1 Physiology of Fracture Healing
Introduction
Fracture fixation is an important part of orthopaedic trauma. Understanding the physiology of fracture healing will provide insight into the biologic and mechanical factors at play in bone healing. This chapter provides an overview of the components of fracture healing, how a fracture heals, and the clinical relevance of these topics (▶Video 1.1).
I. Fracture Healing Components
Bone blood supply and the effects of fracture and reaming
Endosteal blood flow from high-pressure system nutrient arteries creates a centrifugal (inside to out) pattern of blood flow.
Periosteal blood flow from low-pressure system supplies the outer ~one-third of bone cortex.
Fracture disrupts blood flow causing acute hematoma to develop at the injury site.
Reaming damages the endosteal blood supply and temporarily changes the blood flow pattern to centripetal (outside to in).
Cells
Mesenchymal stem cells (MSCs)—precursors to blood vessels, muscle, fat, cartilage, and bone. Differentiate down different pathways depending on the mechanical and biologic signals received (▶ Fig. 1.1 ). Can come from remote or local cell populations. In bone, local populations are periosteal, endosteal, muscle, blood vessels, and bone marrow.
Fig. 1.1 Factors involved in differentiation of mesenchymal stem cells (MSCs). Depending on the signals received, MSCs can differentiate into blood vessels, bone, cartilage, muscle, or fat. Arrows are positive signals promoting differentiation into that cell type. T’s are negative signals preventing differentiation into that cell type. BMP-2, bone morphogenetic protein 2; C/EBP, CCAAT-enhancer-binding proteins; EGF, epidermal growth factor; FGF-2, fibroblast growth factor 2; LRP5/6, low-density lipoprotein receptor-related protein 5/6; MEF2, myocyte enhancer factor-2; MRF, muscle regulatory factors; Osx, osterix; PDGF, platelet-derived growth factor; PPAR, peroxisome proliferator-activated receptor; TGF-β transforming growth factor beta; VEGF, vascular endothelial growth factor.
Chondrocytes—derived from MSCs and form cartilage intermediate during endochondral bone formation.
Osteoblasts—derived from MSCs or via transdifferentiation of hypertrophic chondrocytes. Produce collagens and other proteins (e.g., osteocalcin, osteopontin) to form extracellular matrix (ECM) and also secrete regulatory proteins (e.g., osteoprotegerin [OPG], receptor activator of nuclear factor kappa-B [RANK] ligand, bone morphogenetic proteins [BMPs]) that affect fracture healing processes. They have parathyroid and vitamin D receptors and are responsible for matrix production during intramembranous and endochondral bone formation.
Osteocytes—fully differentiated osteoblasts that exist in mature bone matrix. They respond to parathyroid hormone (PTH) or mechanical loading and secrete sclerostin to help regulate osteoblastic bone formation or osteoclastic bone resorption.
Osteoclasts—derived from monocytes/macrophages, not from MSCs. These cells are responsible for bone resorption during intramembranous bone formation and remodeling. These attach to bone surfaces via integrin receptor signaling. Once attached, a ruffled border is created forming a local acidic environment to dissolve hydroxyapatite. Osteoclasts have the RANK receptor. PTH stimulates bone resorption by increasing osteoclast activity indirectly via osteoblast production of RANK ligand (RANKL).
Extracellular matrix (ECM)
Organic component of ECM consists of different collagens and other stored proteins responsible for cell adhesion and signaling. The collagen expressed varies throughout the different stages of secondary fracture healing. The organic component of mature bone tissue is known as osteoid.
Inorganic component is predominantly hydroxyapatite (Ca5(PO4)3OH). Mineralization of osteoid results in mature bone tissue.
Cytokines (proteins that modulate immune response and cellular communication), growth factors (proteins that affect cell differentiation, proliferation, and function), and transcription factors (intracellular DNA-binding proteins that modulate gene transcription): There is a myriad of these. Their function depends on location and time of expression during fracture healing.
Proinflammatory cytokines (e.g., tumor necrosis factor alpha [TNF-α], interleukin-1 [IL-1], IL-6)—recruit inflammatory cells, promote angiogenesis, modulate osteoblast/osteoclast differentiation, and affect cellular gene expression.
Transforming growth factor beta (TGF-β) superfamily, including BMPs—carry out MSC recruitment and differentiation into chondrocytes or osteoblasts and cellular proliferation. These proteins are stored in bone ECM in latent form.
Vascular endothelial growth factor (VEGF), angiopoietins—vascular ingrowth, neoangiogenesis, and revascularization of callus.
