10 Biologics Used in Bone Healing
10.1 Introduction
Bone healing is not the only goal of fracture surgery, but is always a primary goal and can be the most challenging. There are three basic biologic requirements for achieving this goal of functional bone healing—osteogenic cells, osteoinductive factors, and osteoconductive scaffold. 1 In the setting of fractures, bone defects, and nonunions, the mechanical environment is a critical fourth component for success, leading to the use of the “diamond concept” terminology. 1 For this discussion regarding the use of biologics in bone healing, it is imperative that focus on the mechanical component is not lost as biologic augmentation alone will not be effective in reaching the goal of functional bone healing, and the associated costs, time, and effort will be wasted. Adherence to the AO principles of fracture treatment is still required. 2
As researchers attempt to find the Holy Grail in terms of a single solution for achieving functional bone healing in all clinical situations, it is evident that multiple approaches are currently necessary for a complete armamentarium capable of successfully treating the typical spectrum of cases. The use of biologics and bone graft substitutes for bone healing has been the focus of a great deal of basic science and clinical research. This chapter reviews the uses of currently available biologics for enhancing bone healing based on best current evidence.
10.2 Autologous Bone Graft—The Gold Standard
Over 500,000 bone grafting procedures occur in the United States every year with associated costs in the hundreds of millions of dollars. 3 To date, autologous bone grafts remain the gold standard for enhancing bone healing primarily because of their osteogenic, osteoinductive, and osteoconductive properties. In addition, some types of bone autografts, such as a tricortical iliac crest graft, can aid in mechanical stability. Autogenous bone grafts are also readily available and relatively cost-effective. Although there can be donor site morbidity associated with harvesting the bone, autograft eliminates the concern for generating an immunogenic response and for disease transmission. However, there are consequences of harvesting bone from patients, including chronic pain, infection, iatrogenic nerve injury and fractures, and limited supply in some situations. 4 , 5
The two primary forms of autogenous bone graft include cancellous and cortical. 3 Cancellous bone graft is most commonly used and contains mesenchymal stem cells (MSCs) and osteoblasts with a trabecular structure that provides osteogenic and osteoinductive properties that make it eight times more metabolically active than cortical bone. Autogenous cancellous bone grafts are osteoconductive and support early revascularization and incorporation with graft resorption and active bone formation as early as 4 weeks. Cortical bone adds mechanical stability, but is less biologically active and requires osteoclastic resorption for revascularization and incorporation. Revascularization of cortical bone takes approximately 8 weeks and the resorption process leads to decreased mechanical properties during this time period.
A number of harvest sites are available for obtaining autogenous cancellous bone grafts. Virtually any long bone metaphyseal region can be used, but the most common site for clinical harvest is the iliac crest. 1 Iliac crest provides abundant graft and the harvesting technique is familiar to most orthopaedic surgeons. Complications associated with harvesting from the iliac crest occur in up to 40% of patients and include fracture, seroma or hematoma, infection, and pain 6 , 7 , 8 (Fig. 10.1).

10.2.1 Reamer–Irrigator–Aspirator
The Reamer–Irrigator–Aspirator (RIA) (DePuy Synthes, West Chester, PA) has gained popularity as a method for harvesting autograft from the medullary canal of long bones, primarily the femur. Multiple studies have described RIA-harvested grafts, including harvested cell characteristics, 9 viability, and osteogenic potential of the cells, 5 , 9 , 10 growth factors, 10 , 11 and associated complications. 4 In comparison to iliac crest graft, graft obtained via RIA from the femoral canal has similar osteogenic potential, 5 larger bone graft volumes, 12 similar rates of union, and infection in treatment of nonunions or bone defects, and is associated with less postoperative pain. 13
10.2.2 Vascularized Autograft
In some cases of recalcitrant nonunion, bone defect or poor vascular bed (e.g., femoral head avascular necrosis), vascularized bone can used to improve the chances for functional bone healing. By transferring corticocancellous bone graft with its blood supply, the graft is rapidly incorporated and is biomechanically superior to nonvascularized autograft during the first 6 to 12 months, which is the time period needed for nonvascularized grafts to be incorporated 3 (Fig. 10.2). However, the anatomical constraints, the skills required for harvesting and microvascular anastomoses, and the donor site morbidity associated with these techniques limit their use.

