16 Mechanical Aspects of the Shoulder
The upper extremity is designed to fulfill a large variety of tasks. The “link chain” of upper arm, lower arm, and hand permits motion in a large volume of space. The hand can reach almost any point within a sphere centered at the center of the humeral head and a with radius equal to the length of the arm. The almost unrestricted mobility of the hand enables objects to be gripped and manipulated under visual control as well as in regions out of sight (behind the back, for example). Upper arm, lower arm, and hand can execute quick and forceful as well as fine, very accurately guided movements. Forces and moments can be oriented in virtually any direction. The maximum values of such forces and moments depend on the location and orientation of the hand relative to the trunk. Knowledge of this dependence on position and orientation is of practical importance in the design of workplaces or orthopedic aids and appliances. Further information on this topic is presented in textbooks of ergonomics.
16.1 Link Chain of the Upper Extremity
In humans, as in all other vertebrates, the limbs are fixed to the trunk by means of “girdles,” whose architecture is adapted to the mechanical function of the limbs. The pelvic girdle consists of the iliac bones and the sacrum, joined together by the symphysis and the iliosacral joints. Both these joints are characterized by being very stiff and having a small range of motion. The joint between the sacrum and the fifth lumbar vertebra forms the junction with the trunk. The pelvic girdle is adapted to the function of the legs, which is to support the body weight and locomotion.
Fig. 16.1 shows an overview of the architecture of the shoulder girdle and the arm. The shoulder girdle consists of the clavicle and scapula, connected by the acromioclavicular joint. The scapula glides on the posterior thoracic wall; it is fixed to the trunk by muscles alone. It can translate about 10 cm upwards, downwards, or laterally, and can rotate about an axis perpendicular to its own (imagined) plane. To a small extent, it can also be tilted with respect to the thorax. The clavicle is connected laterally to the acromion (acromioclavicular joint) and medially to the sternum (sternoclavicular joint). These two joints at either end of the clavicle can be described in mechanical terms as ball-and-socket joints which allow the clavicle to rotate in addition about its long axis. The range of motion of these joints is strongly restricted by their ligamentous connections. In point of fact, the shoulder girdle articulates with the rest of the skeleton only via the clavicle. The link between the scapula and the thorax is sometimes called the “scapulothoracic joint,” but it is not a joint in the anatomical sense. Due to its deformability and its loose attachment to the trunk, the shoulder girdle is superbly adapted to upper-extremity functions such as touching, grasping, manipulating, and climbing.

The shoulder joint (glenohumeral joint) links the upper arm to the shoulder girdle. It is a ball-and-socket joint with a large range of motion. The joint surface of the humeral head is spherical; it articulates with the shallow socket of the glenoid cavity of the scapula. The surface area of the glenoid cavity (which is about 6 cm2) is much smaller than the surface area of the humeral head (which is about 24 cm2). 1 Whether there is a precise fit between the head and the socket or whether the radius of the socket is slightly larger than that of the head is a matter of some debate. Radiographic views can simulate an incongruency between head and socket, as the cartilage is thicker at the periphery of the socket than at its center. According to Graichen et al, 2 in addition to rotational motion, translational motions in the order of a few millimeters are possible in the shoulder joint due to the incongruency of head and socket.
The elbow joint, the link between upper and lower arm, moves very much like a hinge joint. The wrist, the link between lower arm and hand, also moves like a hinge joint, and motion is also possible in the plane of the palm of the hand, in the direction of the thumb or the little finger (radial or ulnar deviation). The multiplicity of possible hand motions is further augmented by the rotation of ulna and radius ( Fig. 16.2 ). The relative change in position of these bones permits supination and pronation (rotation about the long axis of the hand) even when the elbow joint is held firm.

The upper arm and shoulder girdle, like the femur and the pelvic girdle, are linked by ball-and-socket joints. Compared with a hinge joint, a ball-and-socket joint has a larger range of motion. To increase the range of motion of the upper extremity as much as possible, the socket (glenoid cavity) is not fixed rigidly but follows the motion of the upper arm. When the upper arm is raised, the scapula undergoes displacement and rotation relative to the thorax ( Fig. 16.3 ). This is made possible by the joints between the scapula and the thorax (as we have said, not “a joint” in the anatomical sense) and between the scapula and the clavicle, which have no counterparts in the pelvic girdle.

