18 Functional Biomechanics with Cadaver Specimens
Cadaver specimens are a powerful tool for biomechanical testing when it is difficult to control or measure the movements or forces of interest in joints or soft tissues in vivo. Donated specimens allow researchers to better understand a vast array of biomechanical themes, including functional anatomy, pathology, joint motion, material properties of human tissue, and consequences of arthroplasty. Cadaver specimens perform a vital role in preclinical testing of new surgical techniques and implant designs, and can be used to validate computer simulations. Pairs of specimens can often be used in conjunction, with one acting as a control. In some cases, it is possible to have intraspecimen controls, where various interventions are performed on the same specimen, making direct and multiple comparisons possible and eliminating some specimen variability.
Key Concepts: Ethical Issues
The use of cadaveric specimens in medical research has been an integral part of increasing understanding of human body mechanics for many years. Ethical approval should be obtained prior to conducting experimental studies on specimens to ensure donated bodies are used in a responsible manner and for a meaningful purpose. Cadaveric specimens for research purposes can be obtained from hospitals directly where informed consent must be sought from family members of the deceased, or through donor banks where individuals donate their body and a fee can be paid to cover processing costs by registered institutions. Legislation regarding the storage and management of cadaveric tissue varies from country to country and is tightly regulated; therefore, individuals should check with local authorities prior to commencing experiments.
18.1 Specimen Management
Human tissue decomposes if left in an open air environment, and therefore cadavers are commonly preserved using two methods: in a fresh frozen state or in an embalmed state. In the frozen state, tissue should be stored below −20°C and be completely defrosted at room temperature prior to use. Defrosting can take 24 hours or more depending on the size of the specimen. Freezing has been shown not to have a significant effect on collagenous tissue properties 1 or on compression and torsional strength of bone. An alternative to freezing is tissue embalming, where formaldehyde is used either alone or in combination with other fixative agents. The fixation process acts by increasing crosslinks within and between collagen fibers, altering the action of defleshing agents and subsequent tissue decomposition. Although embalming has the potential to prolong tissue usage, there are a number of disadvantages of its use. During biomechanical testing, embalmed specimens have been found to result in loss of range of motion due to tissue stiffening, and there is strong evidence that formalin significantly alters the mechanical behavior of the ligaments and bone, meaning formalin-fixed specimens are not representative of in vivo conditions. Care must therefore be used by the researcher in deciding which preservation method is most appropriate to meet the research objectives of the planned experiment.
Responsible laboratory management of cadavers is vital to prevent tissue decomposition and enable as close as possible comparison to be made with in vivo tissue. Stress relaxation tests have identified that changes in water content influence the viscoelastic behavior of soft tissues, with dehydration found to cause increased stiffness, and overhydration resulting in increased tissue compliance. The effect on creep (the increase in strain with the tissue under sustained or repeated stress) has also been investigated, and although effects were found to be reversible, exposure of ligamentous tissue to sucrose (to cause tissue dehydration) and phosphate buffered saline solutions (to cause tissue overhydration) caused decreased and increased tissue creep, respectively. Water-rich structures such as the menisci and articular cartilage have been found to lose height and thickness following only 1 hour of exposure to air, and the mechanical properties of intervertebral disks have been found to have a significant dependence on hydration. These findings should act as a caution to the researcher to take care when managing tissue in the laboratory setting. A protocol to ensure appropriate monitoring of cadaver tissue hydration is necessary to ensure validity and reliability of experimental results; this may include regular spraying of specimens with a wet solution. If the specimens are thin, testing them in a 100% humid environment may be an option.1
18.2 Experimental Design
The correct choice of hardware and measuring instruments for use with cadaveric specimens is crucial and must be done with the objectives of the study in mind. In general, cadaveric biomechanical studies on osteosynthesis are done to determine mechanical properties (e.g., stiffness, fixation strength, the number of load cycles to failure, and the stability of fixation methods). Three experimental stages are usually carried out to compare biomechanical responses: (1) the intact specimen; (2) after osteotomy, fracture creation, or soft tissue resection; and (3) after surgical intervention or repair.