10.3 Platelet-Rich Plasma and Mesenchymal Stem Cells
The autogenous biologic therapies, platelet-rich plasma (PRP) and mesenchymal stem cells (MSCs), are used to enhance bone healing, primarily for fracture nonunions. The goal in using these therapies is to promote fracture union by providing stimuli for fracture healing while minimizing costs, complications, and morbidity. PRP is osteoinductive and MSCs are osteogenic. However, these therapies are not osteoconductive alone so are typically combined with scaffolds such as auto- or allograft bone, or synthetic substitutes, when used in bone healing situations.
As presented in previous chapters, PRP is used more commonly to address soft tissue or articular pathology, but it has been used in fracture management based on its potential for recruitment of stem cells and endothelial cells. 14 Platelets contain more than a thousand bioactive proteins in granules, some of which can stimulate fracture healing, including platelet-derived growth factor (PDGF) and transforming growth factor-β (TGF-β). 15 It is thought that PRP is osteopromotive rather than osteoinductive because the growth factors known to be released from platelets affect primarily the early stages of bone healing. 16
Most data available regarding use of PRP in bone healing are from animal models. Little evidence exists for benefit in adding PRP to autograft, allograft, or MSCs. 17 When combined with synthetic grafts, however, PRP is beneficial in providing osteopromotive-osteoinductive properties to the osteoconductive matrix of synthetic bone substitutes. 18 When percutaneously injected, PRP has been reported to improve impaired cellular proliferation and chondrogenesis in a diabetic rat femur fracture model. 16 However, PRP alone or combined with allograft is less effective at bone formation when compared with individual growth factors, such as bone morphogenic protein-7 (BMP-7). 19 , 20
MSCs have appealing potential for clinical use in stimulating bone healing. Percutaneous harvesting techniques from the iliac crest and adipose tissue allow for retrieval of MSCs from patients with fractures, bone defects, and/or nonunions with minimal risk for donor site morbidity and disease transmission. However, the number of viable MSCs retrieved using these methods is relatively low (1 per 500,000 nucleated cells at most), varies significantly from patient to patient, and decreases with age (1 per 1,000,000 nucleated cells in elderly patients). 21 To increase the number of MSCs available for use in a patient, it has been suggested that multiple harvests and concentrate cells using centrifugation be taken. 22 This technique has been reported to increase the average number of available MSCs from approximately 600 to 2,500 cells/cm3.
When MSCs were used in patients with atrophic tibial nonunions, there was a significantly lower chance of union when MSCs were less than 1,000 cells/cm3 on initial harvest and less than 30,000 cells/cm3 when concentrated. Although injection of MSCs was associated with an 88% union rate when the cells were concentrated, injection of MSCs has fallen out of favor for use in fracture care because the cells tend to migrate from the fracture site and lead to heterotopic ossification. 21 One potential solution to minimize migration is to combine MSCs with demineralized bone matrix (DBM). However, when direct-harvest or concentrated human MSCs were combined with DBM in an athymic rat critical-size femoral defect model, bone union was not reliably achieved. 23
More recent technology allows for combining of MSCs and PRP in a controllable, concentrated form as bone marrow concentrate (Angel System, Arthrex, Naples, FL). Bone marrow is aspirated from any appropriate location (iliac crest, long bone metaphysis) and processed using centrifugation in conjunction with a proprietary platelet sensor to concentrate bone marrow–derived MSCs in PRP. This technology may provide a point-of-care biologic that has osteogenic, osteoinductive, and osteopromotive capabilities for use in enhancing bone healing. Based on this potential, this is the injectable biologic we currently use in our clinical practices. 24
