1.5 Soft-tissue injury: pathophysiology, evaluation, and classification
To access the videos, please follow the URL link
1 Introduction
The biology of bone healing is dependent on a number of factors, the most important of which is the status of the soft-tissue envelope. Girdlestone likened the bone to a plant with its roots in soft tissue, and this comparison is a powerful image for the trauma surgeon to remember.
The effective treatment of fractures depends on good soft-tissue management.
The energy transfer required to fracture bone also results in damage to the neighboring soft tissue, with a “zone of injury” surrounding any fractured bone. This chapter describes the evaluation, classification, and pathophysiological response to soft-tissue injury, and lays the foundation for the management of the trauma patient.
Open fractures and fractures with severe, closed soft-tissue damage are often associated with polytrauma. Life-saving treatment must always take priority and the surgeon must consider both the local injury and the whole patient. Evaluation of the fracture should also determine the extent of the soft-tissue injury, which will be a key factor in management. The surgeon needs to be familiar with the pathophysiology of the soft-tissue injury and the timing, risks, and benefits of the different treatment options.
Knowledge of advanced wound care and an understanding of the biology of wound healing is as important as knowledge of the various fracture treatment options, which will assist the trauma surgeon in making appropriate management decisions.
2 Pathophysiology and biomechanics
The condition of the wound after injury is determined by several factors, including:
Type of insult and area of contact (blunt, penetrating, crushed, ballistic, etc)
Magnitude of the force applied
Direction of force
Area(s) of body affected
Wound contamination
General physical condition of the patient
A combination of these factors will produce different types of wounds ( Table 1.5-1 ). Wounds do not only differ in their physical presentation but also in the type of treatment required and the prognosis for healing [1]. All injuries cause bleeding and tissue destruction. This activates humoral and cellular mechanisms to stop bleeding and resist infection. The sequential healing process starts immediately after trauma and can be divided into three phases:
Type of force | Type of injury |
Sharp, pointed | Stab wound, cut |
Blunt | Contusion injury, blow |
Extension, twist | Laceration |
Shear | Degloving, wound defect, avulsions, abrasion |
Combination of forces | Wounds from blows, impaling, bites, and gunshot |
Crushing | Traumatic amputation, rupture, crush injury |
Thermal | Burns |
Exudative or inflammatory phase
Proliferative phase
Reparative phase
3 Pathophysiological responses in healing
Bioactive proteins, such as growth factors, are crucial for tissue healing and are released with platelet activation, eg, platelet-derived growth factor (PDGF), transforming growth factor (TGF), vascular endothelial growth factor (VEGF), insulin-like growth factor (IGF), epidermal growth factor (EGF). The extracellular matrix (ECM) also affects cellular growth and differentiation, activates the repair system and growth factor release, and is in turn regulated by growth factors, such as TGF-β [2]. These bioactive proteins are histopromotive factors, which set the stage for tissue healing including cellular chemotaxis, proliferation, differentiation, removal of debris, angiogenesis, and the development of ECM. Research into the effect of plasma-based biomaterials on soft tissue and bone healing has shown that soft-tissue repair seems to be positively influenced, and infection rates are lower but conclusive evidence of improved bone healing remains elusive [3].
3.1 Inflammatory phase
In the inflammatory phase, there is an increased interaction between leukocytes and the injured microvascular endothelium. Trauma exposes subendothelial collagen structures, leading to the aggregation of platelets. These release serotonin, adrenaline, and thromboxane-A, causing vasoconstriction and producing cytokines, such as PDGF and TGF-β that have a strong chemotactic and mitogenic effect on macrophages, polymorphonuclear neutrophils, lymphocytes, and fibroblasts. Vasoconstriction and thrombocyte aggregation contribute to clotting and are an important part of the coagulation process to stop bleeding. As an adverse effect the damaged tissue is underperfused, leading to hypoxia and acidosis. The first cells to move from the small vessels into the damaged tissue are polymorphonuclear neutrophils (PMN) and macrophages. Polymorphonuclear neutrophils are mobilized rapidly and produce an extremely vigorous initial response. The main function of macrophages is the removal of necrotic tissue and microorganisms (phagocytosis and secretion of proteases) and the production and secretion of cytokines (PDGF: mitogenic and chemotactic; TNF-α: proinflammatory and angiogenic; β-FGF, EGF, PDGF, and TGF-β: mitogenic [4]).