Viewed in a plane fitted to the scapula, the ratio of the rotational motion of the upper arm to that of the scapula for arm elevation angles above 30° ranges between 5:4 and 3:1. 4, 5 This interplay between the motions of upper arm and scapula is called the scapulohumeral rhythm. In the individual case the scapulohumeral rhythm is well reproducible and in the case of small loads is virtually independent of the load on the shoulder. 6 With increasing age the concomitant motion of the scapula decreases. 7 The concomitant motion of humeral head and glenoid cavity stabilizes the shoulder joint (see Section 16.4). It also ensures that the humerus does not impinge on the acromion when the arm is elevated above 90°.
16.2 Muscles of the Shoulder Region
The large number of muscles in the shoulder region accords with the wide variety and range of motion of the shoulder. The muscles can be categorized according to their areas of insertion ( Table 16.1 ).
Fig. 16.4 provides an overview of the muscles that move the upper arm forwards, backwards, or to the side, or rotate it about its long axis. Since even simple motions usually involve the activation of several muscles, it is easy to see from the figure that biomechanical models of the shoulder must necessarily be complex. The muscle group consisting of teres minor, infraspinatus, supraspinatus, and subscapularis is called the rotator cuff ( Figs. 16.5 and 16.6 ). Activation of these muscles generates a force that presses the humeral head into the glenoid cavity, thus preventing instability of the shoulder joint. If these muscle forces are absent or a tendon is ruptured, instability of the joint may ensue. Except at the end of the range of motion, the shoulder joint capsule is slack and cannot provide stability.



16.3 Stability of the Shoulder Joint
In mechanical terms, a configuration is referred to as stable if it returns to its initial state after a small external perturbation. A configuration is called unstable if a small perturbation suffices to change it completely (see Section 1.7). In reference to joints of the body, the terms stable and unstable are sometimes used imprecisely. The term instability is sometimes used when merely an increased range of motion is observed. For example, an increased range of motion of adjoining lumbar vertebrae compared to the norm in healthy persons is called segmental instability of the spine. Correctly, it should be called hypermobility, because the articulating bones can always return to their initial position. In the mechanical sense, a joint is unstable only when the articulating bones cannot return to their initial positions after being acted on by an external force or after performing a particular movement.
Instability of the shoulder joint exists if the humeral head dislocates from the glenoid cavity because of low muscle tone or under the influence of an externally applied force. Dislocation may occur during small, everyday movements such as putting on a coat or turning over in bed. This event is analogous to the luxation of the hip seen in patients with a dysplastic acetabulum. Displacement of the shoulder joint is termed caudal, anterior (the most common), or posterior, depending on the direction in which the humeral head dislocates. Athletes in disciplines requiring forceful overhead movements of the arm (javelin throwing, for example) are at increased risk of dislocations of the shoulder joint. 10
The predisposition of the shoulder joint to instability is the price paid for the large range of motion of this joint. To facilitate a large range of motion, the shoulder joint is designed as ball-and-socket joint. This type of joint permits angular motion in a large range and simultaneous rotation about the long axis of the bone ( Fig. 16.7 ). To prevent the ball, or sphere, of the loaded joint from being pushed out of the socket, the socket must enclose a part of the sphere. The more the sphere is enclosed, the smaller the risk of its being pushed out. At the same time, however, the more the sphere is enclosed, the smaller the joint’s range of motion. The range of motion of ball-and-socket joints in the human body is end-stopped by contact between the articulating bone and the rim of the socket. In the example shown in Fig. 16.7 the socket encloses the sphere by 180°. The range of motion α is, however, much less than 180°. If the diameter of the long bone where it transitions into the sphere were smaller, this would increase the range of motion—but the diameter of the bone cannot be reduced too much without excessively compromising its strength.

Alternatively the range of motion can be increased by reducing the extent to which the sphere is enclosed by the socket. Nature chose this design in the shoulder joint, combining the spherical head of the humerus with a shallow socket ( Fig. 16.8 ). However, when the socket encloses only a small portion of the sphere, the risk of instability increases. In a friction-free ball-and-socket joint the vector of the joint load is perpendicular to the joint surface; it intersects the center of the sphere. In the absence of additional safety measures, the sphere will always be pushed out of the socket when the line of action of the joint load F does not intersect the socket. In the example shown in Fig. 16.8 , the force F generates a moment in relation to the lower rim of the socket


which tends to lever the sphere out of the socket (the minus sign results from the sign convention for moments). In this situation, a reaction force F 1 acting from the socket on the sphere cannot alone—that is, without additional muscle or tendon forces—establish mechanical equilibrium.
The shoulder joint is protected against instability in three ways. First, in the scapulohumeral rhythm the socket follows the motion of the arm. This increases the likelihood that the line of action of the load vector, which always points approximately along the direction of the upper arm, will intersect the socket. Secondly, the muscles of the rotator cuff can exert an additional force on the humeral head, pressing the head into the socket. As an example, Fig. 16.9 shows a joint load vector F which initially does not point into the socket. However, if, in addition to the force F, a muscle force F m is exerted, the vector sum F r of the two forces points into the socket and provides joint stability. Thirdly, the shoulder joint is stabilized by the joint capsule and the ligamentous connections between humerus and scapula (coracohumeral and glenohumeral ligaments). Slack in the middle of the range of motion, these structures become tight at the end of the range, where they assist in stabilizing the joint. 11