10.4 Growth Factor Therapy
Commercially available growth factors for enhancing bone healing include recombinant bone morphogenic protein (rhBMP) 2 and 7. 25 BMPs are part of the TGF-β superfamily. These BMPs stimulate the production of osteogenic cells. BMP-2 is Food and Drug Administration (FDA)-approved for use in open tibia fractures within 2 weeks of injury and single anterior interbody level lumbar spine fusion. 25 BMP-7 (OP-1) has a humanitarian exemption from the FDA for use in recalcitrant long bone nonunions and revision posterolateral lumbar spine fusion. Common issues with each include need for supraphysiological doses, high cost, and antibody development. 25
Preclinical and early clinical studies investigating BMP-2 were very favorable for increasing fracture union in open tibia fractures and anterior interbody lumbar spine fusion. 25 However, more recent studies, as well as reports of inappropriate data reporting (primarily in spine procedures), have called into question its effects. 26 Two prospective trials revealed that higher severity open tibia fractures (Gustilo and Anderson type IIIa and b) had a lower rate of further surgery and decreased infection rates. 27 Furthermore, BMP-2 combined with allograft has a higher rate of union compared to autograft alone in delayed reconstruction of tibia fractures with bone loss. 28 Complications associated with BMP-2 include ectopic bone formation in fracture patients 29 and life-threatening cervical swelling in spine patients. 26
BMP-7, on the other hand, has primarily been used in nonunions. A Cochrane database review revealed four prospective randomized controlled trials comparing BMP-7 with autograft in tibial fracture nonunions and showed no significant difference in union rates. 30 However, Friedlander et al reported that donor site pain in the autograft group continued up to 2 years after surgery in 13% of patients. 31 Multiple case series have shown similar union rates to the prospective trials of approximately 80% in tibial nonunions and up to 100% in humeral nonunions. 27 BMP-7 has also been reported to be significantly more effective in long bone nonunion healing when compared with PRP in a randomized control trial. 32 When to use autogenous cancellous bone graft with BMP-7 versus when to use BMP-7 alone is the primary question for clinical use of this growth factor. Using BMP-7 without autograft avoids donor site morbidity and the reported success rates associated with use of BMP-7 in nonunions is approximately 85% with or without autograft. 27 However, in recalcitrant nonunions, it is generally considered prudent to combine autogenous cancellous bone graft with BMP-7 in order to maximize the chances for success in these difficult cases.
Both commercially available BMPs are associated with complications. Outside of spine surgery, as indicated previously, the BMPs are primarily associated with ectopic bone formation. 27 The cost of these products should also be a consideration for use, especially considering the lack of significant improvement in success for treating nonunions when compared to autograft. When considering cost-effectiveness, there appears to be benefit for use of BMP in open tibia fractures and potentially for use in nonunion surgery based on faster healing rates. 27 Based on safety, efficacy, and cost considerations taken together, BMPs are not recommended for ubiquitous use but should be considered for use on an individual patient basis when functional bone healing will be compromised (Fig. 10.3).