Macrophages are responsible for the cytokine-induced early activation of immunocompetent cells, the inhibition and destruction of bacteria, and the removal of cell debris from the damaged tissue. However, the capacity of the macrophages for phagocytosis is limited. If their capacity is overloaded by an excessive amount of necrotic tissue, this decreases the antimicrobial activities of the mononuclear phagocytes. Since these phagocytic activities are associated with superoxide production and high oxygen consumption, areas of hypoxia and avascular areas are especially threatened by infection and bacterial biofilm formation. Thus, the pathophysiological rationale for performing radical surgical debridement of dead tissue is to support the phagocytic process of the macrophages.
Chemotactic substances, such as kallikrein, improve vascular permeability and exudation by releasing the nanopeptide, bradykinin, which belongs to the α2-globulin fraction. Originating from tissue debris, prostaglandins stimulate the release of histamine from mast cells and cause local hyperemia that is necessary for the metabolic processes of wound healing. In addition, highly reactive oxygen and hydroxyl radicals are released during the peroxidation of membrane lipids, which cause a further destabilization of the cell membranes. These mechanisms result in an impairment of capillary endothelial permeability that again promotes hypoxia and acidosis in the damaged areas. The infiltrating granulocytes and macrophages with their capacity to resist infection and to engulf cell debris and bacteria (physiological wound debridement) play a key role in the inflammatory response of traumatized tissue and therefore have a decisive effect on the subsequent reparative processes.
3.2 Proliferative and reparative phases
The proliferative phase begins when fibroblasts, followed by endothelial cells, migrate into the area of the wound and proliferate. This is stimulated by mitogenic growth factors. These cells have a series of growth factor receptors on their surfaces and, by paracrine and autocrine processes, release several cytokines and synthesize structural proteins of the extracellular matrix, such as collagen. Fibronectins, proteins detached from the surface of the fibroblasts by hydrolases, facilitate the bonding of type I collagen to α1-chains. This is an important prerequisite for progressive, reparative cell proliferation.
There is a smooth transition to the reparative phase and, simultaneously, the proliferating endothelial cells form ingrowing capillaries, which is the typical characteristic of granulation tissue. At the end of the reparative phase, water content is reduced and the collagen initially formed is replaced by cross-linked collagen type III. Fibrosis and scarring follow. The role of the growth factors in scar formation remains unclear but it seems that TGF-β plays a decisive role [5].
4 Diagnosis and treatment of closed soft-tissue injuries
4.1 Problems of diagnosis and assessment
The absence of a visible wound can cause clinicians to underestimate the severity of a closed injury. The degree of injury and ischemic tissue may not be apparent and this can make diagnosis and therapeutic decisions difficult [6]. Many modern imaging techniques permit qualitative assessment of closed soft-tissue injuries but clinically useful quantitative assessment of damage is lacking. There are no diagnostic criteria that allow definitive, preoperative differentiation between reversibly (living) and irreversibly (dead or dying) damaged tissue. Thus, clinical experience and good judgment remain essential when selecting options for treatment and prognosis. Drugs that can reduce posttraumatic microvascular dysfunction and restore the disturbed microcirculation are being investigated [7].
4.2 Secondary damage
The immune response to trauma results in a drastic increase in leukocyte-endothelial interaction and subsequent loss of endothelial integrity with increased microvascular permeability ( Fig 1.5-1 ). This leads to transendothelial leakage of plasma and interstitial edema [8]. Edema may reduce the microvascular blood supply in adjacent areas and this can result in progressive necrosis of skeletal muscle or skin in marginal areas that were not directly affected by trauma. Thus, secondary tissue loss may occur.

