1 Anatomy of the Fracture
Abstract
This chapter discusses the importance of the anatomy of distal radius in relation to fracture. First, we describe the microanatomy of the distal radius. Its microanatomy resembles that seen in everyday engineering structures; the subchondral bone plate and arrangement of trabeculae enabling the wrist to handle high multidirectional loads. Stability of the wrist is achieved by multiple ligamentous rings that confer stability within and between the rows and columns of the wrist. Ligamentous insertions play an important role in fracture morphology, both initiation and propagation of the fracture lines.
Hand position at impact determines the position of the carpus on the distal radius. When a line of differential load occurs in the scapholunate interval, scapholunate ligament injury (SLI) may result. SLI is associated with specific fracture types. “Unmasking” with scapholunate diastasis occurs when there is compromise to the secondary stabilizers.
The volar marginal rim fracture represents an important subset of fractures. These are associated with a higher rate of carpal ligamentous injury and fixation failure. Distal radius plate design has evolved to attempt to capture this fragment.
Lastly, we present a biomechanical model for distal radius fracture.
1.1 Introduction
Distal radius fractures (DRFs) are one of the most commonly treated injuries in orthopaedic practice. A bimodal distribution exists; in younger patients, caused by high-energy and in older patients, caused by low-energy falls. Osteoporotic DRFs reflect a change in the microarchitecture of the distal radius with aging and are a predictor for subsequent fracture of long bones. Classification systems have attempted to provide clarity to the morphology and treatment of these injuries.
With an improved understanding of the anatomy of distal radius, we are better equipped to treat the patient, respecting not just the fracture components but also the contribution of the surrounding ligaments, carpal bones, and other associated injuries. This chapter will explore the importance of each component and its interaction in the mechanism of fracture. First, we will study the microanatomy of the distal radius; and then we will focus on fracture initiation and the role of the carpus; and third, the role of the carpal ligaments and fracture propagation. We will look at the specific anatomy of the volar rim fracture. We will propose a biomechanical model for DRF.
1.2 Microanatomy of the Distal Radius: A Feat of Evolutionary Engineering
Singh reported the trabecular structure of the proximal femur and changed our approach to the understanding and management of these injuries. 1 The microstructure of the distal radius assists in understanding its behavior under load. We performed an analysis of the architecture of cadaveric distal radii on micro-computed tomography (CT) 2 , 3 and found it resembled many of the engineering concepts in everyday man-made structures. Interestingly, an engineer once advised us that he knew when he had the design correct and when it resembled the structures identified in nature.
1.2.1 The Subchondral Bone Plate: The “Leaf Spring” of the Wrist
The subchondral bone plate is a 2-mm-thick multilaminar plate that absorbs impact and transmits the load to the radial metaphysis. The superficial “primary” bone plate bridges the entire articular margin. Multiple deeper layers resemble a leaf spring used in the suspension of heavy motor vehicles.
Between these laminae, and connecting them, lie intralaminar struts. These are initially perpendicular to the joint line, but more proximally align with the radial shaft. Voids between the struts and laminae absorb impact and enable vessels to perforate. The struts between the laminae are mini “I-beams,” which make the structure strong but still enable it to bend. Engineers refer to this multilayered lamina construct as a “honeycomb sandwich panel.”
With physiological wrist extension, the volar capsule becomes taut, and the scaphoid and lunate load the distal radius subchondral bone plate.
The multilayered subchondral bone plate resists buckling and transmits the load to the intermediate trabeculae and then the metaphyseal arches (▶Fig. 1.1).

On a magnified view, areas can be seen where these trabeculae coalesce to form a rod and extend as longitudinal ridges down the diaphysis (▶Fig. 1.2). These align in the direction of load through the cortical bone and reinforce the radius, similar to the longerons in a plane’s fuselage (▶Fig. 1.3), and prevent torsional failure and buckling.


