10 Wound closure and coverage techniques (IV)
10.6 Free flaps—principles
Authors Stefan Langer, Hans-Ulrich Steinau, Christoph Andree, Lars Steinsträßer
10.6.1 History
Since the 1980s, the microvascular technique has become a standard procedure particularly in plastic surgery. It is of great help for reconstructive surgery because almost any defect at any site of the body has become “repairable” using autologous tissue transplantation. The range of flaps has increased dramatically during the last decades, as a result of various anatomical works describing meticulously the anatomy of muscle pedicles (chapter 10.3). By now, almost every muscle can be raised as a free flap ( Fig 10.6-1a–d ). With increasing interest in the vascular distribution within muscles, the contribution of blood circulation in muscles for the overlying skin was finally recognized, ie, each superficial muscle provides vascular connections via musculocutaneous and/or septocutaneous perforating vessels to the overlying skin (chapter 2). Accordingly, it became possible to include a segment of skin with the muscle flap, thus creating the musculocutaneous flap [52]. Based on Taylor′s concept of skin circulation [35], fasciocutaneous [53] or perforator flaps [54] have been described that are widely used today (chapter 10.3). Refinements in free-flap surgery are made with regard to donor-site morbidity (ie, functional loss, extensive scaring, and contour deformity) and the choice of the most suitable flap for a specific defect including tissue composition, volume, texture, and color.

10.6.2 Basic considerations in free flap surgery
Surgical skills
Any surgeon can be trained in microsurgery. He or she requires similar technical skills, good eye-hand coordination, great care in handling tissues in general and soft tissues in particular, as well as much patience and practice. Given proper instruction and training in a microsurgical laboratory course of 1–4 weeks, the necessary skills needed to pass into the clinical setting can be acquired. Before actually performing an anastomosis, several preconditions should be met as defined by Acland [55]:
careful preoperative planning
enjoyable working environment
appropriate equipment (surgical loupes, microscope, micro instruments, and sutures) adapted to these vascular calibers
comfortable sitting position and hand support
anatomical knowledge of access and exposure
proper choice of vessels
hemostasis.
Equipment for microsurgery
The microsurgeon sees his operative field magnified through two instruments: the surgical loupes and the operating microscope. The operating microscope traditionally provides the necessary magnification (~ 12-fold) and illumination for microsurgery in order to adequately perform the anastomosis of vessels of ~ 1–4 mm in diameter, respectively sutures of nerves. The microscope should be double headed in order to allow both surgeon and assistant to see the same operative field, which is transmitted by beam splitters. In addition, individual control of focus and zoom mechanisms through separate optical systems and remote control by foot are advisable. Finally, coaxial illumination is a necessity in order to guarantee a clear, bright, and sharp visual field without shadows. Video and photographic attachments compliment the entire system. Disadvantages include the cost, size, maintenance, setup time and adequate storage of the equipment.
The use of high-power and customized surgical loupes (magnification 2.5–4-fold) has been advocated for the crucial steps during dissection of the flaps and their vascular pedicles at the donor site as well as the preparation of the recipient vessels. Furthermore, refinements of the loupes concern the variability of the working distance (~ 25–50 cm), the width of the visual field ranging from a normal to an extended field, the integrated light source and the weight. Altogether this generates more freedom of movement than a microscope. Microscopes mounted on a head set may eventually combine the advantages of the operating microscope and the surgical loupes, though the wearing comfort is limited due to weight and a tensed and stable fixation onto the head, possibly inducing head and neck pain. Although the microscope on a foot rest may be cumbersome, it is still the ideal magnifier because it allows the assistant to see what the surgeon sees.
The list of instruments necessary to perform microsurgical procedures includes ( Fig 10.6-2 ):
jeweler′s forceps (straight and angled)
micro-scissors (round-tipped for dissection and sharptipped for vessel cutting)
needle holder (round-tipped for easier passing of the needle through the vessel)
vessel-dilating forceps
micro-toothed forceps
microsurgical clamps (approximating or double clamp and single clamp) of different sizes. Do not use “bulldog” clamps because of the traumatizing “imprints” to the vessel wall.
clamp-applying forceps.
All of these instruments are extremely delicate and, therefore, highly prone to damage.
Nonresorbable polypropylene or polyamide 8-0 to 9-0 sutures are usually used for vascular anastomosis in free-flap surgery and 10-0 sutures for digital arteries and veins. The appropriate needle is nontraumatic and round in shape.
In search for faster “no-suture” methods for anastomosis, a variety of devices is currently in use or under clinical investigation, such as staples, ring clips, coupling devices, magnets, and laser welding. However, except for the venous coupling device, none of the “faster” methods has yet proven to be superior to a diligent and meticulous microsurgical technique, especially in the reconstruction of defects in the lower extremities.
Anastomoses
Atraumatic tissue handling refers to minimizing damage inflicted on the vessel, both in dissection and anastomosis and directly correlates with patency of the anastomosis. The following factors may influence anastomotic patency [56]:
atraumatic surgical handling of the vessel
diameter of the vessel
flushing of the flap
absence of tension and kinking of the anastomosis
mean arterial pressure and blood flow especially during freeing of blood flow after completion of the anastomosis and during reperfusion of the flap
use of anticoagulant and antithrombotic molecules (dextran, unfractionated heparin, low-molecular-weight heparin, acetylsalicylic acid, etc).
An anastomosis may be carried out in an end-to-end or an end-to-side fashion, using the interrupted single-stitch technique with the surgeon′s knot lying on the outside of the vessel′s lumen ( Fig 10.6-3a–b ). Alternatively, a venous anastomosis can be performed with uninterrupted or interrupted sutures according to the preference of the surgeon.
Since compression and kinking of vascular pedicles are associated with disturbed inflow and/or outflow, some advocate the local application of ~ 2 ml fibrin glue once the anastomosis is completed, even though fibrin glue is not seen as an alternative to suturing [57]. Fibrin glue seals small holes within the vessel wall and gives the pedicle some rigidity and adherence to the adjacent tissues, protecting it from shear stress-induced movements, while tailoring the flap during its placement.