4.3 Systemic response to soft-tissue injury
Severe soft-tissue injury results in local microvascular and cellular damage and can also lead to a marked systemic inflammatory response due to the release of proinflammatory cytokines (TNF-α, IL-1, IL-6, IL-10). These affect vascular endothelium in various organs, resulting in margination, migration and activation of polymorphonuclear neutrophils, increased capillary permeability, interstitial edema, and an inflammatory response. The systemic inflammatory response syndrome (SIRS) may result in damage to various organs, eg, multiple organ dysfunction syndrome (MODS). The damage is not only to organs such as the lungs (ARDS), liver, gastrointestinal tract, kidneys, myocardium, and central nervous system but also affects the entire immune system: sepsis remains the most common cause of death in these patients. Thus, the pathophysiological changes in damaged tissue after soft-tissue trauma are the product of a vicious cycle.
4.4 Evaluation of soft-tissue injury
4.4.1 Case history
To determine the appropriate choice and timing of treatment, the surgeon needs to know when, where, and how the injury occurred. For instance, prolonged entrapment in a car may indicate the possibility of compartment syndrome, and barnyard injuries have a high risk of infection. Gunshot injuries are influenced by the velocity of the bullet and the associated cavitation, which will result in a significant, occult zone of injury that must not be overlooked.
Most important is the knowledge of the amount and direction of force or energy causing the injury. This determines both the extent of the injury and the necessary steps in treatment. The greater the force, the more serious the damage and sequelae will be.
4.4.2 Systematic examination
Assessment of the skin and muscle
Soft-tissue injuries in closed fractures are less obvious than in an open fracture, but still have enormous importance. Their evaluation can be much more difficult than open injuries and their severity is easily underestimated. Simple abrasions represent an injury of the physiological skin barrier and can allow the development of deep infection.
“Closed skin degloving” occurs when there is shear force, resulting in the skin separating from the underlying fascia without any external break in the skin. The resultant cavity can be filled with serous fluid or hematoma, and the vascularity of the dermis is compromised. The dermis is also denervated and an insensate area of skin, which feels “slippery” to the touch, is pathognomic of a closed degloving injury. Treatment consists of emergent drainage of the collection and pressure dressing. In some instances, an indwelling drain should be left in situ and withdrawn progressively. The aim of this treatment is to allow contact between the separated layers, and acts as an in situ skin graft. The affected skin must be observed diligently to ensure no necrosis occurs. If a degloving injury occurs with concomitant breach in the skin, it is termed an “open degloving injury”. The management is much the same, however if there is an associated fracture this injury should be regarded as an open fracture.
Research has shown that the intradermal collection is at risk for contamination and may act as a source of deep infection (The Morel-Lavallée lesion in pelvic and acetabular fractures is an example) [9, 10].
“Fracture blisters” are formed when there is significant acute swelling of a limb, with resultant shearing at the epidermal level. Fracture blisters are sterile and best treated by antiedema measures alone. However, blood-filled fracture blisters are “more serious” blisters, as they are indicative of significant deeper tissue damage and potential healing delay ( Fig 1.5-2 ).

In open fractures (see chapter 4.2), the wound should be covered by a moist sterile dressing after it has been thoroughly irrigated in the emergency department and this should not be removed until the patient is in the operating room. Only there, and under sterile conditions, can the full extent of the soft-tissue damage be assessed. Some authors suggest that a photograph is important to facilitate surgical planning for the team. There is a significant increase in reported infection rate with repeated exposure of the wound. Further evaluation in the operating room under sterile conditions and optimum vision is useful and may affect the final classification and prognosis. The degree of wound contamination is important and influences the course and outcome of the injury. Foreign bodies and dirt particles give useful information about the level of contamination and help the surgeon with grading these injuries. High-velocity gunshot wounds, blasts, and farming injuries are considered as severely contaminated.
Surgical debridement becomes a diagnostic exercise as skin edges, subcutaneous fat, muscles, and fascial elements are checked for viability and bleeding. The definitive assessment of a soft-tissue injury requires an experienced surgeon because it determines the treatment protocol as well as the surgical approach and choice of implant for fracture fixation [11].