Although there are only two growth factors that are currently commercially available, several others are being investigated. Vascular endothelial growth factor (VEGF) and hypoxia-induced factor-1a (HIF-1a) show promise in basic science studies, but are not currently commercially available based on cost, their relatively short half-life, and processing, preservation, and handling limitations. 25
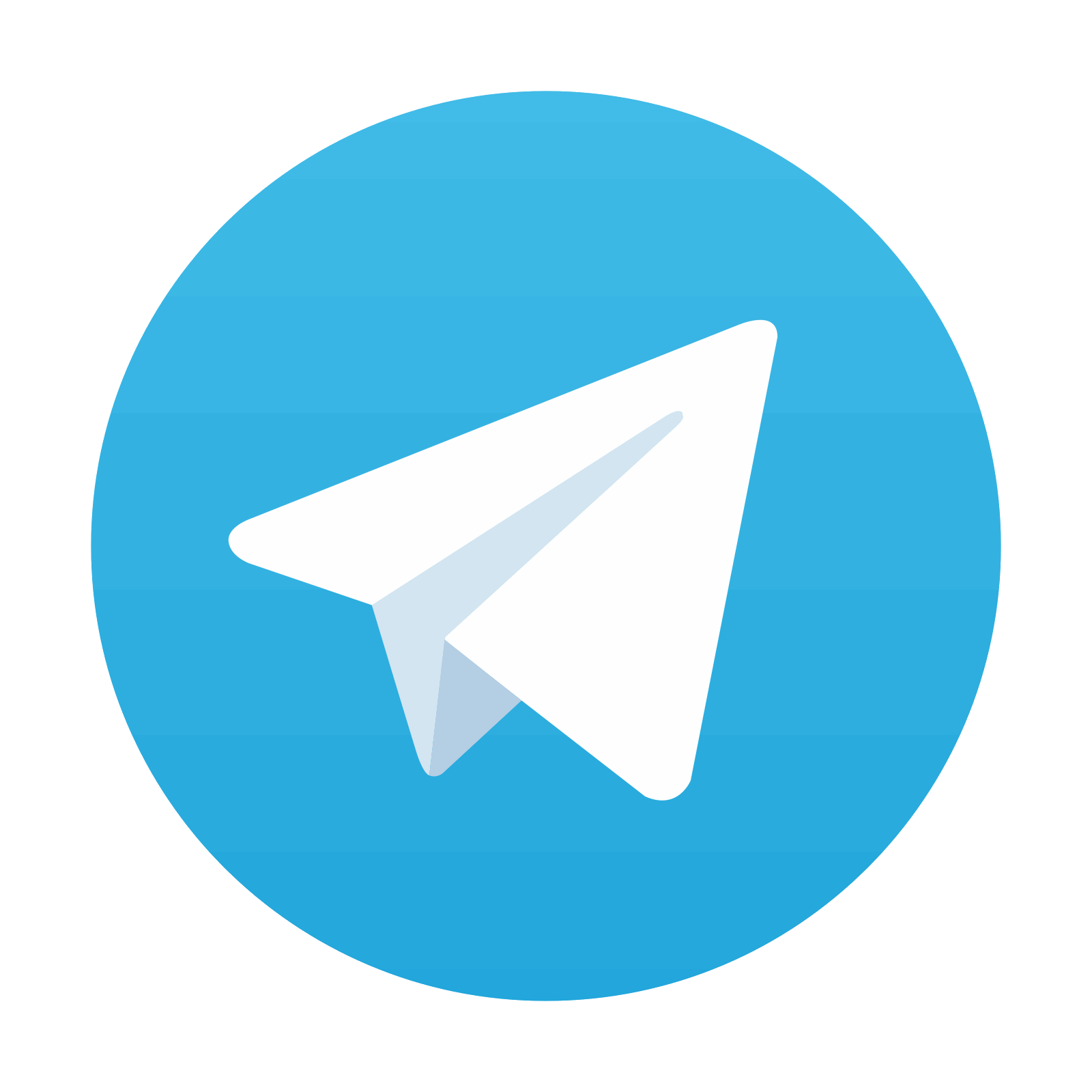
Stay updated, free articles. Join our Telegram channel

Full access? Get Clinical Tree
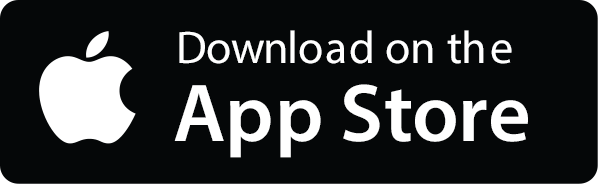
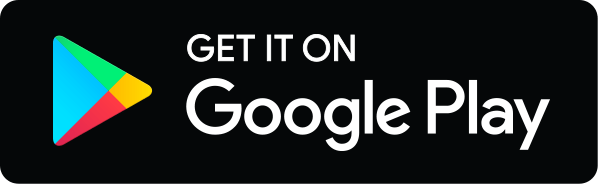