1.5 Implants
Material Nicola Kildea, Jessica Hayes
Care and storage of implants Judith Roberson
Principles of care and handling of implants
Checking and ensuring validity of sterile implants
Intraoperative handling and tracking
Damaged and broken implants Nicola Kildea, Anna Wilkins
Authors Nicola Kildea, Jessica Hayes, Judith Roberson, Anna Wilkins
1.5 Implants
The aim of implants in the treatment of fracture fixation is to restore the structural and functional integrity of damaged bone, while encouraging it to heal. Attaining this goal is dependent on a complex interplay of material properties, device design, physiological requirements, implant handling, and the patient’s health condition.
1.5.1 Material Nicola Kildea, Jessica Hayes
Materials used for fracture fixation implants include but are not limited to metals, degradable and nondegradable polymers, ceramics, bioresorbable calcium phosphate cements, and nonresorbable cements. New implant materials (generally within these classes) are continuously being researched and developed.
Implant materials used in internal fracture fixation must meet certain mechanical and biological requirements. Properties such as biological safety (biocompatibility), corrosion resistance, ductility, strength, stiffness, and fatigue behavior must be considered when choosing an implant material. Metal offers a good degree of these properties and is the most commonly used material in manufacturing implants for internal fixation. The metals used today in fracture fixation are either stainless steel, commercially pure titanium (cpTi), or titanium alloys, such as titanium-6% aluminium-7% niobium (TAN), and titanium-15%molybdenum (Ti15Mo).
Choice of implant material
The choice of material depends primarily on the requisite function to be accomplished and also on the manner in which the implant will be applied. The anatomical location and the required function (ie, high- vs low-load bearing, single- or multicomponent system) of the device will also determine the type of material to be used. Materials used for internal fixation must fulfil various fundamental mechanical requirements, the main considerations being stiffness and strength of the material, its ductility, and importantly, its biopassivity. These are discussed in the subsequent chapters. Other factors, such as economics, implant availability, surgeon’s preference, and patient needs and preferences may also be important in the final material used.
Stainless steel
Today stainless steel is one of the most frequently used biomaterials for implants in internal fixation. Stainless steel is a good implant material as it has excellent mechanical properties; it is corrosion resistant and cost-effective compared with other suitable metals (although this is no longer true for complex highly engineered implants, such as LCP and expert tibia nails when the cost of the material is small compared with costs of manufacturing and engineering each device). It also has an excellent record since it has been used successfully for many years in humans as an implant material.
There are studies, however, which show allergic reaction to nickel. Stainless steel is estimated to contain 1–2% nickel; although the clinical relevance of these is unclear. In contrast, cpTi and its alloys have the advantage of not containing nickel within the bulk material; therefore, nickel sensitivity is not an issue. However, recently new nickel-free stainless steels are being developed to address this issue, although these would be more expensive than conventional stainless steel.
Commercially pure titanium (cpTi)
Titanium has also been used as an implant material safely for many years. Because of cpTi’s superior strength, corrosion resistance, acceptance by bone and soft tissue (good biocompatibility), superiority over stainless steel under cyclic loading and excellent biopassivity, cpTi has in recent years emerged in Europe (although not in North America, apart from with nails) as the forerunner in internal fixation devices. The density of unalloyed titanium is lower than stainless steel. This decrease in density equates to a weight reduction of approximately 50% when materials of similar volumes are compared. This can make larger titanium implants more comfortable when implanted into the patient. Titanium also produces less image artefacts in magnetic resonance imaging (MRI) than steel.
Titanium alloys
One issue that surrounds the use of unalloyed titanium is its apparent weakness when used in high-load bearing regions. This concern has been overcome by the introduction of titanium alloys. In the last 25 years or so, TAN has come to the forefront. The microstructure of TAN provides improved implant strength compared with cpTi but it has decreased tensile strength and ductility. Consequently, titanium alloys, such as TAN, are not suitable for cerclage wire as the poor ductility means it does not have the malleability required for wire. Titanium alloys tend not to be used for areas that need a great deal of device contouring and manipulation due to the microcracking that can occur. In contrast, the high strength and low modulus of elasticity of titanium alloys are ideal for implants demanding high resistance to stress loading. The alloys have similar biocompatibility properties to titanium and produce far fewer MRI artefacts than steel.
Corrosion resistance
“Corrosion is an electrochemical process that results in the destruction of metal by the liberation of ionic metal.“
Corrosion differs for single-component and multicomponent systems (ie, screw and plate). If tested as a single-element stainless steel—cpTi, TAN, and Ti-15Mo are all highly corrosion resistant, even in the presence of biological fluids. However, multicomponent systems are more challenging (Fig 1.5-1).