The load bearing area of the lunate is very volar. The sagittal images demonstrate the thick volar trabecular columns spanning to the volar cortex of the metaphyseal radius (▶Fig. 1.4). This explains the devastating effect of Barton volar lip fracture, as the carpus will simply dislocate volarly.

Further dorsal on the lunate facet, trabeculae course dorsally forming a curve in the shape of a gothic arch, with an underlying intramedullary “vault.” From medial to lateral through the distal radius, these gothic arches are in series—connected by interarch struts. In normal bone under normal loads, the parabolic shape of the arch transfers loads in a longitudinal and lateral direction, to the base of the arch without creating tension (▶Fig. 1.4).
At the base of each arch, the trabeculae merge with the cortex, which then becomes thickened—therefore buttressing the arch. In contrast, at the articular margin, the cortex is thin. It acts to suspend the subchondral bone plate and serves as a site for ligament attachment, rather than to bear the load.
1.2.2 The Arch Bridge Concept
The microstructure of the distal radius resembles an arch bridge with the following equivalent: deck—SBP, intermediate struts—intermediate trabeculae, arches—arches, and bridge foundation—cortex. The deck is a tightly held lattice with multiple “I-beams” in a multilayer sandwich panel construct. This resists buckling, absorbs impact, and takes the entire load (▶Fig. 1.5).

The arches and intermediate struts are a semiflexible construct, which distributes compressive forces from the deck to the base (diaphysis). 4 The orientation of the microstructure ensures that forces are distributed in compression rather than tension (where the bone is weakest).
1.2.3 Multiple Rings Concept of the Wrist
Force transfer from the hand to the radius at the time of DRF involves the transmission of force through the three columns of the wrist. The three columns are bound by a series of ligamentous rings. These ligamentous rings provide stability within and between the proximal and distal rows of the wrist (▶Fig. 1.6).