10.6.3 Indications and composition of free flaps
As a free flap is transferred with intact circulation, its use is indicated whenever the simple skin graft would be out of place and whenever a flap is preferable or even necessary in order to cover exposed, vital or nonvascularized structures (ie, tendons, bare bone, joints, or implant material) ( Fig 10.6-4a–f ). Other indications are the restoration of function (muscle = functional motor unit) or cosmetic units. Free flaps are also used to pad bone prominences (ie, sacrum, ischium, trochanter, or heel) and to cover traumatized areas for which secondary operations are planned in order to repair underlying structures (eg, implant exchange and bone grafting for nonunion, knee prosthesis for post traumatic osteoarthritis). Customized free flaps are more versatile and can be optimally adapted to a defect in comparison to local or regional pedicled flaps if available at all. Whether it is preferable to use fasciocutaneous tissue instead of muscular tissue, particularly in regard to contaminated or infected traumatic wounds, is still a matter of debate. Mathes et al were the first to experimentally compare in vivo musculocutaneous flaps with randomly perfused skin flaps in order to determine the bacterial clearance and oxygen tension of each [58]. Bacterial challenge of the flaps resulted in total necrosis of the skin flap, whereas the musculocutaneous flaps demonstrated long-term survival. These results correlated well with a low bacterial count and a high oxygen tension measured beneath the musculocutaneous flap. Although microvascular tissue transfer has become the gold standard for complex defects in the lower extremities, no prospective study comparing fasciocutaneous flaps ( Fig 10.6-5a–b ) with muscle flaps ( Fig 10.6-6 ) has been carried out to date. Nevertheless, the good results obtained with fasciocutaneous flaps have seriously challenged the dictum of only reconstructing soft-tissue defects with muscle flaps, particularly in the presence of infection ( Fig 10.6-7a–f ) [59]. From an antiinfection point of view, fasciocutaneous flaps seem to be of equal value in comparison to muscle flaps. Moreover, fasciocutaneous flaps are more pliable and often result in a better match with the cutaneous environment than muscle flaps. Angiogenesis allows a faster and safer integration of the flap into surrounding tissues, thus rendering these flaps less dependent on the pedicle in comparison to muscle flaps. Accordingly, fasciocutaneous flaps will tolerate secondary surgical procedures earlier and better [60]. However, defects with considerable, deep tissue loss including bone might still benefit from large muscle flaps acting as a plug.


Special subgroups of free flaps comprise:
The composite flap (eg, osteoseptocutaneous fibula flap (chapter 12.20), musculocutaneous latissimus dorsi flap): a flap consisting of various tissues (eg, skin, fascia, muscle, bone, cartilage).
The chimeric flap (eg, latissimus dorsi and serratus flap) (chapter 12.19): a versatile tissue construct combining regional flaps, the pedicles of which all derive from a single main vessel [61] ( Fig 10.6-8 ).
The prelaminated flap: Additional tissue is added to an existing flap (without manipulation of its axial blood supply) in order to produce a multilayered, composite flap [62].
The prefabricated flap: It involves the introduction of a new blood supply by means of transferring a vascular pedicle into a volume of tissue. After a period of neovascularization, this flap may be transferred, based only on its implanted vascular pedicle [62].
The flow-through flap: Both the proximal and the distal ends of the vascular pedicle acting as a conduit are anastamosed to provide blood flow to distal tissues, eg radial forearm flow-through flap. Flow-through flaps may be considered if segmental revascularization and coverage of a defect is indicated, because these flaps provide dual functionality in one operation, ie one native vascular conduit and at the same time tissue for defect coverage. Accordingly, two separate operations including reconstruction of the vascular conduit by means of a vein graft subsequently followed by flap coverage of the defect can be avoided, as can the morbidity of both procedures [63, 64].