Wnt/β-catenin—canonical Wnt pathway regulates the amount of β-catenin transcription factor present intracellularly, guides differentiation of MSCs into osteoblasts, and regulates osteoblast activity during bone formation. Wnt signaling also inhibits osteoclastogenesis by increasing osteoblast synthesis of OPG.
Sclerostin—secreted by osteocytes and inhibits Wnt signaling.
OPG/RANKL—OPG is a decoy receptor for RANKL. RANKL produced by osteoblasts stimulates osteoclastogenesis. Their balance leads to resorption of mineralized cartilage and formation of woven bone.
Metabolic/endocrine components
Calcium.
Vitamin D—necessary for bone mineralization.
Vitamin C—necessary for collagen production.
PTH—important homeostatic regulator of serum calcium level and vitamin D metabolism by actions on bone, kidneys, and intestine. It also regulates endochondral bone formation. In recombinant form (Forteo®) it used to increase bone mass. It has also been used in treatment of nonunions, though clinical evidence of efficacy for this indication remains to be proven.
II. Types of Fracture Healing—Putting it All (and the Bone) Back Together
Bone is one of the few tissues that will heal without a scar (i.e., bone will absolutely become bone again). It is in contrast with healing in most of other tissues where there is some component of fibrous tissue (i.e., scar) at the repair/regeneration site. Bone is composed of cells and ECM. Woven bone is immature bone with randomly organized collagen fibers. Lamellar bone is composed of parallel layers of collagen fibers. The physiology of fracture healing remains incompletely understood. However, there are two well-described pathways of fracture healing: primary and secondary.
Primary (direct, intramembranous): This process of fracture healing occurs when a fracture is rigidly fixed (e.g., lag screw and neutralization plate, compression plate; ▶ Fig. 1.2 ).
Fig. 1.2 Primary bone healing. Emanating from a Haversian canal a cutting cone is created. At the lead end of the cone are osteoclasts resorbing bone. They are followed by osteoblast that lay down osteoid. Osteoid eventually mineralizes to become new bone tissue.
MSCs differentiate directly into osteoblasts. Based on Perren’s strain theory, this is a low-strain environment (< 2%).
Cutting cones of osteoclasts followed by osteoblasts laying down osteoid which eventually mineralizes. This recreates Haversian canals/osteons directly across the fracture site.
Secondary (indirect, endochondral): This process of fracture healing occurs in mechanical environments with relative stability (e.g., cast, intramedullary nail [IMN], bridge plate, external fixation; ▶ Fig. 1.3 ).
Fig. 1.3 Secondary bone healing. Following fracture, an acute inflammatory response occurs including the production and release of growth factors and cytokines, and the recruitment of mesenchymal cells to differentiate into chondrocytes. A cartilaginous intermediate is formed that then becomes vascularized. Osteoblasts and osteoclasts get recruited and the cartilage intermediate becomes mineralized and ultimately bone matrix is formed. Finally, this bone is remodeled to fully restore a normal bone structure.
Based on Perren’s strain theory, this is a moderate-strain environment (~2–10%). The amount of strain present decreases over time as the stiffness of the tissues bridging the fracture changes.
Following fracture, an acute inflammatory response occurs including the production and release of growth factors and cytokines and the recruitment of mesenchymal cells to differentiate into chondrocytes. A cartilaginous intermediate is formed that then becomes vascularized. Osteoblasts and osteoclasts get recruited and the cartilage intermediate becomes mineralized and ultimately bone matrix is formed. Finally, this bone is remodeled to fully restore a normal bone structure. Classically, the process is divided into four stages:
Inflammatory/hematoma—inflammatory cells (e.g., macrophages, neutrophils) and platelets debride the wound and release cytokines and cell recruitment factors. Early granulation tissue forms. This stage occurs immediately and up until ~2 weeks after injury.
Soft callus (cartilaginous)—MSCs aggregate and differentiate into chondrocytes. An intermediate cartilage scaffold is formed bridging the fracture site. This stage occurs ~2 to 6 weeks after injury.
Hard callus (endochondral bone formation)—chondrocytes hypertrophy (characterized by collagen X) and intermediate cartilage scaffold is degraded by matrix metalloproteinases and ultimately calcifies. Blood vessels invade and bring cells that differentiate into osteoblasts. Woven bone is laid down. This occurs ~6 weeks till injury site bridged.
Remodeling—woven bone is slowly replaced by lamellar bone. Osteoblasts form new bone while osteoclasts resorb the woven bone. Restoration of bone marrow cavity starts. This occurs after hard callus has formed until bone is fully remodeled according to Wolff’s law. It can take years to complete.
These are idealized stages of secondary fracture healing that in reality occur along a continuum overlapping in time.