18.2.1 Experimental Hardware
The anatomical part that is to be studied and the objectives of the experiment both play an important role in the design of the test rig. In general, most studies require the design and construction of a new test rig that can best simulate the biomechanics of the anatomical part for a particular experiment. Major design considerations include desirable range of motion and loading conditions: types of loads (static, dynamic, cyclic, etc.); loading direction (axial, torsional, bending, etc.); magnitudes of loads (e.g., physiological or most unfavorable); and loading methods (e.g., direct to the bone or via muscle pulling). Test rigs can be configured to include a newly designed loading apparatus (Fig. 18.1a). The design can vary from a very simple hanging-weight mechanism to more sophisticated mechanisms consisting of several servohydraulic or pneumatic actuators. For studies aiming to determine mechanical properties or those in which load or displacement is to be controlled, test rigs are commonly used on universal materials testing machines (UTMs) (Fig. 18.1b), equipped with load cells for force measurement. With UTMs, loads can be applied to bones at a variety of different rates and magnitudes as desired, either until specimen failure or between preset limits of load or displacement. It must be noted that the selection and use of UTMs and load cells must be done with awareness of their specifications and limitations of use. In particular, they should be able to reproduce physiological strain rates when simulating injuries, because of the ratedependence of tissue properties. Many university engineering departments will have these machines.

Mounting a specimen in a test rig usually requires custom-designed jigs and fixtures. This is because most standard gripping accessories are not suitable for gripping bone or soft tissues. Bone specimens such as the proximal humerus or distal tibia are usually held in cylindrical or rectangular-shaped metallic pots by means of acrylic bone cement or screws, or a combination of the two. It may also be necessary to make an alignment fixture to ensure that the specimen is mounted with a desired orientation, so that consistent loading conditions are attained as desired. An example of this might be, for example, that a femur is mounted so that the path of an axial compressive load passes from the center of the head of the femur to the center of the knee. Depending on the objectives of the test, mounting blocks can be designed to provide complete immobilization of the socket or to allow socket movements in certain directions (degrees of freedom), depending on allowable motions of each end of the test specimens. This may be important to avoid the mounting “locking-in” unwanted bending effects when the specimen tries to deform under load. In some cases, mounting blocks are also designed so that their positions are adjustable. When designing a test that loads the joint directly, without additional muscle forces (as in Fig. 18.1b), then data on joint forces that have been obtained from instrumented prostheses in vivo are available to download from www.orthoload.com.
The manner in which muscle forces are implemented in a cadaveric experiment is often important, because most of joint force arises from the muscles, rather than from body weight. The rig shown in Fig. 18.1a, for example, includes the action of the quadriceps simulated by means of cables attached to the individual muscle heads close to the patella. The cables are tensed by taking them over pulleys to hanging weights, which are simple and accurate for constant loading. The directions and magnitudes of muscle tension must be derived from the anatomy/physiology/biomechanics literature, for example by sharing the forces among a group of co-contracting muscles by using published data on physiological cross-sectional areas, then scaling the tensions by reference to data on the intensity of electromyographic signals during the relevant physical activity in vivo. Variable tensions may be simulated most easily by using pneumatic cylinders with calibrated air pressure regulators. Advice should be sought from a mechanical design engineer, because there are several other ways to load the specimen, including servohydraulic cylinders, springs, or electromagnetic actuators for example, in order to obtain reliable data in the easiest manner.