Classification of free flaps according to their perfusion demand:
High-flow flaps: high perfusion demand such as muscle flaps
Low-flow flaps: low perfusion demand such as fasciocutaneous or osseous flaps
High-flow flaps are composed of tissues that consume a lot of oxygen, which is not synonymous with partial oxygen tension of the tissue (pO2). In contrast, low-flow flaps are composed of tissues that consume little oxygen. Skeletal muscles and skin show pO2-values of 20 mmHg and 45 mmHg, respectively.




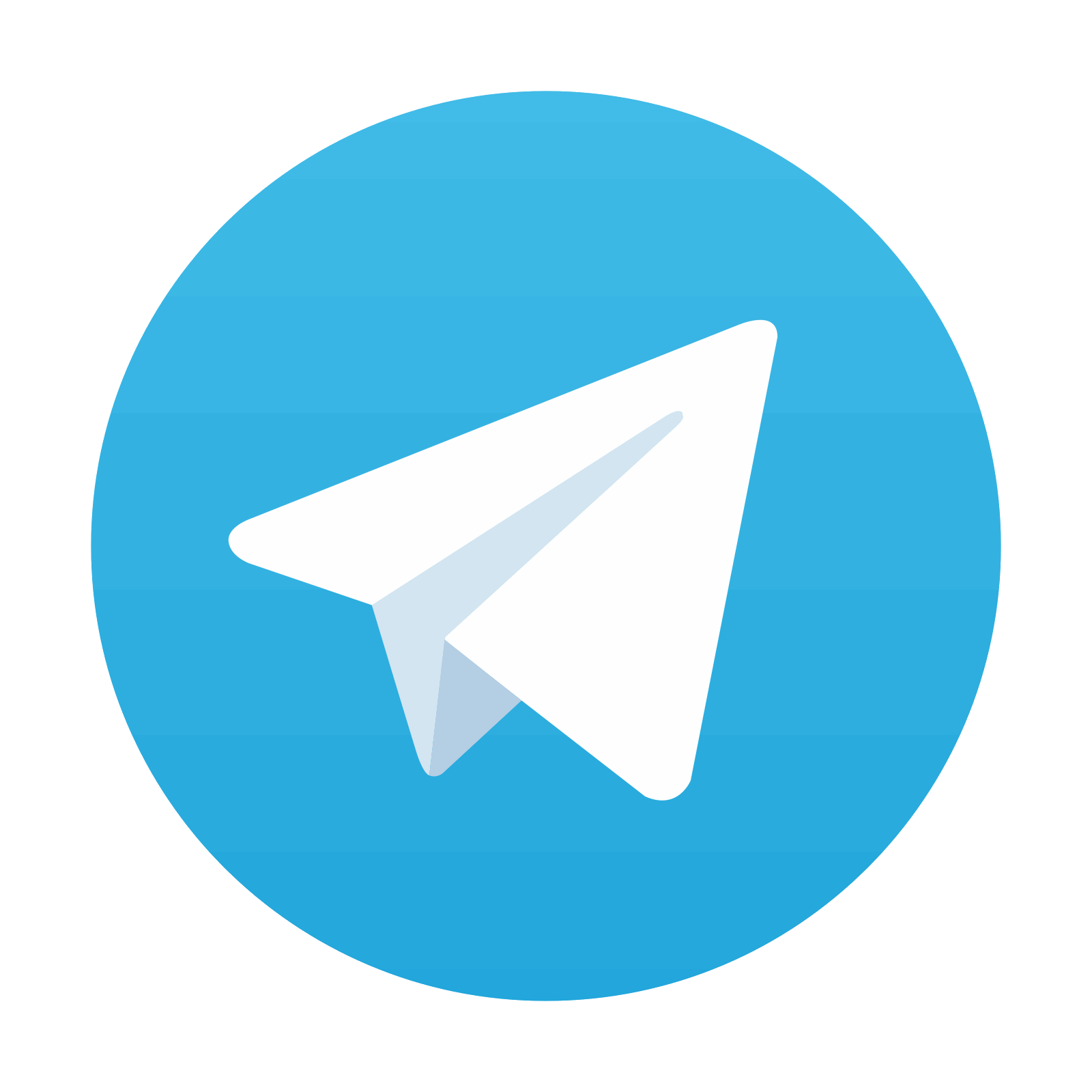
Stay updated, free articles. Join our Telegram channel

Full access? Get Clinical Tree
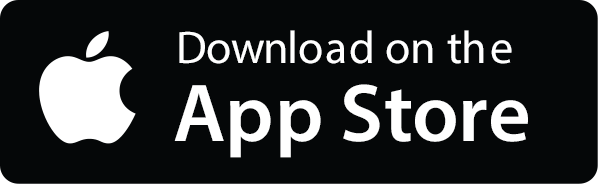
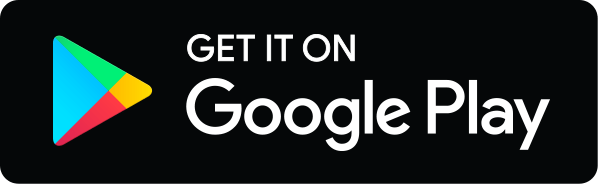