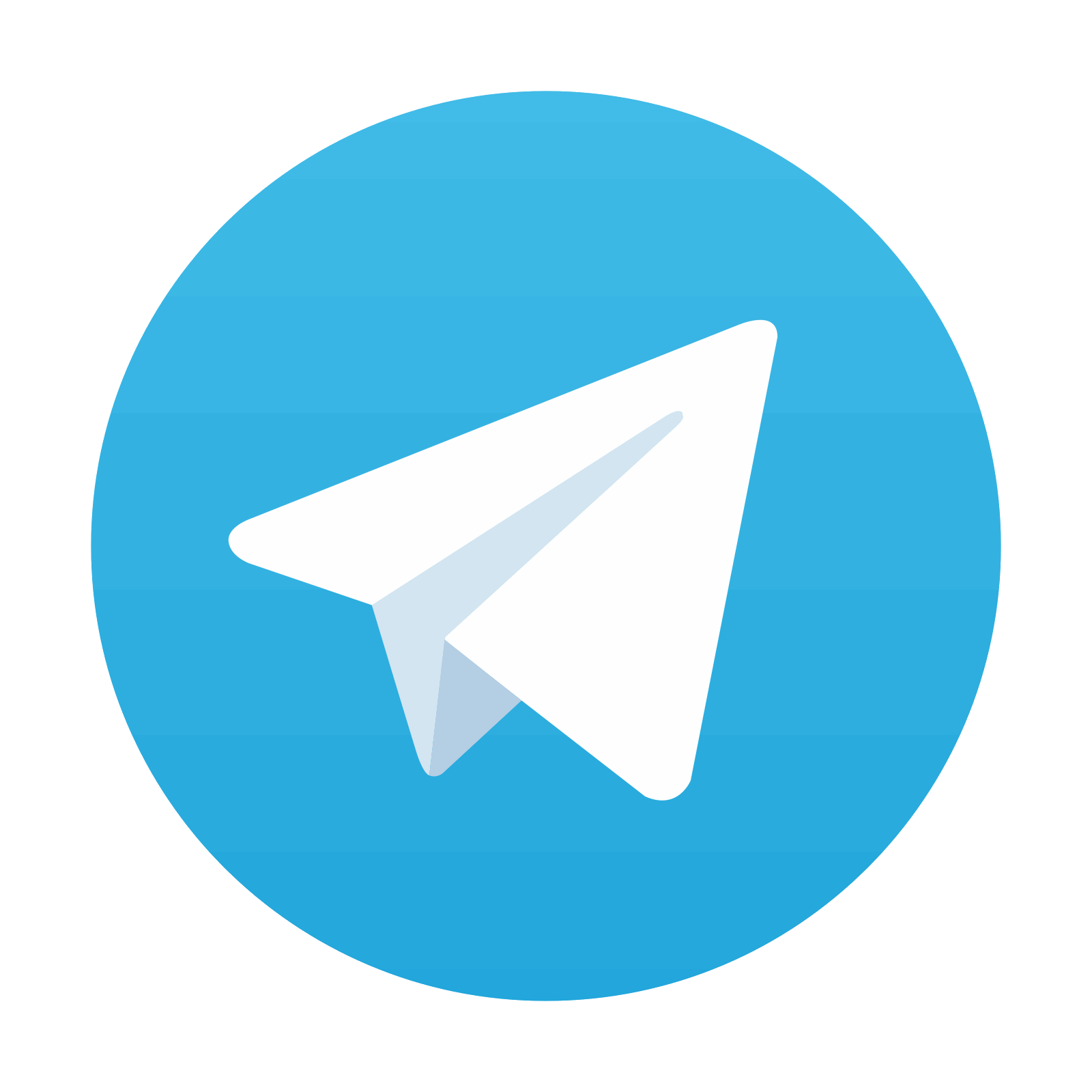
Stay updated, free articles. Join our Telegram channel

Full access? Get Clinical Tree
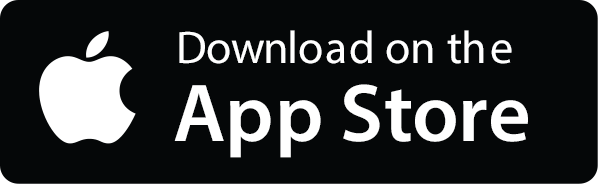
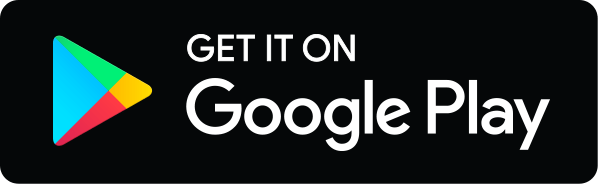