Assessment of the vascular status
It is mandatory to determine the vascular status of all injured limbs. The peripheral pulses, temperature, and capillary refill must be checked and compared with the uninjured side. The vascular status must be recorded in the medical history. Although the absence of a palpable pulse is an important pointer to potential vascular damage, the presence of a pulse or good capillary refill does not necessarily guarantee an intact vascular supply. Doppler examination of the injured and unharmed extremity may be helpful for screening and the ankle-brachial index (ABI) is also useful [12]. In all cases of doubt or where the case history, the physical examination, or the radiographic fracture pattern suggests vascular damage, the opinion of a vascular surgeon must be obtained urgently. Management strategies include urgent angiography in the vascular radiology suite, immediate on-table angiography or direct exposure of the injured vessel. The method chosen depends on local facilities, timing, and protocols.
Assessment of the neurological status
Neurological assessment can be difficult in unconscious patients with multiple injuries. However, examination of the reflexes and the response to strong, painful stimuli give some indication of major deficits. These examinations must be performed repeatedly because the confirmation of a major nerve deficit can be decisive in the choice between salvage versus amputation in severely injured extremities.
Assessment of the fracture
The radiographic fracture pattern provides essential indirect information about the soft-tissue injury and can show foreign bodies, dirt, soft-tissue density and entrapped air around and/or distal to the fracture site. The force absorbed by the bone is indicated by the fracture pattern, and indicates the equivalent force likely to have been applied to the soft tissue.
At the time of debridement, careful inspection of bone fragments, their relationship to the soft-tissue envelope and blood supply, as well as the information obtained from x-rays, help to optimize assessment of the damage.
4.5 Compartment syndrome
Compartment syndrome is due to raised pressure in a closed fascial or osteofascial space that results in local tissue ischemia. This will compromise neuromuscular function and may result in muscle necrosis with loss of function, infection, and possible amputation [13, 14].
Compartment syndrome is seen most frequently in the lower leg, but can also occur in the forearm, buttock, thigh, hand, and foot. It may occur at any time during the first few days after trauma or surgery.
4.5.1 Pathophysiology
In closed fractures with soft-tissue injury, the threat posed by compartment syndrome is not to be underestimated. It is triggered by an increase in intramuscular volume and pressure within a closed osteofascial space, at a level above a critical microvascular perfusion pressure [15]. The cause may be exogenous pressure (eg, restrictive plaster casts) or endogenous pressure due to an increase in volume within the compartment. The latter results from hemorrhage, perivascular infusions, or edema that is caused by abnormal capillary permeability, which in turn is due to prolonged ischemia or reperfusion ( Fig 1.5-3 ). If impairment of the microcirculation from increased tissue pressure persists, severe and irreversible neuromuscular dysfunction due to hypoxia will result and lead to muscle necrosis and axonotmesis.

Originally, it was believed that the threshold for compartment syndrome was a constant intramuscular pressure more than 30 mm Hg. However, it is now recognized that the key factor is the difference between the diastolic blood pressure (dBP) and the intramuscular pressure (IMP). This determines the mean muscle perfusion pressure (MPP/DeltaP), for example: 78 (dBP) – 51 (IMP) = 27 (MPP/DeltaP).
dBP – IMP = MPP/DeltaP
If the muscle perfusion pressure is less than 30 mm Hg, there will be hypoxia and anaerobic cell metabolism. It is important to note that blood pressure has a direct relationship to perfusion pressure. Thus, multiply injured patients with hypotension and hypoxia are predisposed to compartment syndrome. Injuries with a high risk of developing compartment syndrome include vascular injuries with peripheral ischemia and reperfusion, high-energy trauma, severe soft-tissue crush, and complex fractures of the tibia [11]. The aim of any therapeutic procedure must be immediate compartment decompression by dermatofasciotomy to achieve reperfusion of the capillary bed.
Acute compartment syndrome is commonly progressive in nature, and requires urgent attention to avoid irreversible muscle and tissue damage. The law of Laplace relating to membrane permeability and equilibrium applies.