The ability of all these metals to resist corrosion is attributable to the oxide layer which forms on their surfaces (readily for titanium and its alloys). This passivation layer offers protection from the toxic elements found within the bulk of the material by preventing excessive diffusion of oxygen into the base material. The composition and the thickness of this oxide layer differ with any given material. Because of these differences, the oxide passivation film that forms on titanium and its alloys is more resistant to corrosion and thermodynamically more stable than the chromium oxide film that forms on stainless steel.
For internal fixation devices, the major form of erosion is fretting that occurs when micromotion between two adjacent implant components is encountered (such as when a screw head moves in relation to the plate hole). This micromotion can result in the production of small metal “fretting” particles into adjacent tissue, and if severe enough can cause failure of the fixation. With cpTi and its alloys, metal debris produced from micromotion generally results in relatively large metal particles (sizes in micrometer range) and often produces discoloration of the local surrounding tissues. In contrast, because of smaller particles produced by steel fretting (nanoparticulates), dissemination is often encountered and metal particles derived from steel can be found in the liver, kidney, and in the lymphatic system.

Ductility
“The ductility of a material is the degree of permanent (plastic) deformation it can tolerate before it breaks,” or in other words the degree to which an implant, such as a plate, can safely be contoured. Materials of high strength, such as titanium alloys and cpTi, offer less ductility than steel. This means that generally a titanium plate cannot be bent or contoured as easily as a stainless steel plate. However, cpTi and its alloys have an elastic modulus closer to that of bone than stainless steel; thus, local stress concentrations on bone are reduced with use of these materials. Titanium plates should only be bent once to shape because over or multiple bending may cause a loss of strength in that area. It is recommended that surgeons do not bend preformed titanium plates.
Strength
Strength is the ability of a material to resist the application of forces without deformation. Thus, strength determines the level of load an implant can resist. “For internal fixation, the resistance of an implant to repeated load, which may result in failure by fatigue, is a critical issue.” The strength of cpTi is approximately 10% less than steel; however, an increase of implant cross-section/thickness compensates for the difference in material strength. While steel is more resistant to single loads than cpTi, the latter is superior under more natural high-cycle repeated load.
1.5.1.1 Biocompatibility
This describes the suitability of a material for exposure to the body tissues or fluids within the site of application; ie, the way the body reacts to the implanted material. The body provides a hostile electrolytic environment that can lead to the corrosion of implants. Nonbiocompatible materials may cause the foreign body reaction with fibrous encapsulation and/or inflammation.
The manner in which material affects the body must be demonstrated through testing and analysis before they are approved by regulatory boards for implantation in the body. The major materials, outlined here that are used for fracture management implants today, are considered biocompatible and are approved for human use. However in reality no material is truly biocompatible. In fact, most material used to make orthopaedic implants have some toxic components but the naturally occurring oxide passivation layer on their surface helps shield these toxic elements from the body. They can all potentially corrode and cause complications inside the body. In general, cpTi and titanium alloys are considered to have better biocompatibility than stainless steel which is again attributable to the oxide layer of the material, and there is some evidence that titanium implants may be more resistant to infection than stainless steel ones.
Implant-tissue interface
The implant-tissue interface is the contact between implants to soft tissue and bone. There are different tissue reactions depending on what metal implant is used. To date, stainless steel implants are fabricated for clinics with a smooth, mirrorlike surface; while in contrast, cpTi and its alloys are produced with a standard microrough surface. This difference in the material surfaces produces diverse biological responses due to the resulting differences in their implant-tissue interfaces. Due to the smooth surface of stainless steel, micromotion within the implant-tissue interface may occur. This micromotion can lead to the formation of a thick and dense fibrous capsule with a liquid-filled void. The dead space provided by the capsule prevents access of any of the body’s cellular defence mechanisms; providing a safe haven within the void for possible bacterial growth and infection. In some cases, however, the prevention of soft-tissue adhesion to an implant can be beneficial, such as in hand surgery when tendon gliding is required for free movement to occur over the implants. The movement of tendons is large (several millimeters) and this prevents the formation of a liquid-filled void. In pediatrics implant removal is more common and it is usually easier to remove current polished stainless steel implants than titanium implants (which are not currently polished) (Fig 1.5-2).


In contrast, the surfaces of cpTi and its alloys (as currently used in clinical practice) promote direct tissue adherence to the implant, and generally do not form fluid-filled voids around the implant. This decreases the chance of infection, and increases the integration of the implant into the surrounding hard and soft tissues, which can make these implants difficult to remove (Fig 1.5-3).
The development of polished cpTi and cpTi alloy implants offer mechanical and biological benefits of these materials, but assessment of their ease of removal is ongoing. Selection criteria for implant material must be made on an assessment of each case, as both have advantages and disadvantages (Fig 1.5-4).