Within Rows
The distal radioulnar joint is bound by a fibroligamentous ring. Disruption of this at the time of DRF can lead to dislocation of this joint and distal radioulnar instability, if not addressed at the time of surgery.
Within the proximal carpal row, interosseous ligaments on the volar and dorsal aspects of the scapholunate and lunotriquetral joints form a ring. Transmission of force from the central column to the scaphoid, lunate, or both can lead to a disruption of these ligaments and a “greater arc” injury or an impaction fracture of the distal radius.
The distal carpal row is tightly bound by interosseous ligaments, allowing for minimal motion within the row. Disruption of this ring rarely occurs at the time of DRF but is seen with high-energy axial fracture-dislocations of the carpus.
Between Rows
A series of ligaments connect the rows of the wrist. Kuhlmann ring refers to the volar radiotriquetral ligament and the dorsal radiocarpal ligament (DRC) complexes. 5 Disruption of this ring in complex high-energy DRFs can lead to ulnar translocation of the carpus. The DRC also acts as a secondary stabilizer to the scapholunate joint.
The scaphotrapeziotrapezoid (STT) ligament stabilizes the scaphoid to the distal carpal row. As a secondary stabilizer of the scapholunate joint, disruption of this complex can lead to scapholunate diastasis and dorsal intercalated segmental instability (DISI) deformity.
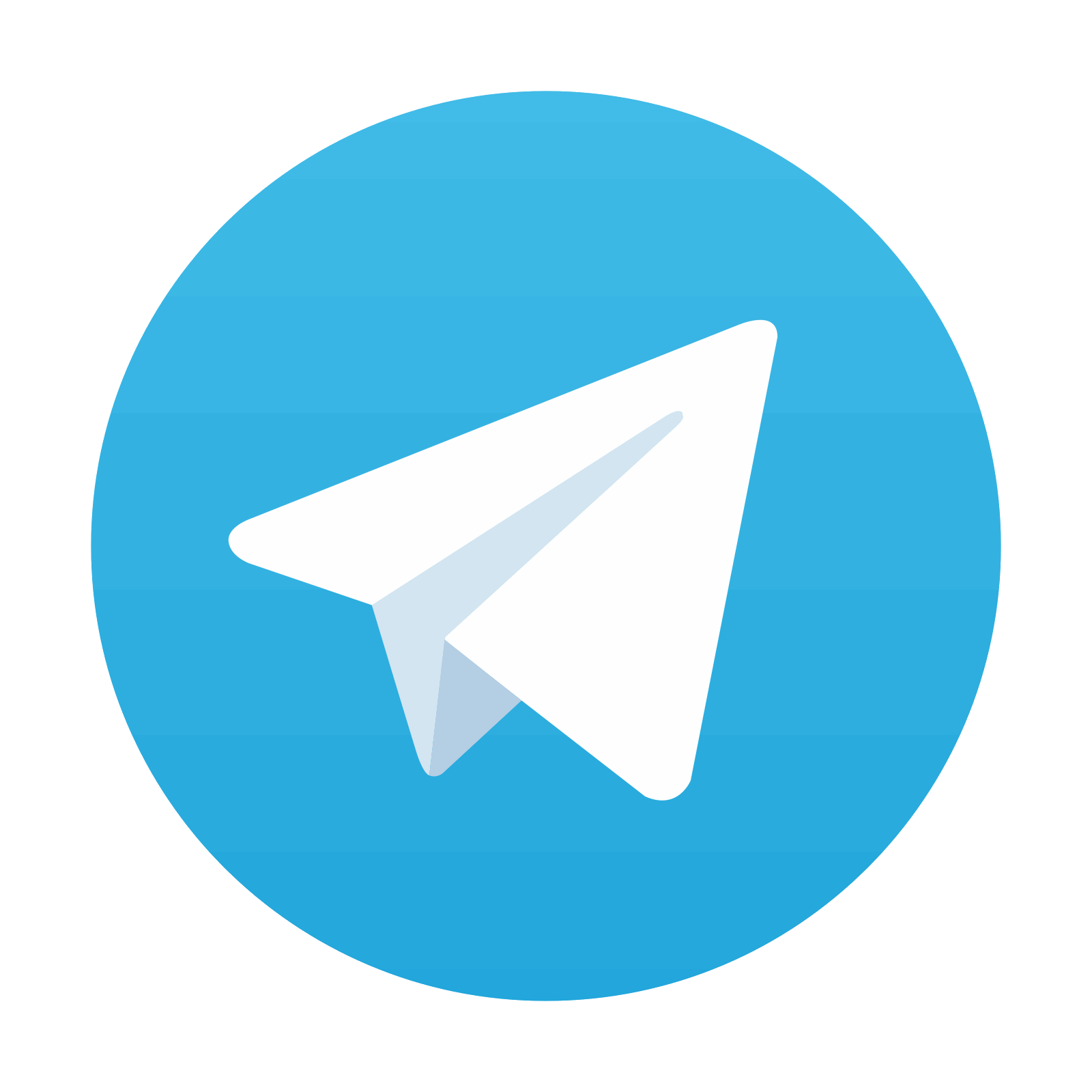
Stay updated, free articles. Join our Telegram channel

Full access? Get Clinical Tree
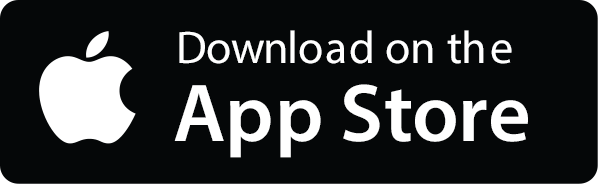
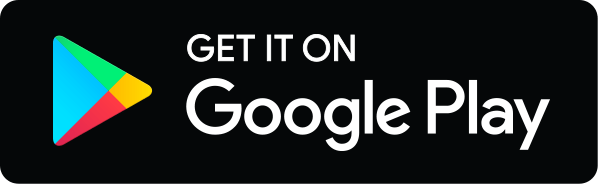