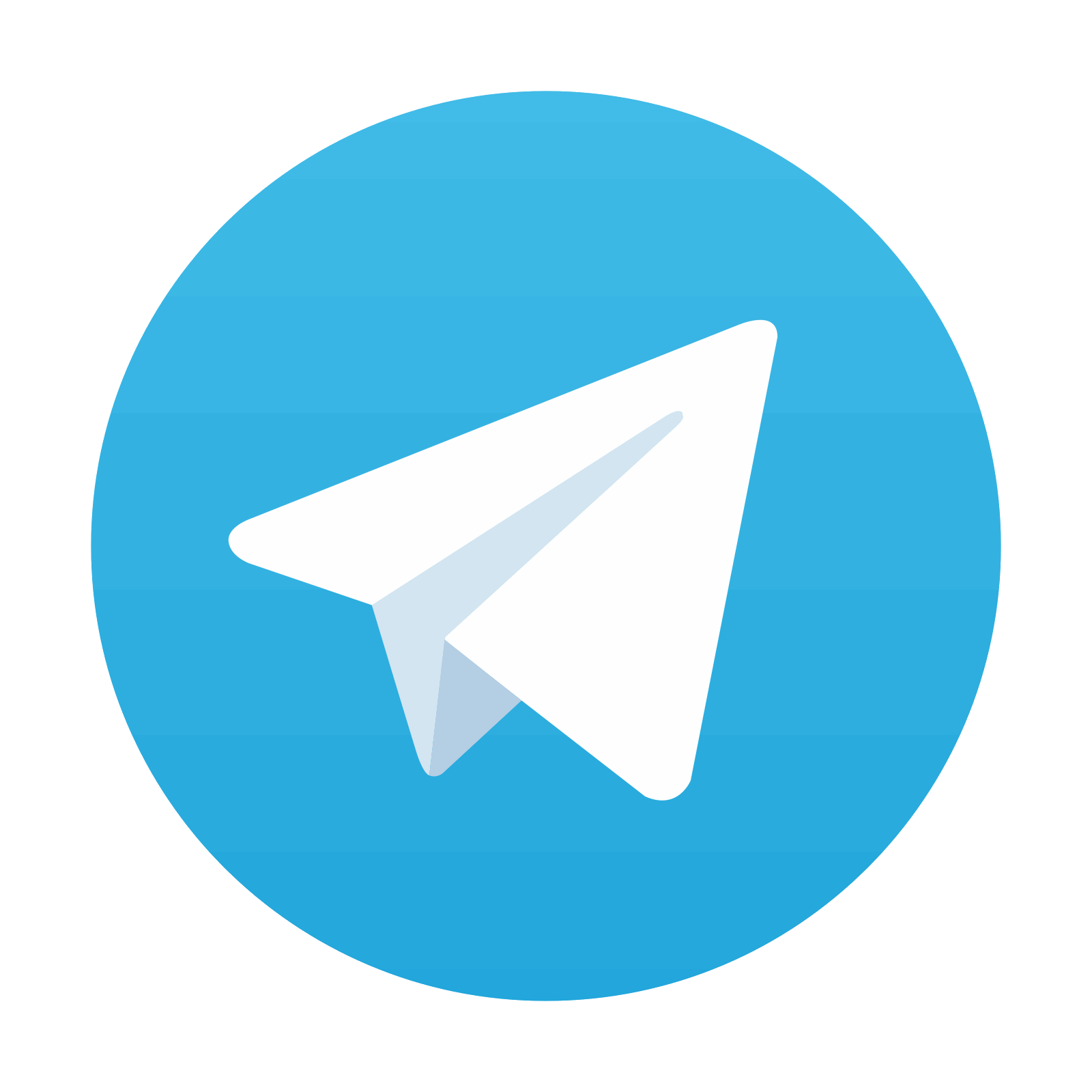
Stay updated, free articles. Join our Telegram channel

Full access? Get Clinical Tree
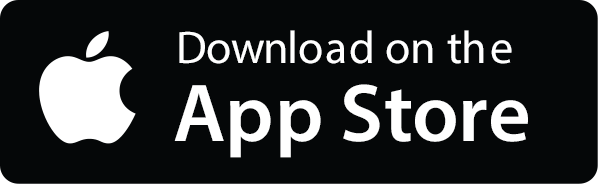
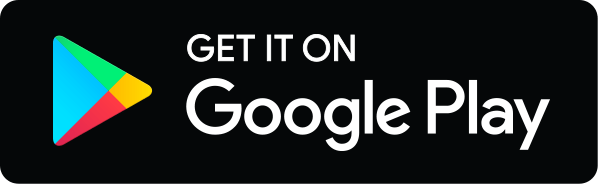