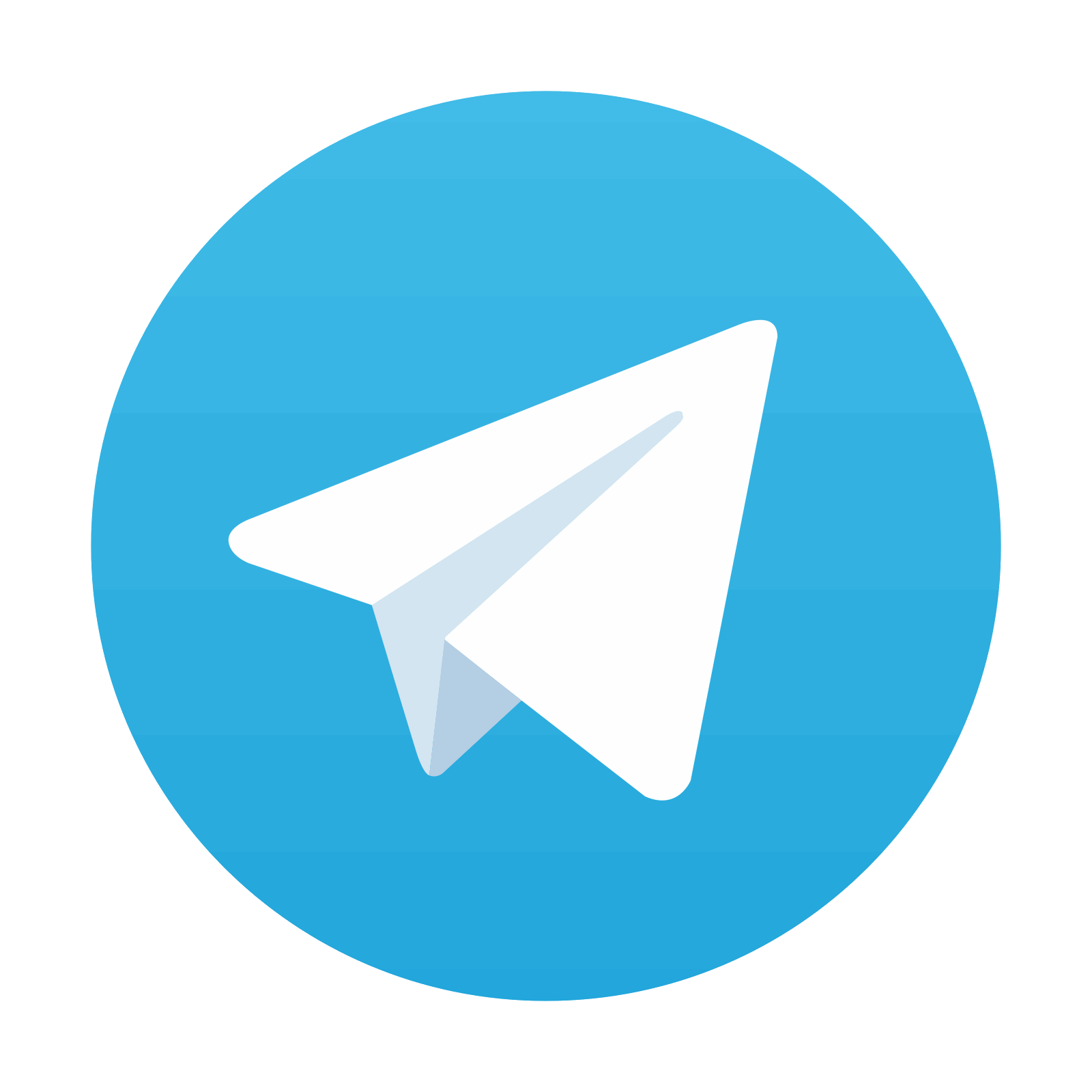
Stay updated, free articles. Join our Telegram channel

Full access? Get Clinical Tree
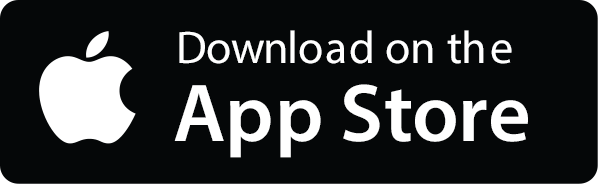
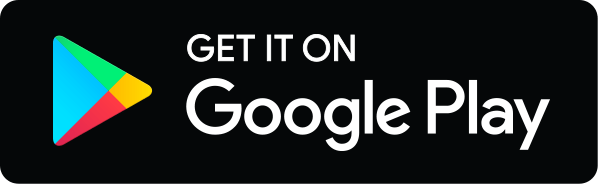
