joint (K), and ankle joint (A) centers, the femoral mechanical axis, the tibial mechanical axis, and the mechanical weight bearing axis can be defined as the line HK, KA, and HA. The varus/valgus angle and the flexion/extension angle are defined as the intersection angles of the projected lines of the tibial and femoral mechanical axes in both the frontal and sagittal planes [3]. The inclination angle of the tibial plateau in the sagittal plane (tibial slope) corresponds to the intersection angle between the tangent to the tibial plateau and the mechanical tibial axis. Deviation from the mechanical axis is measured as the distance of the mechanical axis to the knee center (mechanical axis deviation = MAD) in the frontal plane and is given in mm [3]. Accurate definition of the above mentioned parameters is mandatory to correctly identify and adequately correct a deformity.

Another important aspect is ligament and soft-tissue laxity. In addition to skeletal varus or valgus deformity asymmetric joint-gap width may be caused by separation of the lateral or medial compartments due to ligament laxity [15]. This must be taken into account when planning the correction angle (see chapter 4 “Basic principles of osteotomies around the knee”). The skeletal and ligamentous causes must be differentiated. A mathematical formula is given in chapter 5 “Detailed planning algorithm for high-tibial osteotomies”. Alternatively skeletal deformities can be differentiated from ligament laxities by exerting varus and valgus stress [16]. The convergent angle of the joint surface (see chapter 1 “Physiological axes of the lower limb”, Fig 1-6 ), which is defined as the intersection angle between the tibial and femoral knee base line, can be calculated with the system software.
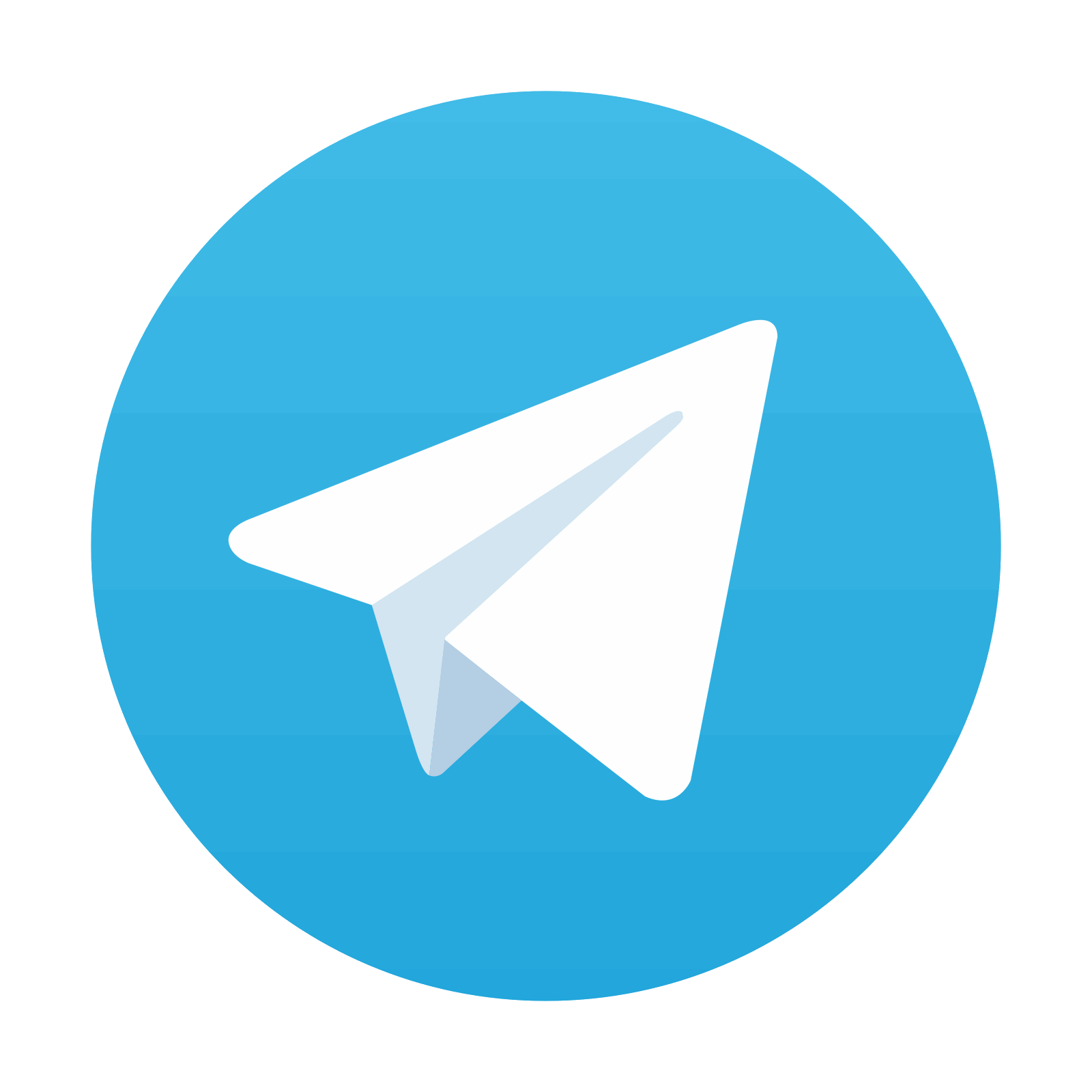
Stay updated, free articles. Join our Telegram channel

Full access? Get Clinical Tree
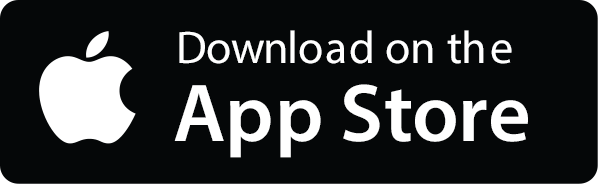
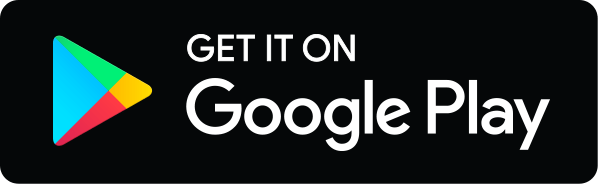