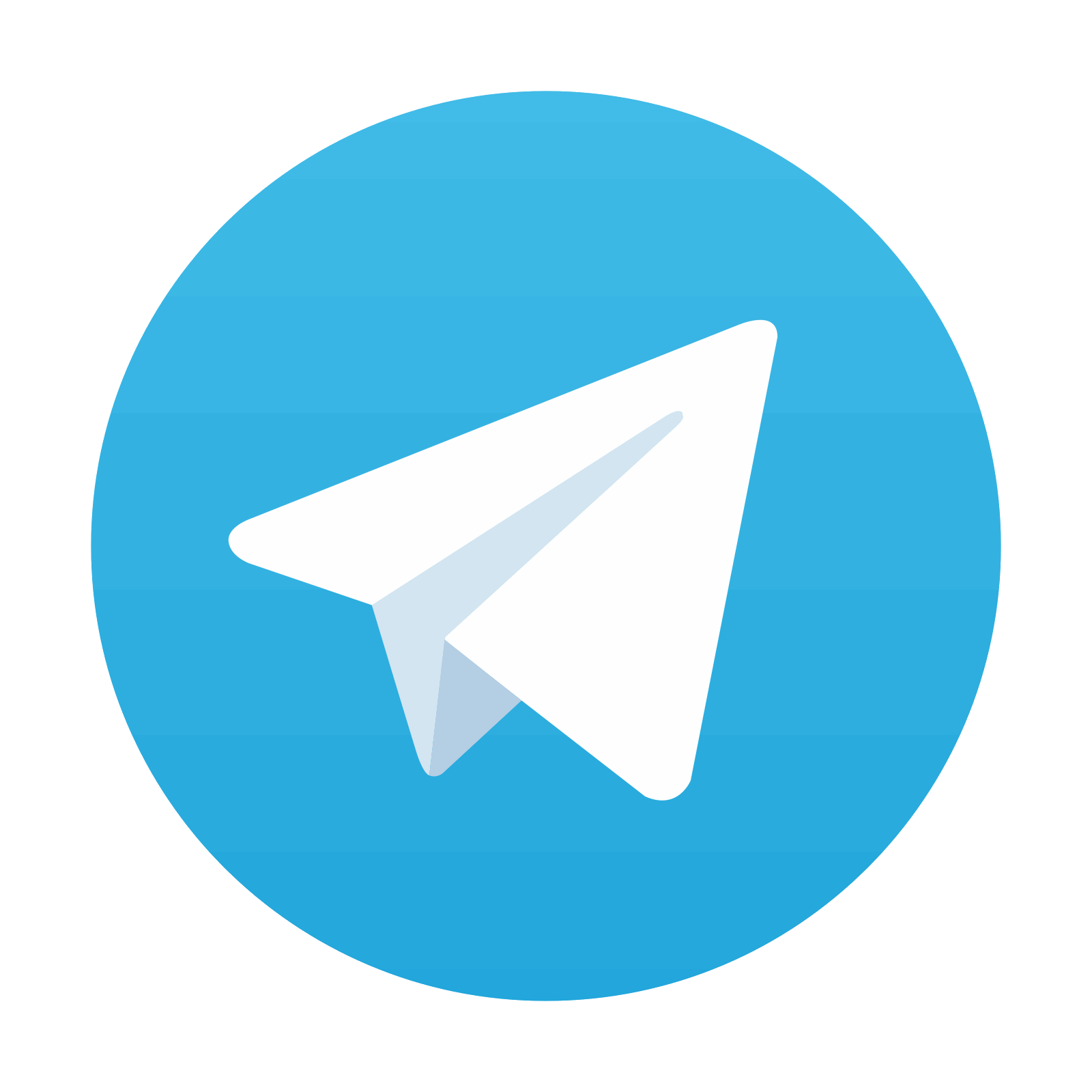
Stay updated, free articles. Join our Telegram channel

Full access? Get Clinical Tree
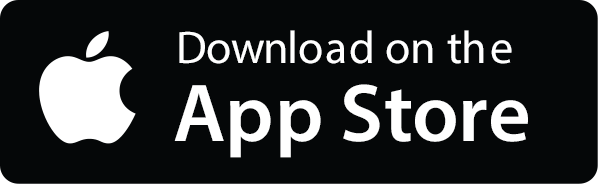
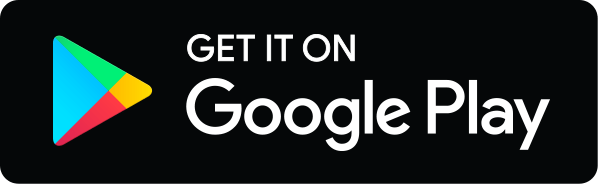