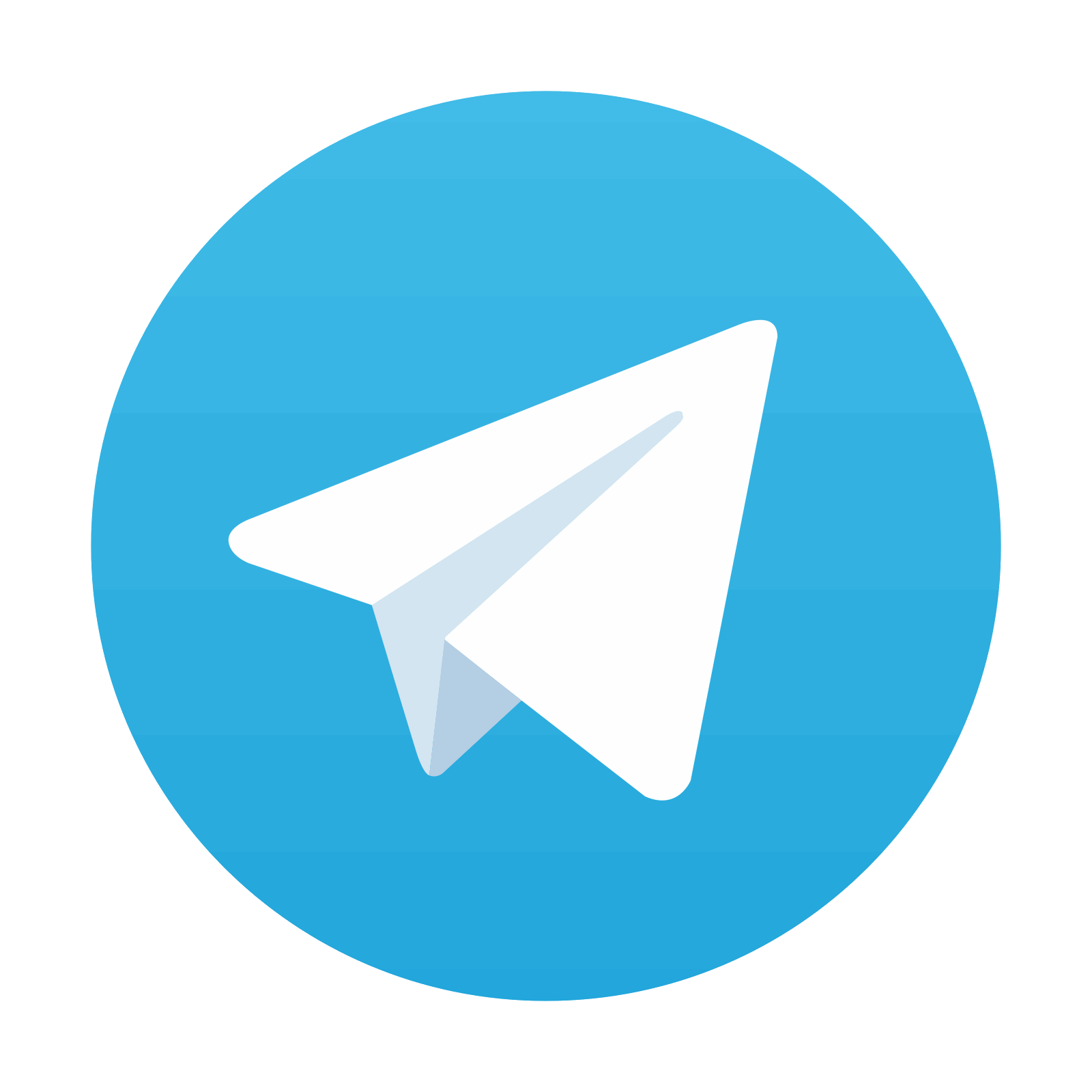
Stay updated, free articles. Join our Telegram channel

Full access? Get Clinical Tree
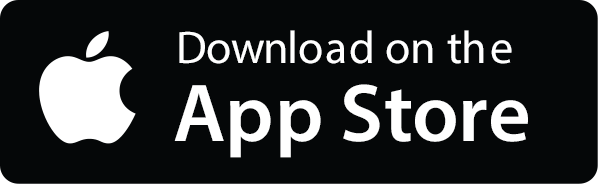
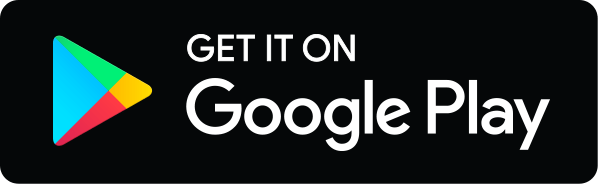